Chemical Identification: Techniques and Applications
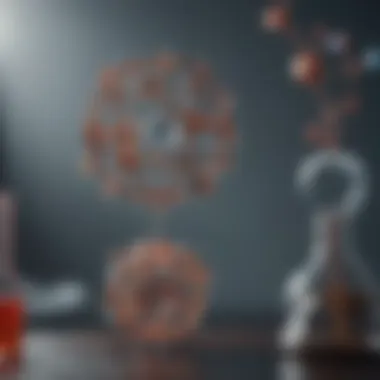
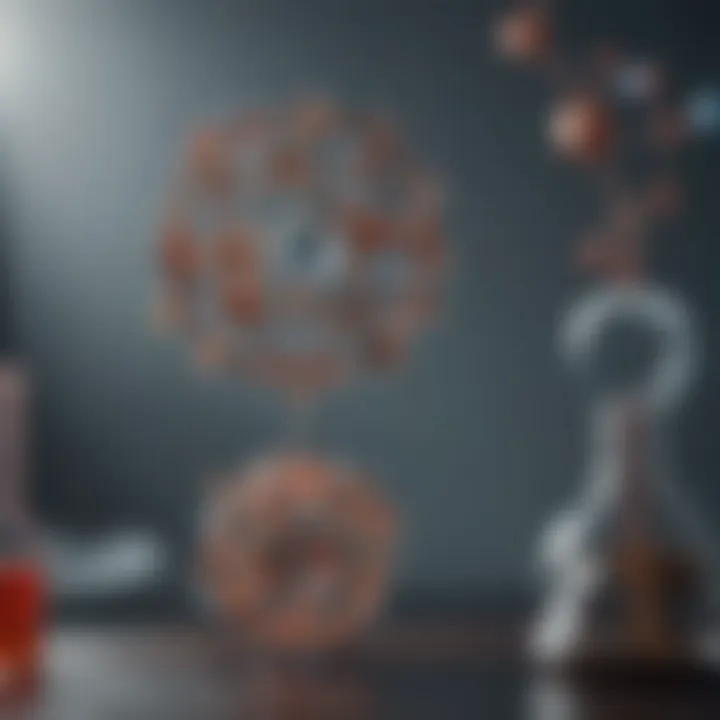
Intro
Chemical identification is an intricate process that serves as a bedrock for multiple scientific disciplines and industries. Accurate identification of chemical substances is essential to ensure safety, effectiveness, and compliance with regulatory standards. This article aims to provide an in-depth view of chemical identification methods, their historical development, current practices, and their significance across various fields such as pharmaceuticals and environmental science.
Research Overview
Understanding the methodologies employed in chemical identification reveals not only the complexity of the task but also its crucial role in advancing science and technology. Through gathering insights from a variety of studies, we can appreciate how different methods have evolved and been integrated into modern practices.
Key Findings
Several key findings emerge from current research on chemical identification:
- Multidisciplinary Approaches: Effective chemical identification often requires collaboration across various disciplines.
- Technological Advancements: Modern techniques such as spectroscopy and chromatography have considerably improved accuracy.
- Regulatory Importance: In fields like pharmaceuticals, proper identification is fundamental for regulatory compliance and public safety.
These findings underline the importance of ongoing research and development in this field.
Study Methodology
The methodologies range from traditional techniques, such as titration and gravimetry, to advanced methodologies like mass spectrometry and nuclear magnetic resonance (NMR). Detailed investigations into these methodologies can provide substantial insights into their effectiveness and limitations.
Background and Context
Historical Background
The quest for identifying chemical substances traces back centuries. Early practices involved cumbersome techniques and manual analyses. Advances in both theory and technology have led to contemporary practices that are more reliable and efficient, allowing for rapid assessments in various settings.
Current Trends in the Field
Today's landscape of chemical identification is marked by:
- Automation: Many laboratories are embracing automated systems to enhance throughput and reduce human error.
- Data Integration: With the rise of computational chemistry, the integration of large data sets and machine learning algorithms is gaining traction, improving prediction and identification accuracy.
- Sustainability: There is a growing interest in identifying green chemicals. This trend aligns with global initiatives to promote environmental sustainability.
