Agarose Gel Recipes: A Comprehensive Guide
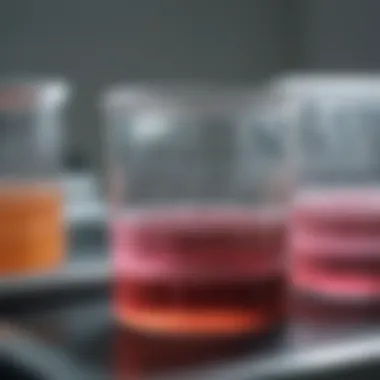
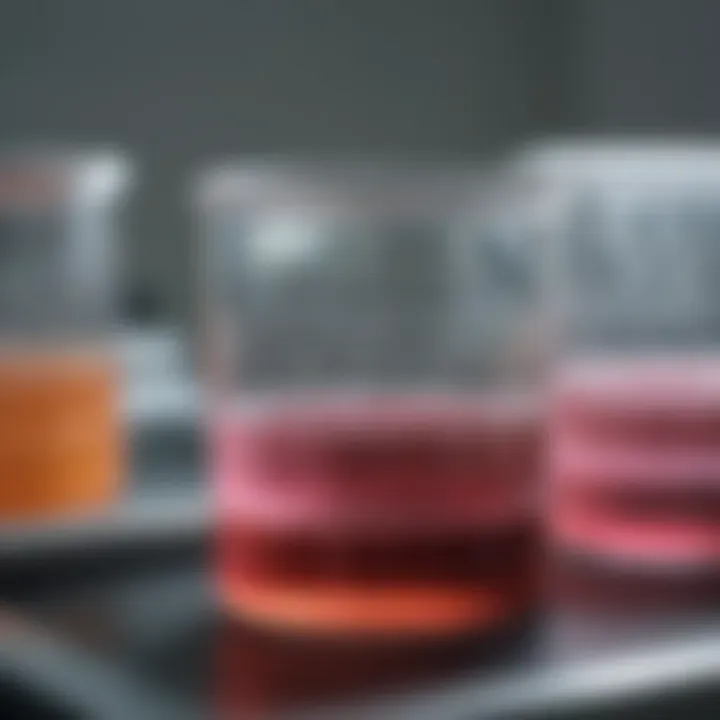
Intro
Agarose gel electrophoresis is fundamental in molecular biology research. It provides a reliable technique for analyzing DNA and RNA, crucial for various applications such as genotyping, sequencing, and cloning. The right agarose gel recipe is central to the success of these processes. This guide aims to present a thorough overview of agarose gel preparation, relevant methodologies, and practical applications.
Research Overview
Key Findings
Research indicates that the choice of agarose concentration significantly affects the resolution of nucleic acids. Typically, lower concentrations of agarose are preferable for resolving larger DNA fragments, while higher concentrations work better for smaller fragments. Adapting gel composition to specific research needs can lead to improved separation and identification of biomolecules.
Study Methodology
The methodologies discussed in this guide arise from a combination of experimental protocols and laboratory practices developed over the years. Many researchers rely on standard recipes, though variations exist based on individual experimental requirements. Accompanying these recipes are tips for optimization and troubleshooting, ensuring effective results in the lab.
Background and Context
Historical Background
The development of agarose gel electrophoresis traces back to the late 20th century when it became a popular tool in genetic research. Originally, polyacrylamide gels were predominantly used; however, agarose emerged as a biopolymer that provided advantages in terms of ease of use and lower toxicity. Researchers have since refined gel recipes and electrophoresis techniques to enhance efficiency and resolution.
Current Trends in the Field
In contemporary research, agarose gels continue to evolve. There is a growing interest in modifying standard agarose with various additives to improve performance under specific experimental conditions. For example, the incorporation of reactive dyes allows for enhanced visualization of nucleic acid bands. Additionally, many researchers are investigating the use of alternative gelling agents. These include agarose derivatives that may offer unique properties beneficial for specialized applications.
"Understanding agarose gel recipes is crucial for ensuring reproducibility and accuracy in molecular biology experiments."
