Exploring Boron Ionization: Principles and Applications
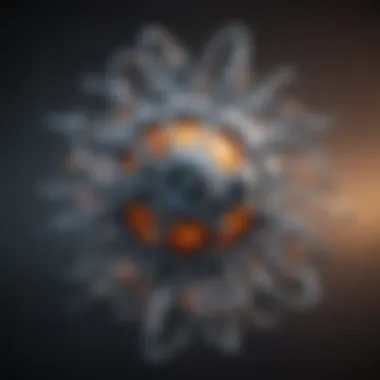
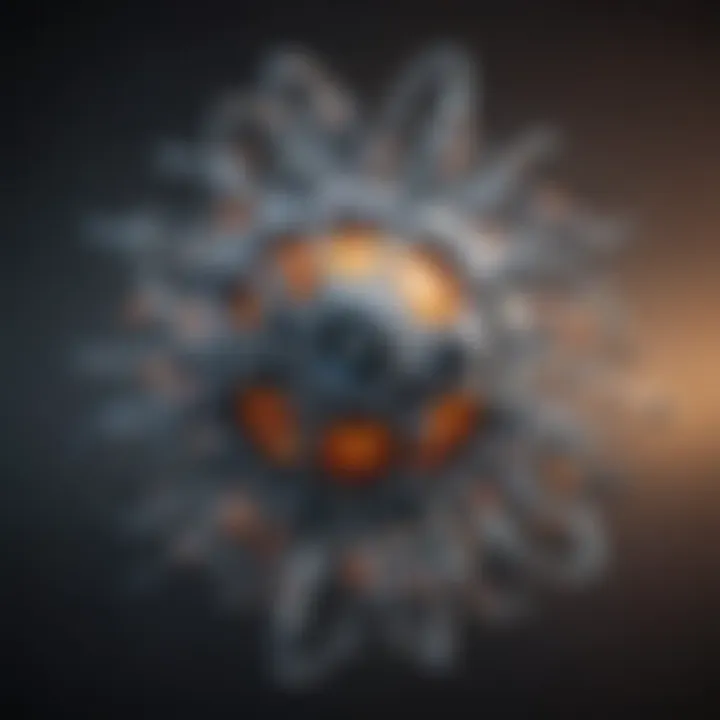
Intro
Understanding the ionization of boron necessitates a deep dive into its fundamental properties and behaviors. This element, while often overlooked amidst its more glamorous counterparts, plays a crucial role in several scientific domains. From its historical significance to its cutting-edge applications in today’s advanced technology, boron continues to draw the attention of researchers and professionals alike.
Research Overview
Key Findings
The exploration of boron ionization reveals striking insights, particularly in its reactivity and the methods used to study its ionization process. Researchers have identified that the ionization energies of boron are intricately tied to its electronic configuration. Key findings in the realm of boron ionization illustrate that its capability to form stable compounds in various oxidation states makes it a versatile element, crucial for myriad applications.
"Boron’s unique position in the periodic table is a double-edged sword; it exhibits both metallic and non-metallic characteristics, making it invaluable in numerous fields."
Study Methodology
In investigating the ionization of boron, scientists employ a mix of quantum mechanical models and experimental techniques. Notably, photoelectron spectroscopy stands out as a vital tool for studying the energy states of boron ions. This method allows for a detailed analysis of electron configurations and is pivotal in understanding the elemental behavior of boron under different conditions.
Background and Context
Historical Background
Boron was discovered in the early 19th century, and its use has evolved over time. Initially recognized for its role in reducing melting points, boron soon found significance in glassmaking and agriculture. This historical utilization laid the groundwork for deeper scientific inquiry into its ionization properties.
Current Trends in the Field
Today, boron ionization is at the forefront of several innovative applications, particularly in materials science and medicinal chemistry. Research is increasingly focused on the synthesis of boron-containing compounds, given their unique properties that impact drug design and development. Furthermore, advancements in nanotechnology continue to highlight boron’s potential in creating novel materials with enhanced thermal and electrical conductivity.