Chemical identification remains an essential aspect of scientific research, directly influencing various scientific fields. The ongoing advancements in methodology and technology not only enhance our understanding but also ensure the safe and effective use of chemicals in society.
"In the world of chemical research, accurate identification is not merely a necessity; it is a cornerstone of scientific integrity."
With a focus on evolving practices and the role of technology, further analysis will explore the detailed methodologies employed in chemical identification.
Preface to Chemical Identification
Chemical identification plays an essential role in both scientific research and industrial applications. Understanding the identity and composition of chemical substances allows for accuracy in experimentation, regulatory compliance, and product development. In an era where chemicals are ubiquitousβfrom pharmaceuticals to environmental samplesβensuring correct identification underpins many facets of safety and effectiveness in a variety of sectors.
Definition and Importance
Chemical identification can be defined as the process of determining what chemical substances are present in a sample and understanding their characteristics. This process is crucial for many reasons:
- Safety: Accurate identification ensures proper handling and use of chemicals, mitigating risks of accidents and hazardous exposure.
- Regulatory Compliance: Many industries are bound by strict regulations that require precise chemical identification for compliance, particularly in the pharmaceutical and chemical manufacturing sectors.
- Quality Control: In production settings, identifying chemicals assists in maintaining the quality and consistency of products. It ensures that the correct substances are used for intended applications.
- Research Integrity: In laboratories, the inability to correctly identify a substance can lead to erroneous conclusions, affecting the overall integrity of scientific work.
Historical Context
The history of chemical identification stretches back several centuries. Early methods relied on observable physical and chemical properties. The advent of more advanced methods, like spectroscopy in the 19th century, greatly expanded the capabilities for identifying chemicals. Over time, mechanistic understanding alongside technological developments has shaped methods of identification today, moving from qualitative observations to sophisticated quantitative techniques. The interplay between growing knowledge and innovative technologies has continually refined the practice, allowing for more accurate and efficient identification of compounds.
In modern applications, the complexity of mixtures and the emergence of new substances present challenges that require continual adaptation in identification techniques. Recognizing this evolution facilitates a deeper appreciation for the intricate methodologies used today.
Principles of Chemical Identification
Chemical identification is rooted in established principles that ensure the accurate recognition and characterization of chemical substances. The significance of these principles cannot be overstated, as they form the backbone of methodologies used across various scientific disciplines. A thorough understanding of these underlying concepts is crucial for researchers looking to apply chemical identification techniques successfully. This section will explore fundamental concepts, as well as the role and importance of chemical nomenclature in chemical identification.
Fundamental Concepts
At the core of chemical identification are fundamental concepts that involve the properties and behaviors of substances. These principles guide everything from basic laboratory practices to advanced analytical techniques.
- Molecular Structure: Understanding a molecule's structure is essential for identifying compounds. Different arrangements of atoms result in different chemical properties.
- Chemical Properties: Each substance has unique chemical properties. These include melting and boiling points, solubility, reactivity, and others which help in identifying chemicals.
- Physical States: Recognizing whether a substance is solid, liquid, or gas provides immediate insight into its identity. For example, water exists as ice at lower temperatures and as vapor at higher ones.
- Functional Groups: Functional groups are specific groups of atoms that determine chemical behavior. Identifying these can significantly narrow down a substance's identity.
- Spectral Data: Various techniques such as infrared spectroscopy and mass spectrometry provide spectral data that can identify substances based on their interaction with different forms of energy.
These concepts form a framework for chemical analysis, ensuring that identification processes are systematic and reliable. Each of these areas interlinks to provide comprehensive data about a chemical, which is critical in both academic and industrial settings.
Chemical Nomenclature
Chemical nomenclature refers to the system of naming chemical compounds. It offers a standardized method for communicating chemical identities. Understanding nomenclature is essential for anyone working with chemicals, as it clarifies what substances are being referenced. Here are key aspects:
- Systematic Naming: This follows specific rules set by the International Union of Pure and Applied Chemistry (IUPAC). For example, ethanol is systematically named based on its structure and functional groups.
- Common Names: While less formal than systematic names, common names are widely used in many industries. For instance, acetic acid is commonly known as vinegar.