With background context set, the guide will proceed to detail agarose gel recipes, common pitfalls, and troubleshooting methods.
Prelims to Agarose Gel Electrophoresis
Agarose gel electrophoresis is a foundational technique in molecular biology that allows for the separation of nucleic acids and proteins. This method utilizes a gel matrix made from agarose, a polysaccharide derived from seaweed, to provide a medium through which charged molecules can migrate under the influence of an electric field. The importance of agarose gel electrophoresis cannot be overstated, as it serves as an essential tool for analyzing the size, purity, and quantity of nucleic acids such as DNA and RNA.
In this section, we will explore its historical background and significance to the field of molecular biology, examining how this technique has evolved over time and the critical role it plays in various applications.
Historical Background and Development
The origins of agarose gel electrophoresis date back to the early 1970s when researchers sought better methods for DNA analysis. Traditional techniques, primarily using polyacrylamide gels, had limitations including toxicity and difficulty in preparation. Agarose emerged as a safer alternative, allowing for easier handling and preparation, making it more accessible for widespread laboratory use.
Early studies demonstrated that agarose could separate large DNA fragments effectively, which was particularly useful for genetic analysis, cloning, and sequencing applications. Since then, advancements in gel formulation, as well as improvements in electrophoresis equipment, have enhanced resolution capabilities and decreased the time needed for results. The method has become integral to various research disciplines, including genetics, microbiology, and forensic science.
Importance in Molecular Biology
Agarose gel electrophoresis offers several benefits that underline its significance in molecular biology. Firstly, it provides high resolution for separating nucleic acids based on size. This makes it ideal for applications such as:
- DNA Fragment Analysis: Crucial for genetic fingerprinting and restriction fragment length polymorphism studies.
- PCR Product Verification: Ensures that the polymerase chain reaction is successful and that only the desired product is amplified.
- RNA Integrity Assessment: Important for confirming the quality of RNA before proceeding with further experiments.
Moreover, the versatility of agarose gels extends to their modification, allowing researchers to tailor gel compositions for specific applications. For example, varying the agarose concentration can optimize resolution for small or large fragments. This flexibility significantly contributes to the method's popularity.
In summary, agarose gel electrophoresis has evolved into a cornerstone technique in molecular biology. Its historical development has paved the way for numerous applications, making it a critical component in research and diagnostics.
Understanding Agarose
Agarose is a crucial substance in molecular biology, particularly in the field of gel electrophoresis. Understanding its properties and composition is essential for researchers aiming to achieve accurate results in their experiments. Agarose serves as the matrix through which nucleic acids or proteins migrate when subjected to an electric field. This section delves into the chemical composition and physical properties of agarose, shedding light on its advantages and considerations that researchers must be aware of when choosing agarose for their experiments.
Chemical Composition
Agarose is derived from agar, which is extracted from red algae. Its core chemical structure consists of a linear polymer of repeating units of agarobiose, a disaccharide formed by 3,6-anhydro-L-galactose and D-galactose. This composition is important as it influences agarose's gel-forming ability and its subsequent gel characteristics, such as porosity and melting temperature. A crucial aspect to note is that the variability in the source of agar may result in differences in purity and properties. For applications requiring precise results, researchers should choose high-purity agarose. The purity of agarose affects not only the clarity of the gel but also the fidelity of molecular separation.
Physical Properties
Agarose has distinct physical properties that make it suitable for various electrophoresis applications. One critical property is its gel strength, which varies with agarose concentration. Higher concentrations yield firmer gels useful for resolving smaller DNA fragments, while lower concentrations produce softer gels ideal for larger fragments. Additionally, agarose is temperature-sensitive; it forms gels when cooled below its gelling temperature, typically around 35-40°C. This characteristic allows for easy handling during preparation.