In this exploration, the dimensionality of boron ionization becomes apparent, touching on principles that underlie its behavior in both natural and controlled environments. Understanding these nuances is essential for a comprehensive grasp of how boron can be leveraged for future breakthroughs in diverse scientific fields.
Prelims to Boron
Boron, often overlooked in favor of its more flashy counterparts like carbon or nitrogen, serves as a pivotal cornerstone in the landscape of modern chemistry and industry. A key player in various chemical reactions, understanding boron is essential—not just for chemists but also for researchers across fields like materials science, agriculture, and even medicine. This introduction lays the groundwork for a deeper exploration into boron’s unique properties, its ionization process, and its far-reaching applications.
Overview of Boron Chemistry
Boron is a metalloid, characterized by its intermediate properties between metals and nonmetals. This duality imparts it with several fascinating chemical behaviors. It readily forms covalent network solids, which are compounds where atoms are interlinked in a continuous structure. One notable compound is boron carbide, known for its hardness, which is second only to diamond. Also, boron is prevalent in its anionic form, such as borate, commonly found in nature and used in various applications.
The essential role of boron chemistry extends into the world of complex organic reactions, often acting as a catalyst or a reagent to facilitate the formation of new chemical bonds. For instance, boron compounds like boranes are utilized in organic synthesis to create complex molecules swiftly. This overview underscores how boron acts as a bridge in creating innovative materials and substances crucial for technological advancement.
Historical Context of Boron Research
Boron has a rich history that intertwines with the advancement of science. First isolated in 1808 by Sir Humphry Davy, its properties and potential were not immediately recognized. It wasn’t until the late 19th century that the significance of boron was truly appreciated, particularly with the onset of industrial chemistry. Its application in glassmaking and ceramics gave birth to the borosilicate glass that we use in laboratories today.
Moreover, the mid-20th century introduced boron’s role in nuclear applications where it becomes critical for controlling neutron flow due to its absorption properties. This time frame not only marked the scientific appreciation of boron but also established a foundation for ongoing research.
The growing body of research throughout the decades highlights boron’s versatility and hints at the remarkable properties that lay waiting to be discovered. This historical context of exploration forms an essential backdrop as we delve into the ionization processes and modern applications of boron, understanding how past discoveries shape contemporary scientific inquiry.
Ionization Fundamentals
The exploration of ionization fundamentals lays the critical groundwork for a comprehensive understanding of boron's behavior at an atomic level. Ionization refers to the process by which an atom or a molecule gains or loses electrons, leading to the formation of charged particles known as ions. This phenomenon holds significance not just in theoretical physics and chemistry but also in numerous practical applications that impact our daily lives. Throughout this section, we will delve into the definition and energy concepts, illuminating their relevance to boron's unique ionization pathways.
Definition of Ionization
At its core, ionization is about creating charged particles, which are pivotal in various chemical processes. When an electron is removed from an atom or molecule, it typically results in a positively charged ion, known as a cation. Conversely, gaining an electron leads to the formation of a negatively charged ion or an anion. This balance of charge is essential to many chemical reactions, whether they happen in nature or in industrial settings.
Ionization can happen in multiple ways: through thermal energy—where heat provides the energy necessary for electrons to escape the attractive pull of the nucleus—or through the absorption of electromagnetic radiation, such as light. Each method highlights how different environments can influence an atom's propensity to become ionized. In boron's case, understanding these processes is fundamental because of its peculiar electron configuration.