- Abbreviations and Symbols: In technical documentation, chemical symbols and abbreviations are frequently employed to save space and time. For example, NaCl represents sodium chloride.
- Context Matters: The choice of nomenclature may depend on the field of study. In pharmaceuticals, a compound may be referenced differently compared to materials science.
Nomenclature is not just a matter of names; it is integral for clear communication across scientific disciplines.
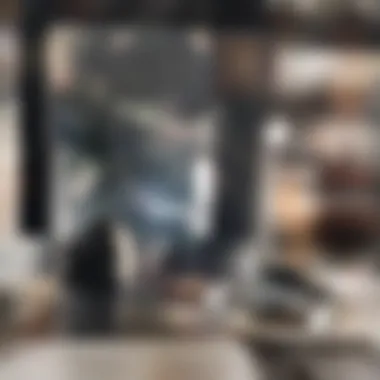
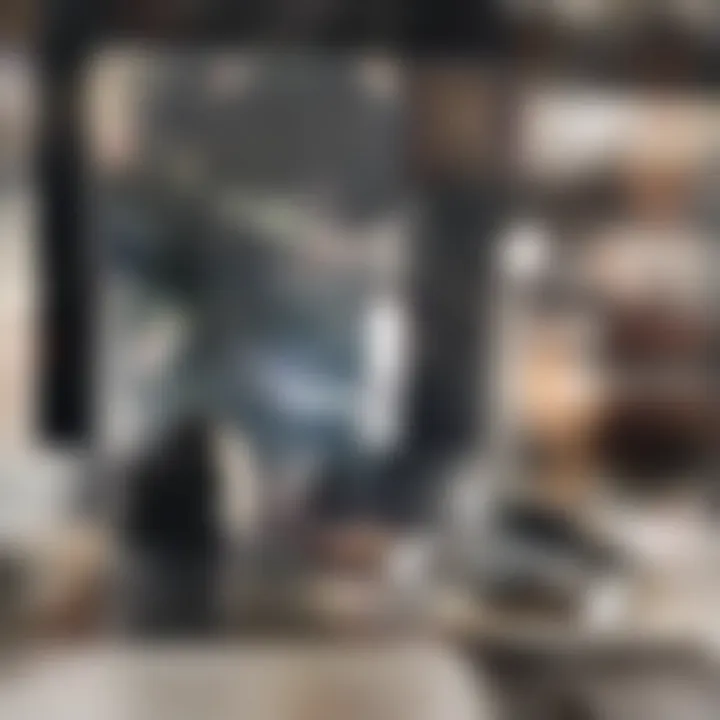
Methods of Chemical Identification
The methods of chemical identification are fundamental to various scientific fields, serving as the backbone for recognizing and analyzing substances. These methods encompass a range of techniques that allow scientists and industry professionals to precisely determine the identity of chemical compounds. Employing a diverse array of methods ensures reliability, accuracy, and repeatability, which are crucial in both research and industrial applications.
Spectroscopic Techniques
Spectroscopic techniques are paramount in identifying chemicals due to their ability to provide detailed information about molecular structures and functional groups. By measuring the interactions between light and matter, these methods offer insights that are essential for characterizing substances.
Infrared Spectroscopy
Infrared spectroscopy is a vital tool in chemical identification. It functions by measuring the absorption of infrared light by a sample, which causes molecular vibrations. This produces a spectrum unique to each compound, allowing scientists to determine functional groups present in the molecule. One of the key characteristics of infrared spectroscopy is its speed; it can provide results in minutes. This makes it a popular choice in many laboratories.
However, infrared spectroscopy has limitations. It is most effective for organic compounds and may not provide clear results for inorganic materials. Despite these drawbacks, its ability to rapidly identify functional groups is valuable in pharmaceutical research and environmental monitoring.
Nuclear Magnetic Resonance
Nuclear Magnetic Resonance (NMR) spectroscopy is another crucial method used for chemical identification. It works by applying a magnetic field to a sample, causing nuclei of atoms to resonate. This technique offers detailed structural information, making it a powerful tool, especially in organic chemistry. NMR is particularly beneficial due to its non-destructive nature, allowing for analysis without altering the sample.