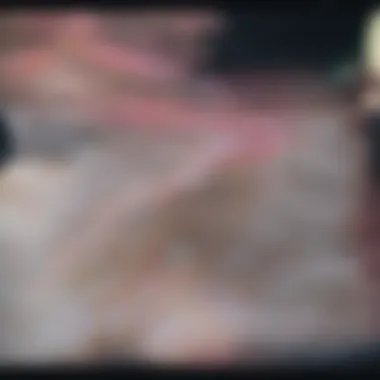
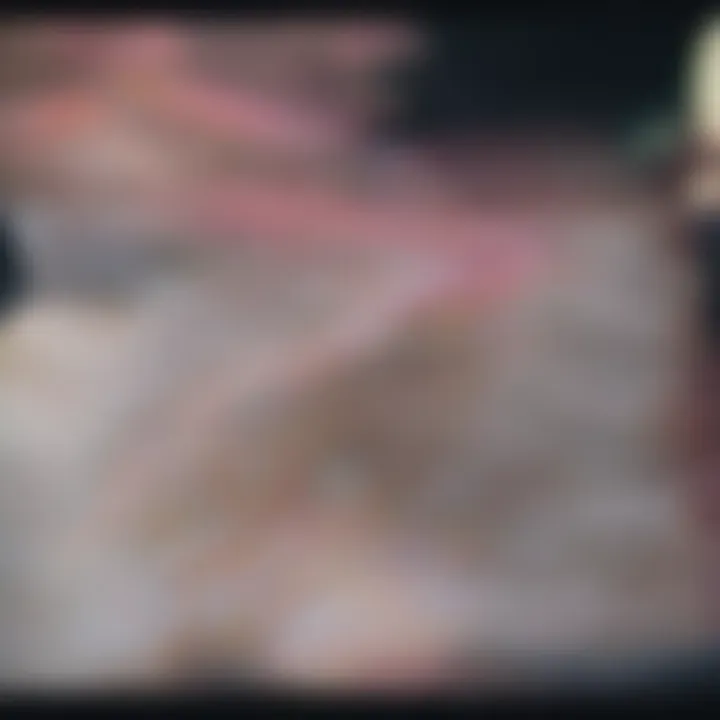
Moreover, agarose gels exhibit low electroendosmosis, resulting in reduced background noise during electrophoresis. This trait is particularly beneficial for the resolution of nucleic acids. Some other physical properties to consider include:
- Melting temperature: Agarose gels can revert to liquid form when heated, allowing for easy re-use of the gel plates.
- Porosity: The pore size can be modified by adjusting the agarose concentration, which impacts the separation efficiency of nucleic acids.
Agarose gels demonstrate good clarity as well, enabling effective visualization of bands after staining. This clarity enhances both qualitative and quantitative analysis during gel electrophoresis.
In synthesis, comprehending the chemical and physical properties of agarose is indispensable for its effective application in molecular biology experiments. The knowledge derived from this understanding guides researchers in selecting the appropriate type of agarose and configuring their gel recipes according to specific experimental needs.
Components of Agarose Gel Recipes
Understanding the components that make up agarose gel recipes is crucial for anyone involved in molecular biology. Each element contributes to the overall functionality and reliability of the gel, impacting the clarity and resolution of the results obtained from electrophoresis. A thorough grasp of these components ensures that researchers can tailor their gel recipes to specific requirements, achieving desired results in experiments involving DNA or RNA analysis.
Agarose Powder
Agarose powder serves as the primary component in agarose gel recipes. This polysaccharide is derived from agar, which comes from the cell walls of red algae. It is significant due to its ability to form a gel when dissolved in boiling water and then cooled. For most applications, agarose concentrations typically range from 0.5% to 2%. The choice of concentration greatly depends on the size of the DNA fragments being analyzed.
High concentrations facilitate the resolution of smaller fragments, while lower concentrations are better suited for larger fragments. Moreover, the purity of agarose powder should not be overlooked. Higher-grade agarose, such as SeaKem GTG or Agarose-LM, yields clearer and more reliable results. It is paramount to use high-quality agarose to reduce background noise and enhance visibility in detection methods.