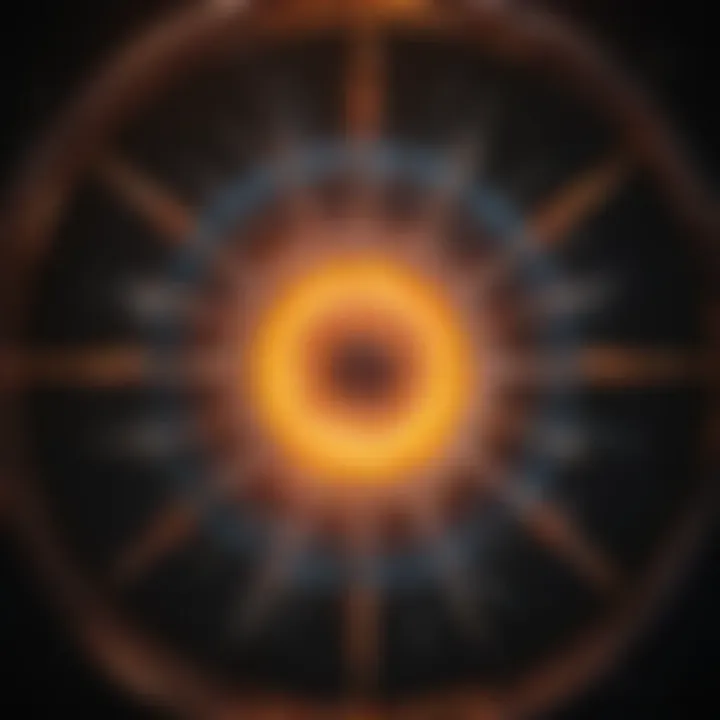
The Ionization Energy Concept
Ionization energy is the energy required to remove an electron from an atom. It varies from one element to another and directly reflects the element's stability and the strength of its electron-nucleus attraction. For boron, with its three electrons in the outer shell, this energy is modest yet significant. As boron atoms strive to achieve stability—typically by losing or sharing electrons—they demonstrate certain distinctive behaviors during ionization.
When we speak of ionization energy in the context of boron, we must consider several factors:
- Atomic Structure: Boron's electron configuration influences how readily it can lose an electron.
- Electronegativity: This concept, which deals with an atom's ability to attract and hold onto electrons, plays a role in how strongly boron holds its electrons.
- Environmental Conditions: Factors such as pressure, temperature, and the presence of other elements can significantly impact the ionization process.
Understanding the nuances of ionization energy is vital for comprehending many aspects of boron's chemical behavior, especially in reactions, materials science, and even biological contexts.
In summary, recognizing both the definition and the energy associated with ionization provides a benchmark for studying boron's properties and its interactions with other elements. The fundamental principles discussed here are the stepping stones to appreciating boron's unique role in various scientific fields.
Boron’s Unique Properties
Boron is no ordinary element; it possesses a set of unique properties that set it apart from its peers in the periodic table. Understanding these characteristics is crucial for comprehending how boron interacts with other elements and compounds, and how these interactions can be harnessed across various fields, from materials science to medicine.
Physical and Chemical Characteristics
Boron is classified as a metalloid, exhibiting properties that are both metallic and non-metallic. It has a relatively low density, around 2.34 grams per cubic centimeter, making it lighter than many metals. Its melting point, standing at about 2075 degrees Celsius, is notably high, which speaks volumes about its stability when subjected to high temperatures. This unique thermal property is particularly beneficial in various industrial applications.
In terms of color and allotropy, boron comes in multiple forms, including amorphous and crystalline varieties. The crystalline form—often found in nature as borax—displays a shiny, metallic luster that intrigues researchers and manufacturers alike. Its intricate bonding capabilities, especially with oxygen and hydrogen, lead to the formation of a variety of boron compounds that are essential in both agricultural fertilizers and glass manufacturing.
Notable characteristics of Boron include:
- High Ionization Energy: This property makes boron less likely to lose electrons easily, allowing it to form stable bonds.
- Covalent Bonding: Boron tends to form covalent bonds, further revealing its behavior as either a donor or acceptor of electrons, depending on the situation.
- Semiconductor Properties: Certain boron compounds act as semiconductors, which has open doors in the electronics field.
These physical and chemical characteristics of boron make it an intriguing subject for study, while also serving practical applications across different sectors.