Nonetheless, NMR requires sophisticated equipment and can be more time-consuming compared to infrared spectroscopy. Its high-resolution data is preferred in research settings where detailed structural elucidation is necessary.
Mass Spectrometry
Mass spectrometry (MS) is a highly sensitive and specific technique used for identifying chemicals. It operates by ionizing chemical compounds and measuring the mass-to-charge ratios of the ions produced. One significant aspect of mass spectrometry is its ability to detect trace levels of substances, which is critical for applications such as toxicology and forensic analysis.
This method offers exceptional accuracy and can determine the molecular weight of compounds. However, mass spectrometry can be complex and requires skilled interpretation of the data. Despite this, its power in identifying complex mixtures cannot be underestimated.
Chromatographic Methods
Chromatographic methods are instrumental in separating and identifying chemical compounds based on their interactions with stationary and mobile phases. These techniques are invaluable for analyzing complex mixtures in various fields, especially in pharmaceuticals and environmental analysis.
Gas Chromatography
Gas chromatography (GC) is widely used in chemical analysis for its efficiency and speed in separating volatile compounds. This method relies on the volatility of substances to separate them as they pass through a column. The key characteristic of GC is its ability to produce highly reproducible results with high resolution.
One unique feature of gas chromatography is its compatibility with mass spectrometry. When coupled with mass spectrometry, it offers powerful identification capabilities. However, GC may not be suitable for non-volatile compounds, limiting its applicability in certain scenarios.
High-Performance Liquid Chromatography
High-Performance Liquid Chromatography (HPLC) is another essential chromatographic method. Unlike gas chromatography, HPLC can handle a broader range of compounds, including non-volatile and thermally sensitive materials. It works by passing a liquid sample through a column filled with a stationary phase, separating compounds based on their interactions.
HPLC is especially beneficial in the pharmaceutical industry for purity testing and quality control. Its high sensitivity allows for the identification of components in complex mixtures. However, its operational costs and the need for specialized equipment can be barriers for some laboratories.
Electrochemical Methods
Electrochemical methods play a crucial role in chemical identification, especially for redox-active compounds. These techniques are based on measuring electrical properties, such as current or potential, to identify substances. Their sensitivity and the ability to work in challenging environments make them particularly valuable in fields such as environmental monitoring.
Electrochemical methods can analyze samples with minimal preparation, which is advantageous in many practical applications. Nonetheless, their specificity may vary depending on the nature of the target analytes and the presence of interfering substances.
Qualitative versus Quantitative Identification
Chemical identification can broadly be classified into two categories: qualitative and quantitative. Each of these approaches serves different purposes in research and industry, and understanding their distinct roles is essential for accurate chemical analysis. The choice between qualitative and quantitative methods often hinges on the specific objectives of an investigation or application.
Differences in Approaches
Qualitative identification focuses primarily on determining the presence or absence of a chemical substance. This approach does not provide information about the amount of the substance; instead, it relies on various techniques to confirm what substances are present in a sample. Techniques such as infrared spectroscopy or mass spectrometry can help highlight the unique characteristics of chemical compounds, enabling researchers to establish their identities.
In contrast, quantitative identification offers valuable insight into the concentration or amount of a chemical substance within a particular sample. This method is crucial in many fields such as pharmaceuticals, where precise dosage is paramount. Analytical techniques like gas chromatography or high-performance liquid chromatography can measure the quantity of compounds, providing comprehensive data on their concentrations. The distinction between these two types of identification is essential for scientists aiming to achieve specific results.
"In analytical chemistry, choosing between qualitative and quantitative methods greatly influences the outcomes of research and commercial analysis."
Applications in Research and Industry
Both qualitative and quantitative techniques play vital roles in various sectors. In the pharmaceutical industry, qualitative methods help identify active ingredients in drug formulas. At the same time, quantitative methods ensure that the dosage of these ingredients meets required safety and efficacy standards.
The environmental sciences also benefit from both approaches. Qualitative methods can detect contaminants in soil or water, indicating the presence of hazardous substances. On the other hand, quantitative methods help assess the levels of pollutants, providing data needed for regulatory compliance and environmental protection measures.