Buffer Solutions
Buffer solutions play an essential role in agarose gel electrophoresis. Primarily, they maintain a consistent pH during the process, which is vital for preserving nucleic acids' stability and charge. Commonly used buffers include Tris-acetate-EDTA (TAE) and Tris-borate-EDTA (TBE). Each buffer has unique properties that make it suitable for different applications.
For example, TAE buffer is generally used for procedures requiring the separation of larger DNA fragments, whereas TBE is more suited for the separation of smaller fragments due to its higher buffering capacity. When formulating buffer solutions, it is also important to consider electrolyte concentration, as this can influence the current and conductivity during electrophoresis.
Staining Dyes
Staining dyes are crucial for visualizing the DNA or RNA in agarose gels. Common choices for nucleic acid staining include ethidium bromide, SYBR Safe, and GelRed. Each of these dyes has different properties, which influence their effectiveness and safety profiles. For example, ethidium bromide intercalates between base pairs, displaying fluorescence under UV light, but it is also a potent mutagen. On the other hand, SYBR Safe poses less risk to health and presents a safer alternative without sacrificing performance.
When selecting a staining dye, it is important to balance efficacy and safety. The choice also depends on the downstream applications, as some dyes may interfere with subsequent analyses if not properly removed. Understanding the characteristics and implications of staining dyes is essential for achieving accurate results in gel electrophoresis.
The selection of appropriate components is essential for optimizing agarose gel recipes, which directly impacts the quality and reliability of your experiment.
In summary, the components of agarose gel recipes—agarose powder, buffer solutions, and staining dyes—each play a pivotal role in determining the quality of electrophoresis results. Mastering the intricacies of these elements allows researchers to produce high-quality gels, facilitating precise analysis of nucleic acids.
Basic Agarose Gel Recipe
The basic agarose gel recipe serves as a foundational aspect in the field of molecular biology. Understanding this formula is essential for researchers looking to analyze DNA or RNA fragments effectively. A well-prepared agarose gel enables accurate separation of nucleic acid fragments based on size, which is critical for many molecular techniques. The simplicity of this recipe allows for its widespread adoption, making it a common choice in laboratories worldwide. This section covers the step-by-step preparation process, considerations for the selection of agarose concentration, and essential equipment needed for execution.
Step-by-Step Preparation
Selecting Agarose Concentration
Selecting the right agarose concentration is crucial for effective gel formation. The concentrations typically range from 0.5% to 3.0%, depending on the size of the DNA fragments being analyzed. For analyzing large fragments, a lower concentration may be more suitable, while smaller fragments may require a higher concentration. This flexibility makes agarose gels appealing as they can be tailored to specific experimental needs. One advantage of adjusting the concentration is that it affects the gel's porosity, which in turn influences the migration speed of the nucleic acids during electrophoresis. Being mindful of this helps to optimize results.
Mixing Components
Mixing the agarose powder with buffer is another vital step in preparing the gel. A common approach involves adding the agarose to a TAE or TBE buffer solution. The careful blending of these components ensures that the agarose dissolves completely, preventing any lump formation that can affect the gel’s integrity. This process is beneficial because it directly impacts the uniformity and transparency of the gel, which are key properties for effective electrophoretic separation. Any inconsistency in mixing might lead to poor resolution or band distortion.
Melting Agarose
Melting agarose is a critical stage in the preparation of the gel. After mixing, the agarose solution must be heated to its melting point, typically around 90 °C. This process facilitates the complete dissolving of the agarose, allowing it to form a homogenous solution. This step is beneficial as it ensures that the agarose will set uniformly once cooled. However, one must be cautious not to overheat the solution to avoid degradation of the agarose, which can compromise its performance.
Cooling and Solidification
Cooling the melted agarose is the final step in the gel preparation process. The solution must be allowed to cool to about 50 °C before pouring it into the gel mold. This temperature ensures that the agarose does not solidify too quickly, which can lead to uneven gel formation. Allowing for proper solidification is crucial for obtaining a gel with consistent thickness and smoothness. Improper cooling can create flaws that could hinder the separation efficiency of the nucleic acids during electrophoresis.
Equipment Needed
A variety of equipment is essential for preparation and use of agarose gels. Some key items include:
- Microwave or Hot Plate for melting agarose.