Comparative Analysis with Other Elements
When comparing boron's properties to those of other elements, particularly within the same group, boron stands out for its unique characteristics. Take silicon, for instance; both elements are metalloids, yet their behaviors diverge significantly.
- Ionization Energy: Boron has a higher ionization energy than aluminum and gallium, following the trend in the periodic table where ionization energy tends to decrease down the group. This higher energy allows boron to retain its electrons more tenaciously, making it a more stable choice in certain reactions.
- Bonding Behavior: While silicon forms predominantly silicon-oxygen bonds, boron often forms clusters, like boranes in the chemical world, displaying a richness in its bonding. This flexibility in bonding arrangements highlights boron’s versatility and the creative applications it can foster.
- Phase Behavior: At elevated temperatures, boron shows a remarkable ability to remain stable while many metals would melt or react unfavorably. This stability is vital in high-temperature applications, such as in the aerospace industry.
"Boron’s distinctive attributes not only define its behavior in chemical reactions but also its critical role in innovative technologies."
In essence, the unique properties of boron tilt the scales in its favor across diverse applications. They optimize its use in high-tech industries, agriculture, and even medicine, portraying boron as an indispensable element in modern science and technology.
The Process of Ionization in Boron
The process of ionization in boron is a crucial theme in better discerning the element’s capabilities and its role across various scientific disciplines. Understanding how boron transitions from an electrically neutral state to an ionized form provides insights into its chemical behavior, aiding in both research and practical applications. The ionization of boron is not just a fundamental concept in chemistry; it has implications that stretch across materials science, biomedicine, and even agriculture. This section seeks to unpack the intricate mechanisms involved in ionization, while also addressing the key factors that influence this process.
Mechanisms of Ionization
Ionization of boron can occur through a variety of mechanisms, each tied fundamentally to the structure of the boron atom itself. Boron, with an atomic number of five, possesses three valence electrons located in its outer shell. When these electrons become increasingly energetic—due to external stimuli like heat, radiation, or electrical energy—they can be stripped away from the atom. This phenomenon can be understood through the concept of ionization energy, which delineates the energy needed to remove an electron from a gaseous atom.
- Loss of Electrons: When subjected to sufficient energy, one of those three valence electrons can detach, creating a positively charged boron ion (
- Formation of Negative Ions: Conversely, if boron encounters extra electrons under certain conditions, it can form negative ions, although this is less common in nature compared to the formation of cations.
- Excitation followed by Ionization: In certain cases, electrons may first absorb energy and get excited to higher energy levels. If the excitation is intense enough, subsequent radiation can lead to ionization.
The ionization process in boron thus illustrates how energy transfer can alter an element's state, holding implications for reactions and interactions within various environments.
Factors Influencing Ionization
Several factors play a pivotal role in determining the rates and efficiency of boron ionization. Recognizing these variables is imperative for researchers looking to manipulate boron for specific applications.
- Temperature: Increasing temperature can augment the energy of atomic motion, prompting more efficient electron loss.
- Ionization Energy: As previously mentioned, boron has a specific ionization energy that dictates how easily it can lose electrons. Understanding this helps scientists forecast how boron behaves when introduced to various conditions.
- Chemical Environment: Boron’s interaction with other elements can influence its ionization. Collisions with other atoms or molecules can lead to electron transfer.
- Pressure and Density: In high-pressure scenarios, the likelihood of ionization increases due to frequent particle collisions.
- Type of Ionizing Radiation: Different types of radiation (alpha, beta, gamma) carry unique energies that can result in varying degrees of ionization.