In materials science, qualitative identification allows for understanding the composition of materials, but quantitative analysis is essential for producing materials with precise specifications. Accurate measurements of composition ensure that materials meet performance characteristics required by applications, such as construction or electronics.
In summary, distinguishing between qualitative and quantitative identification is foundational for effective chemical analysis. Both approaches provide significant benefits tailored to the needs of various fields. Understanding these differences helps guide researchers and professionals in selecting appropriate methods for their specific goals.
Applications of Chemical Identification
Chemical identification serves as a cornerstone in many fields, providing essential data that informs decision-making and research initiatives. Its applications are vast and varied, impacting multiple industries and areas of study. Recognizing the chemical composition of substances allows for improved safety, regulatory compliance, and product development. This section delves into distinct applications in the pharmaceutical industry, environmental analysis, and materials science, highlighting their relevance and importance.
Pharmaceutical Industry
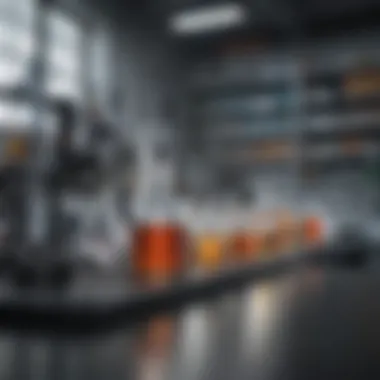
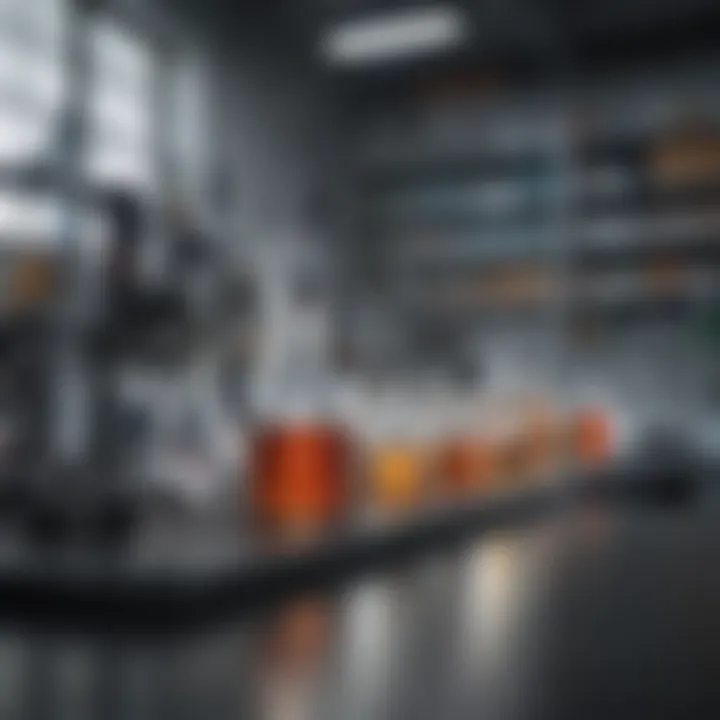
In the pharmaceutical sector, the precise identification of chemicals is critical. Drug development relies heavily on the ability to characterize compounds accurately. This includes identifying the active pharmaceutical ingredients (APIs) and ensuring their purity. Techniques like mass spectrometry and nuclear magnetic resonance are frequently utilized for these purposes.
Accurate chemical identification is crucial for ensuring patients receive the correct dosage and formulation of medications.
Besides quality assurance, regulatory bodies such as the FDA require detailed chemical profiles for drug approval. In this context, methods such as high-performance liquid chromatography (HPLC) provide the necessary data to fulfill these standards.
Furthermore, in pharmacovigilance, identifying chemicals related to adverse drug reactions helps in assessing safety and efficacy. This understanding assists in mitigating risks associated with pharmaceutical products, ultimately leading to safer healthcare outcomes.
Environmental Analysis
Environmental sciences rely on chemical identification to monitor and assess pollution levels. Various techniques help detect harmful substances in air, water, and soil. Chromatographic methods, for example, are essential for isolating pollutants from complex mixtures, thus allowing for accurate quantification.