- Gel Casting Tray for shaping the gel.
- Comb for creating wells in the gel.
- Power Supply for conducting electrophoresis.
- Gel Documentation System for analyzing results.
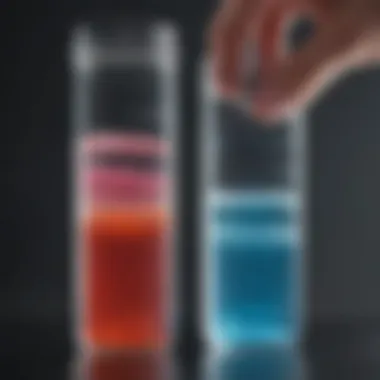
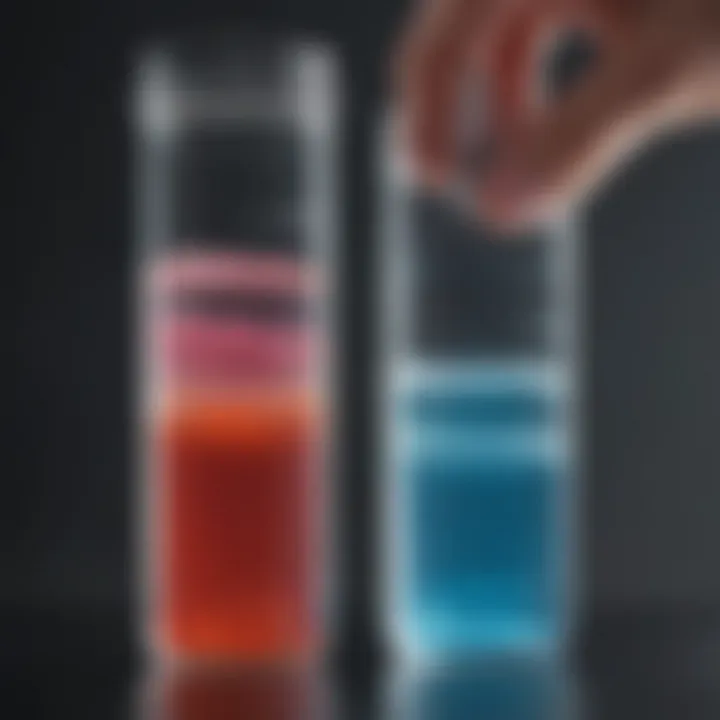
Understanding these components contributes to setting up effective experiments without unnecessary complications. Having the proper equipment enhances the overall efficiency and reliability of the results achieved through agarose gel electrophoresis.
Variations of Agarose Gel Recipes
Agarose gel electrophoresis is a versatile technique, and the choices researchers make in gel preparation can significantly affect their results. The variations in agarose gel recipes are not mere preferences; they determine the resolution, migration rates, and overall efficacy of the electrophoresis process. This part of the article delves into three notable types of agarose gels: high-resolution agarose gels, low melting point agarose gels, and agarose gels specifically tailored for RNA analysis.
High-Resolution Agarose Gels
High-resolution agarose gels are designed for the precise separation of DNA fragments based on size. Researchers often utilize these gels when analyzing PCR products, restriction enzyme digests, or when investigating complex mixtures of DNA. The key advantage of high-resolution gels lies in their ability to resolve very small differences in fragment size. Employing higher concentrations of agarose, typically 2% to 4%, allows for improved resolution.
Considerations for Preparation:
- Concentration Selection: Higher agarose concentration provides finer resolution. However, this can also make the gel stiffer and more difficult to handle.
- Buffer System: The choice of buffer, such as TAE or TBE, can influence the separation capability during electrophoresis. Each buffer has distinct ionic strength and pH properties.
- Temperature Control: Maintaining an optimal temperature during electrophoresis is critical, as it prevents overheating that may affect resolution.
Low Melting Point Agarose
Low melting point agarose is especially useful when researchers need to recover nucleic acids from the gel after electrophoresis. Its melting temperature is lower than standard agarose, which allows for easy melting and retrieval of samples without damaging them. This type of agarose is critical in applications where the integrity of the nucleic acid is paramount.