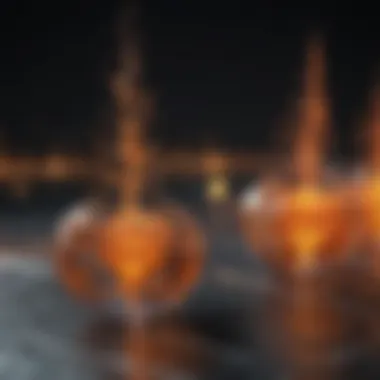
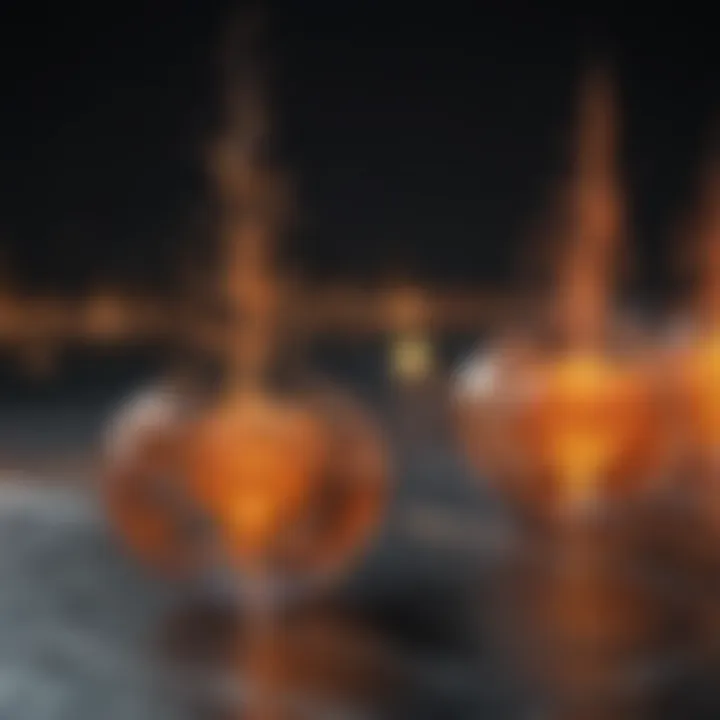
The rate of ionization can significantly vary depending on not just the elemental conditions but the environmental context in which boron resides.
To sum it up, the dynamics of ionization in boron is a complex interplay of atomic structure and environmental factors. This section sheds light on how such processes are rooted not only in theoretical chemistry but also in practical applications, paving the way for future innovations and discoveries.
Experimental Approaches
Research into the ionization of boron necessitates sophisticated experimental approaches that allow for accurate measurement and analysis. The significance of these methodologies cannot be overstated, as they form the backbone of understanding how boron behaves when its electrons are manipulated. By leveraging various experimental techniques, scientists can gain insights into boron's unique properties, its reaction under different conditions, and how it can be applied in both industrial and scientific contexts.
Techniques for Observing Ionization
Understanding ionization begins with effective observation techniques. Various methodologies are employed for this purpose, each with its own set of advantages:
- Mass Spectrometry: This technique allows scientists to determine the mass-to-charge ratio of ions. In doing so, it exposes the energy levels of boron atoms and provides valuable details about their ionization energies.
- Photoelectron Spectroscopy: Often referred to as PES, this method utilizes photons to eject electrons from an atom and measures their kinetic energy. The information gathered reveals the electrostatic environment surrounding boron atoms, shedding light on its electron structure.
- Ionization Energy Measurements: These involve bombarding boron with energetic particles and measuring the energy required to remove an electron. Different conditions can be applied to observe how they affect the ionization process.
- Electron Spin Resonance (ESR): This technique detects unpaired electrons in boron compounds, thus providing insights into the electronic configuration and any ionization events occurring.
Utilizing these various techniques enables researchers to create a more holistic picture of boron’s behavior during ionization processes, leading to developments that can push the boundaries of current understanding.
Synthesis of Boron Ions
Synthesis of boron ions can be seen as an intricate dance between precision and understanding of chemical reactions. Producing boron ions can be done through several methods, including:
- Chemical Vapor Deposition (CVD): This method allows boron to be deposited onto a substrate by means of vaporized chemicals. By controlling the chemical environment, boron ions can be synthesized in a controlled manner, which is critical for semiconductor applications.
- Laser Ablation: Using a focused laser beam, solid boron can be vaporized to create ions. This provides not only high purity boron ions but also allows for greater control over the ion formation process.