Identifying persistent organic pollutants (POPs) or heavy metals within ecosystem samples is pivotal. It aids in understanding their long-term impact on health and biodiversity.
Moreover, advancements in analytical methods, such as Infrared Spectroscopy, enable researchers to identify chemical compounds rapidly in the field, thus enhancing response times for environmental hazards. Regulatory agencies depend on consistent chemical identification for environmental assessments and compliance reporting. It influences policymaking and sustainability efforts significantly.
Materials Science
In materials science, the identification of chemical composition directly impacts the properties and performance of materials. This includes not only metals and polymers but also nanomaterials and composites. Understanding chemical identity leads to innovative approaches in material design.
Techniques like X-ray diffraction and scanning electron microscopy permit detailed analysis of materials at the micro and nanoscale. These insights are vital for developing new materials with tailored properties.
The role of chemical identification is not just academic but is fundamentally tied to industry. Manufacturers utilize this information to ensure product durability, functionality, and safety. It assists in the quality control processes within various manufacturing environments, ultimately impacting economic factors like cost and efficiency.
In summary, the applications of chemical identification are integral across different sectors. They determine how drugs are developed, how environment protections are enforced, and how materials are innovated. Each field mirrors the others in its reliance on precise and accurate chemical identification to progress and achieve both practical and theoretical advancements.
Challenges in Chemical Identification
In the realm of chemical identification, practitioners face a multitude of challenges that can complicate the accurate recognition of substances. These challenges arise from the inherent complexity of chemical mixtures and the potential for contamination, both of which require nuanced understanding and advanced techniques for effective resolution. Navigating these obstacles is essential for ensuring the reliability and accuracy of chemical analysis across various fields.
Complex Mixtures
Complex mixtures present a significant challenge in the identification process. Many substances exist not in isolation but as part of intricate mixtures, often involving numerous components at varying concentrations. When attempting to identify chemicals within such mixtures, it can be difficult to discern individual components, especially if they exhibit overlapping spectral features or similar chemical properties.
Consider, for example, the analysis of environmental samples, such as soil or water, where pollutants may exist alongside naturally occurring substances. In these cases, the identification methods must be sensitive enough to distinguish trace amounts of harmful chemicals from non-toxic alternatives.
To effectively analyze complex mixtures, scientists often rely on advanced methodologies, such as:
- Multi-dimensional chromatography: This allows for separation of compounds based on multiple characteristics.
- Sophisticated spectroscopic techniques: Utilization of instruments that can detect subtle differences in chemical structure.
Such methods, however, often come with increased costs and require specialized training, making them less accessible in some contexts.
Interference and Contamination Issues
Interference from other substances can severely compromise the accuracy of chemical identification. Various environmental factors or substances present in the samples can obstruct the clear identification of target chemicals. For instance, matrix effects can occur when the presence of other components affects the response of the analytical method, leading to misinterpretation of results.
Contamination is another prevalent issue that complicates the identification process. Samples can become tainted from various sources, such as improper handling or the materials used in analysis. This can lead to erroneous conclusions about the substances present.
To mitigate these challenges, laboratories implement several practices, including:
- Strict protocols for sample collection and handling: Ensuring that external factors do not introduce contaminants.
- Routine calibration and validation of instruments: Maintaining accuracy in measurement.
Furthermore, ongoing training for personnel in best practices is crucial to minimize human error.
"Understanding the challenges in chemical identification is necessary for developing solutions that enhance accuracy and reliability."
In summary, recognizing the challenges posed by complex mixtures and interference issues is key to improving chemical identification processes. By addressing these obstacles through advanced techniques and stringent protocols, researchers can enhance the accuracy of their findings and ensure more reliable results.
Emerging Technologies in Chemical Identification
Emerging technologies are reshaping the landscape of chemical identification, bringing forth innovative methods that enhance accuracy and efficiency. The integration of cutting-edge tools and techniques is vital for streamlining processes, making them more reliable in areas such as pharmaceuticals, environmental monitoring, and materials science. As industry demands peak for rapid and detailed chemical analysis, the role of these technologies cannot be overstated.