Key Features:
- Melting Temperature: Usually between 65°C to 70°C, making it suitable for working with sensitive samples.
- Applications: Commonly used in cloning and sequencing experiments, as well as reverse-transcription work.
- Gel Handling: Care should be taken during the cooling process to ensure the gel does not solidify before samples are loaded.
Agarose Gel for RNA Analysis
Agarose gels for RNA electrophoresis need special considerations due to the nature of RNA. RNA is more susceptible to degradation than DNA, thus the integrity of the gel is critical for accurate analysis. Gels used for RNA analysis often incorporate specific stabilizers or dyes to enhance visualization under UV light.
Important Considerations:
- Formaldehyde or MOPS Buffer: Instead of using traditional TAE or TBE buffers, a system using MOPS is often preferred to avoid RNA degradation.
- Loading Buffer: Incorporating an RNA-loading dye, such as ethidium bromide or SYBR Green, aids in the visualization of the RNA bands during electrophoresis.
- Gel Thickness: Thinner gels (around 1% or 1.2% agarose) may enhance the migration of RNA, yielding clearer separation of bands.
The choice of agarose gel type fundamentally influences both the resolution and the usability of the results in nucleic acid analysis.
Common Problems and Troubleshooting
In molecular biology, agarose gel electrophoresis is a crucial technique. However, various issues can arise during its execution. Understanding common problems and how to troubleshoot them serves multiple benefits, including increasing reliability and reproducibility of results. Addressing these challenges is vital for both novice and experienced researchers alike.
Issues with Gel Quality
Gel quality is essential for obtaining accurate results in electrophoresis. Several factors can affect gel quality, such as the concentration and purity of agarose powder. Using low-quality agarose may produce gels with poor visual clarity. This affects the band resolution during electrophoresis.
Another important element is the presence of bubbles. Bubbles trapped in the gel matrix can obscure DNA bands. Therefore, it is important to pour agarose slowly and evenly to minimize trapping air. If bubbles are detected, one can gently run a pipette tip through the gel to dislodge them.
Moreover, it is crucial to ensure that the gel is allowed to solidify properly before loading samples. An underprepared gel can lead to bands diffusing out instead of remaining sharp and defined. To avoid this, one must ensure that the preparation temperature is adequate before pouring.
Electrophoresis Problems
Electrophoresis problems can manifest in various ways, impacting the overall efficacy of the technique. One typical issue is the voltage applied during electrophoresis. If the voltage is too high, it can cause excessive heating, which may result in gel melting or smearing of bands. It is advisable to work within the recommended voltage range.
Staining issues can also be a significant concern. For instance, if staining dyes are not prepared correctly or are not of high quality, the bands may not visualize well. Proper dye selection is critical, as some dyes interact better with specific nucleic acids than others.
Lastly, automation equipment can sometimes malfunction, causing inconsistencies during electrophoresis. Regular maintenance of equipment, including ensuring that gel boxes and power supplies are functioning correctly, can greatly reduce these risks.
"Troubleshooting is not just fixing the problem; it is understanding both the method and its application in detail."
By addressing common problems and learning effective troubleshooting strategies, researchers can enhance their agarose gel electrophoresis techniques. This assurance can improve the confidence in their results, leading to better research outcomes.
Optimizing Agarose Gel Performance
Optimizing agarose gel performance is essential for achieving reliable and reproducible results in molecular biology experiments. The quality of separation, resolution, and clarity of bands depend significantly on several factors. This section delves into the key elements that influence agarose gel efficacy and offers strategies to enhance performance. A focused approach can lead to better data interpretation and successful outcomes in various applications ranging from DNA fragment analysis to RNA integrity assessment.