- Ion Implantation: In this method, ions of boron are introduced into materials, particularly semiconductors. This is vital for creating p-type materials in electronic devices, showcasing yet another facet of boron’s practical applications.
While synthesizing boron ions, various factors including temperature, pressure, and chemical concentrations play significant roles. Each of these synthesis methods contributes to unique insights into boron's ionization and how it can be effectively utilized in different fields, from electronics to materials science.
"The synthesis of boron ions opens up a myriad of applications that can transform industries, from technology to agriculture. It emphasizes the adaptability of boron in several domains of research and application."
By carefully considering the experimental approaches, one not only unlocks the secrets of ionization but also reveals the broader implications of boron’s functionality in our technological landscape.
Applications of Boron Ions
Boron ions are not just fascinating from a theoretical standpoint; they offer various practical applications that resonate throughout several industries. Understanding these applications illuminates the multifaceted nature of boron ions, emphasizing their invaluable roles in industrial processes, agricultural advancements, and medical innovations. With the contemporary emphasis on sustainability and efficiency, boron ions emerge as significant players in advancing technology and improving human health.
Industrial Uses
Boron ions have carved a niche for themselves in the manufacturing sector. One of the most notable industrial applications is in the production of glass and ceramics, where boron compounds enhance the thermal and chemical durability of products. Borosilicate glass, for instance, is known for its resistance to thermal shock, making it ideal for laboratory glassware and cookware. The introduction of boron ions into glass matrices can improve clarity and strength, pushing the boundaries of what these materials can withstand.
Additionally, boron ions play a crucial role in semiconductor technology. The doping of silicon with boron allows for the modulation of electrical properties, which is fundamental in developing integrated circuits. Boron dopants precisely control conductivity, enabling the intricate functionality required in modern electronics.
The versatility of boron ions also extends to materials intended for aerospace applications. Lightweight composites reinforced with boron provide enhanced strength-to-weight ratios, which are essential for aviation and space exploration, where every gram counts. By integrating boron ions into these materials, manufacturers can create more efficient and robust solutions that stand up to harsh conditions.
Applications in Agriculture
In the agricultural realm, boron ions play an essential role as micronutrients, crucial for plant health. It is involved in cell wall formation and reproduction, making it a vital element for various crops. Without sufficient boron, plants may exhibit poor growth, flower irregularities, and fruit development issues. Fertilizers containing boron ions are therefore commonplace in fields where boron deficiency is a concern. These fertilizers are tailored to ensure that crops receive the necessary amount of boron without leading to toxicity, which can negatively affect yield.
Moreover, boron ions have been found to enhance the effectiveness of pesticides and herbicides. By improving the absorption of these chemical agents, boron ions can augment their efficacy, reducing the overall quantity needed for pest control while minimizing environmental impact. This dual action—nourishing plants while allowing for efficient pest management—highlights boron's significance in modern agriculture.
Role in Medicine and Biology
The medical field also leverages boron ions, particularly in cancer therapy and diagnostics. One promising area is boron neutron capture therapy (BNCT), a targeted treatment that utilizes boron compounds. By incorporating boron ions into cancerous cells, they become susceptible to neutron radiation that selectively destroys these cells while sparing healthy tissue. This targeted approach presents a less damaging alternative to conventional radiation therapy, showcasing the potential of boron ions in advancing medical treatments.
Boron ions are also beneficial in biomedical research, where they serve as catalysts or stabilizing agents in organic reactions. Their ability to complex with organic molecules allows researchers to study biological processes at the molecular level, leading to insights that inform the development of new drugs and therapies.
"The applications of boron ions span across different domains, from enhancing industrial materials to pioneering medical treatments, reflecting their essential role in modern science and industry."
Furthermore, boron-based compounds are investigated for their potential in cognitive health. Recent studies suggest that boron plays a role in brain function, impacting processes such as memory and motor skills. Exploring these possibilities may yield revolutionary insights into neurodegenerative diseases, positioning boron ions as key players in future biomedical advancements.
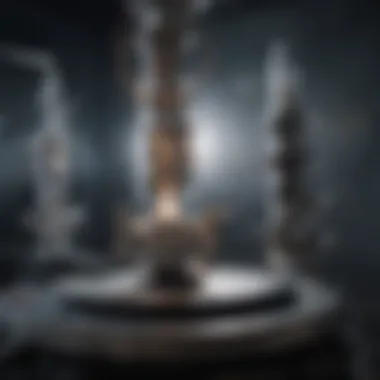
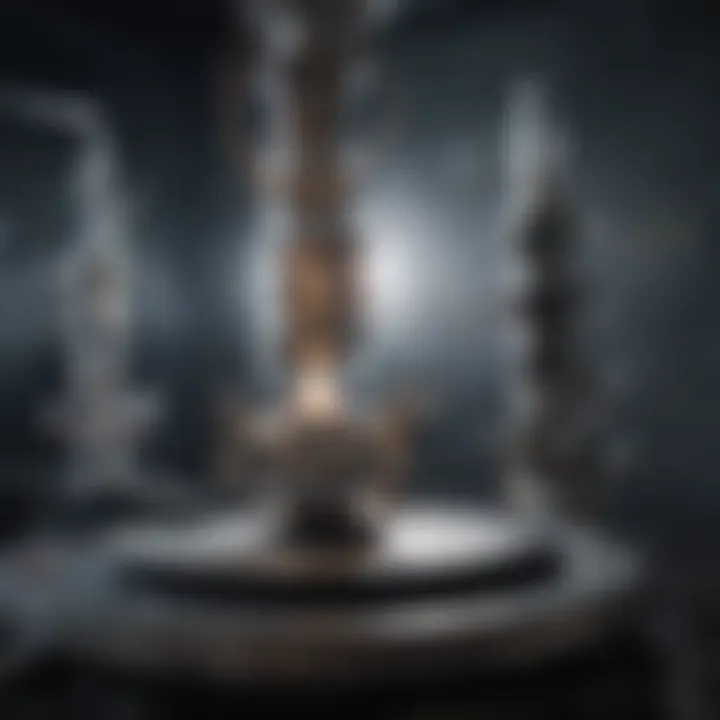
Environmental Considerations
Environmental considerations surrounding the ionization of boron are significant as they encompass both the effects of boron ionization on natural ecosystems and the related safety and toxicity issues that arise within various applications. Boron, while essential in certain quantities for plant growth, can have detrimental impacts when present in excessive amounts. Understanding these elements helps in developing sustainable practices in industrial, agricultural, and medical domains while ensuring ecological balance is maintained.
Impact of Boron Ionization on Ecosystems
When boron ions enter ecosystems, they can influence various processes. For instance, they play a vital role in plant physiology. The right amount of boron helps in cell wall formation and aids in nutrient transport. On the flip side, too much boron can lead to phytotoxicity, where plants exhibit stunted growth and yield reduction. The effects extend beyond plants; in aquatic environments, elevated boron levels can harm fish and other wildlife.
- Soil Health: Boron ionization can alter soil chemistry. High boron concentration in soil can disrupt the availability of other crucial nutrients such as potassium and magnesium.
- Biodiversity: Ecosystems with imbalanced boron levels can witness a decline in species diversity due to selective pressure on sensitive species versus more tolerant ones.
"The balance of nutrients is essential; the introduction of one element cannot come at the cost of another."
Researchers continue to study how environmental boron ion levels can shift with various agricultural practices, including the use of boron fertilizers. Proper management strategies can potentially minimize any detrimental effects on ecosystems while maximizing agricultural productivity.
Safety and Toxicity Concerns
Concerning safety, boron compounds are generally considered relatively low in toxicity in comparison to heavy metals or other more harmful substances. Still, precaution is necessary, particularly in industrial applications.
- Exposure Risks: In occupational settings, individuals working with boron manufacturing or processing may face inhalation risks or skin exposure. Standard hygiene measures are crucial.