Adjusting Agarose Concentration
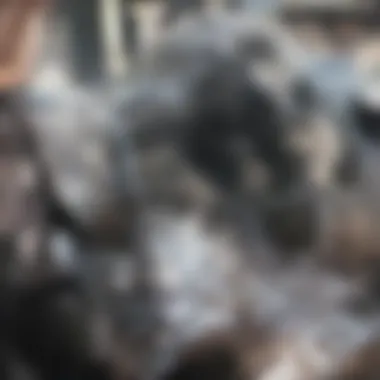
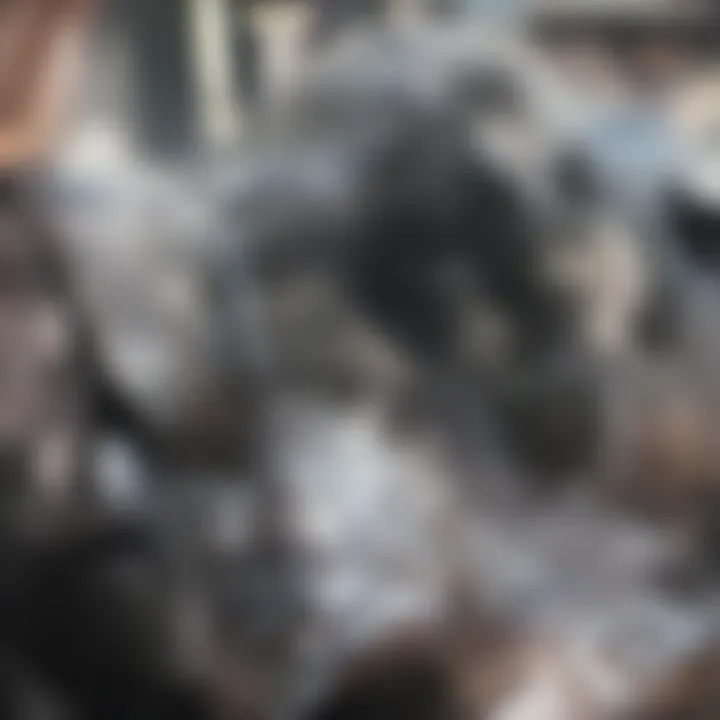
Agarose concentration plays a critical role in optimizing gel performance. Higher concentrations create a denser gel matrix, which is preferable for resolving smaller DNA fragments. Conversely, lower concentrations allow for better separation of larger fragments. Selecting the right agarose concentration is crucial in DNA fragment analysis. Common concentrations range from 0.5% to 2.0%. Researchers need to balance between resolution and the size of the target fragments.
To adjust agarose concentration:
- Evaluate the size of the DNA fragments to be separated.
- Use gradient gels if working with a wide size range. This technique allows different regions of the gel to have varying concentrations.
Buffer System Considerations
The buffer system used during electrophoresis is vital. A buffer maintains pH levels and conductivity, ensuring optimal separation and preventing distortion of DNA bands. Common buffers include Tris-Acetate-EDTA (TAE) and Tris-Borate-EDTA (TBE). Each has specific advantages depending on the application.
Choosing the buffer system involves several factors:
- TAE is generally better for larger DNA fragments because it provides improved resolution over longer runs.
- TBE is suited for smaller fragments and can maintain stability over extended periods.
It is important to prepare the buffer solutions accurately, checking for contamination that can affect gel performance. Furthermore, the buffer volume should be sufficient to cover the gel surface adequately during the run.
Temperature and Electrophoresis Settings
Temperature can greatly impact agarose gel electrophoresis. Heat generated during electrophoresis can affect the gel's behavior, leading to melting or band distortion. So, maintaining proper temperature settings is necessary. Performance also hinges on voltage settings during electrophoresis; too high a voltage can cause excessive heat and poor resolution.
For optimal results:
- Operate at lower voltage settings for longer periods to achieve better separation. This minimizes heat and allows better clarity in bands.
- Monitor the gel for signs of overheating, implementing cooling methods if necessary.
Achieving optimal performance in agarose gel electrophoresis requires careful adjustment of agarose concentration, buffer system selections, and controlling temperature and voltage settings during electrophoresis. Attention to these factors contributes to the effectiveness of the method in analyzing nucleic acids.
Applications of Agarose Gel Electrophoresis
Agarose gel electrophoresis serves as a foundational technique in molecular biology. It enables researchers to separate and analyze nucleic acids based on size, providing crucial insights into genetic material. This versatile method has various applications, each playing a significant role in research and diagnostics.
DNA Fragment Analysis
DNA fragment analysis is one of the most common applications of agarose gel electrophoresis. This technique is essential for characterizing DNA samples obtained from various sources. In practices such as genetic fingerprinting, the separation of DNA fragments through agarose gel allows for the comparison of specific alleles or bands from different samples.
Researchers can assess the size of DNA fragments, which can indicate the possible presence of mutations, deletions, or insertions. Moreover, quantifying the intensity of bands provides information on the amount of DNA present in each fragment. This is particularly crucial in studies involving genetic diversity in populations or forensic analysis.
PCR Product Verification
While polymerase chain reaction (PCR) is a powerful method for amplifying specific DNA sequences, verifying the success of this amplification is crucial. Agarose gel electrophoresis plays a vital role in PCR product verification. By loading PCR products onto agarose gels, researchers can ascertain whether the desired DNA fragments have been successfully amplified.
Visualizing the bands on the gel allows for the assessment of product size against a DNA ladder or marker. This comparison helps in confirming the presence of the target sequence. Additionally, it can reveal issues such as non-specific amplification or primer-dimer formations, which may affect the reliability of subsequent analyses.
RNA Integrity Assessment
In molecular biology, assessing RNA integrity is critical for various applications, including gene expression studies. Agarose gel electrophoresis is an effective method for evaluating RNA quality. When RNA samples are denatured and loaded onto a gel, the distinct bands corresponding to ribosomal RNA can be observed.
The intensity and sharpness of these bands provide information about RNA degradation. Well-preserved RNA will show clear, intact bands, while partially degraded RNA will result in smeared patterns. Researchers can use this information to determine whether RNA samples are suitable for downstream applications, such as reverse transcription or sequencing.
"Agarose gel electrophoresis is a critical technique for anyone studying nucleic acids, offering a clear picture of DNA and RNA quality and quantity."
Future of Agarose Gel Techniques
The landscape of molecular biology is continuously evolving, and agarose gel electrophoresis remains a critical technique. The future of agarose gel techniques focuses on innovation and efficiency. As research progresses, newer methodologies and technologies emerge. This evolution brings about improved accuracy, quicker results, and enhanced usability. For both students and professionals, stay updated on these advancements is crucial for optimizing their experiments.
Emerging Technologies
Emerging technologies in agarose gel techniques are revolutionizing molecular biology. Innovations in gel formulations are designed to enhance resolution and decrease run times. Researchers are developing agarose gels with specialized chemical compositions that improve separation fidelity. For instance, incorporating synthetic polymers or cellulose may directly affect gel permeability. Moreover, advances in detection methods, such as laser scanning and digital imaging, contribute significantly to sensitivity and quantitation. These developments grant scientists capabilities previously unattainable with conventional methods.
Key technological advances include:
- Smart Gels: These gels can change properties in response to environmental conditions, allowing for adjustable separation dynamics.
- Nano-technology Applications: Utilizing nanoparticles to improve the polymer structure for better resolution and strength.
- Real-time Monitoring Tools: Implementing sensors to track the electrophoresis process, facilitating more dynamic experimentation.
Integration with Automation
Automation of agarose gel electrophoresis is steadily gaining traction. This integration allows labs to handle more samples and achieve greater consistency in results. Automated systems minimize human error and variability in gel preparation, sample loading, and electrophoresis execution. With well-designed robotic systems, laboratories can run multiple electrophoresis experiments simultaneously, reducing overall time and manpower.
A few benefits of automation include:
- Consistency: Automated techniques allow for uniform gel preparation, reducing batch-to-batch variations.
- Efficiency: High-throughput capabilities enable labs to process large volumes of samples without substantial manual input.
- Data Management: Integration with software solutions can enhance data collection and analysis, resulting in better tracking of experimental results.