- Regulatory Guidelines: Agencies like the Environmental Protection Agency (EPA) and the World Health Organization (WHO) have established standard limits for boron concentrations to ensure public safety. These regulations are vital to protect workers and the surrounding communities.
However, even with these regulations in place, awareness about the accumulation and long-term effects of boron is still developing. Further research is needed to evaluate how boron ions behave in different biological systems and whether they could pose unforeseen health risks, particularly in vulnerable populations and environments. Beyond monitoring, educational outreach to inform workers and communities about potential risks is also paramount to mitigate hazards associated with boron use.
Future Research Directions
As we look towards the horizon of boron research, it becomes increasingly clear that future exploration will be pivotal for various scientific realms. The importance of this research cannot be overstated; it stands at the crossroads of material sciences, environmental studies, and agricultural innovations. By prioritizing specific elements in boron ionization research, we can unlock numerous benefits, ranging from increased efficiency in industrial applications to novel solutions for agricultural sustainability. Such advancements necessitate careful consideration of methodologies, ethical implications, and potential environmental impacts.
Emerging Trends in Boron Research
Currently, the landscape of boron research is shifting dramatically. Several emerging trends signal a robust future for ionization studies. One notable trend is the use of advanced analytical techniques, such as mass spectrometry and NMR spectroscopy, which enable researchers to unravel complex boron compounds and their behaviors under different conditions.
Another crucial trend is the growing interest in nanotechnology, as boron-based nanomaterials are making waves in various fields. For instance:
- Nanoboron: Researchers are exploring its use in drug delivery systems, which could improve the bioavailability of certain treatments.
- Boron-doped silicon: This is gaining traction in the semiconductor industry, where better electronic properties are constantly sought after.
Thus, these dynamic avenues of research ensure that boron remains a subject of endless inquiry, paving the way for novel discoveries.
Potential Innovations in Applications
The potential innovations stemming from boron ionization studies are vast and varied. With applications unfurling in various domains, there are numerous considerations to keep in mind:
- Agriculture: Innovative techniques such as boron-enriched fertilizers are under investigation. These fertilizers can enhance plant growth, particularly in regions with boron deficiency.
- Materials Science: The development of boron-rich ceramics is promising. These materials could revolutionize industries by providing higher strength and thermal resistance than traditional materials.
- Biomedicine: Boron neutron capture therapy (BNCT) is a noteworthy application in cancer treatment. This technique could lead to more targeted therapies, minimizing damage to surrounding healthy tissues.
"The potential for boron applications is just beginning to be tapped; innovative discoveries will likely transform our understanding and utilization of this unique element."
Ending
The conclusion serves as a vital component in understanding the broader implications of boron ionization. By synthesizing the insights gathered from previous sections, it encapsulates the significant ramifications this knowledge holds for various fields.
Summary of Key Insights
The exploration of boron ionization reveals several key takeaways:
- Fundamental Understanding: Ionization defines how boron behaves in its various states, deeply affecting its reactivity and interactions.
- Applications Across Disciplines: Boron ions find merit in industries ranging from semiconductors to agriculture, illustrating the element's versatility.
- Environmental Impact: An understanding of ionization contributes to a better grasp of its environmental dynamics and potential ecological consequences.
- Safety Considerations: The knowledge of how boron ionizes allows for informed approaches in handling its compounds safely, taking into account toxicity and environmental effects.
Final Thoughts on Boron Ionization
In closing, ionization of boron is not merely a scientific curiosity; it is a topic that resonates across various domains, including industrial applications and environmental impact. With emerging research shedding light on innovative uses, the significance of boron as an element will likely grow.
"Understanding the ionization of elements like boron is crucial for paving the way toward future advancements in technology and sustainability."
As we look ahead, the continued study of this element, paired with advancements in analytical methods, promises to unlock new potentials that could change our approach to chemistry and material sciences. Developing a deeper appreciation for boron's properties lays the groundwork for informed decision-making and innovation in applications yet to be imagined.