Artificial Intelligence and Machine Learning
Artificial intelligence (AI) and machine learning (ML) are revolutionizing chemical identification by allowing systems to learn from data, improving prediction capabilities and analytical performance over time. AI algorithms can sift through large datasets to identify patterns and trends that may not be readily apparent to human analysts.
Benefits of AI and ML in chemical identification include:
- Automated analysis: Reduces the time needed for identifying chemical compounds.
- Enhanced accuracy: Reduces human error through algorithmic evaluations and pattern recognition.
- Predictive modeling: AI can forecast chemical behaviors, helping in preemptive identification.
However, the integration of AI into chemical identification presents challenges. Dependence on quality data is crucial; poor data leads to inaccurate results. Additionally, there is an ongoing debate regarding the transparency of AI decisions in complex chemical analyses.
Advancements in Instrumentation
The rapid advancement in instrumentation technology is another significant facet of emerging strategies in chemical identification. Instruments such as high-resolution mass spectrometers and state-of-the-art chromatographic systems are increasingly used. These tools offer enhanced sensitivity and specificity, facilitating the detection of trace amounts of substances with precision.
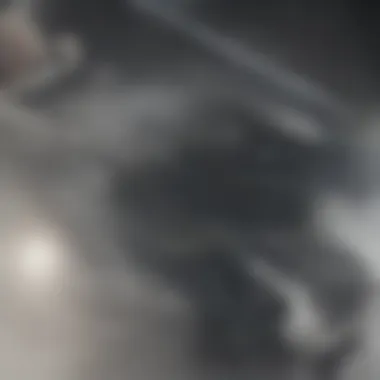
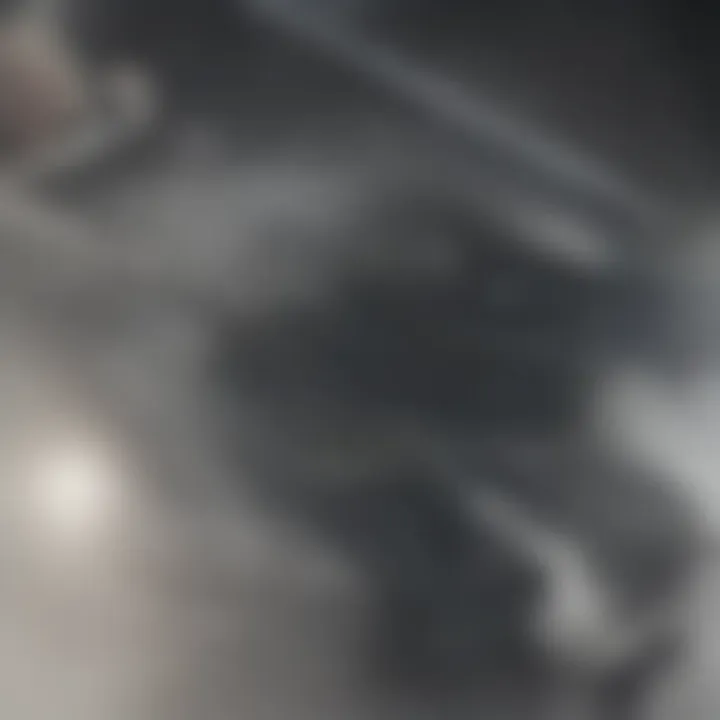
Current advancements include:
- Miniaturization: Compact devices enable on-site analysis, eliminating delays in testing processes.
- Real-time data acquisition: This enhances the speed at which results are generated, crucial for time-sensitive applications, such as detecting substances in environmental samples after a disaster.
Moreover, advancements in data processing capabilities allow for complex chemical analyses that were previously unfeasible. The combination of intricate instrumentation and sophisticated software provides a robust framework for chemical identification today.
"The evolution of technology continuously challenges scientists to adapt and innovate in chemical identification methodologies."
In summary, the development of emerging technologies, particularly in AI and instrumentation, is essential for modern chemical identification. Researchers must navigate the balance between harnessing these advancements and addressing the accompanying challenges. The future of chemical identification is undoubtedly intertwined with these technologies, promising enhanced capabilities and insights.
Regulatory Considerations
Regulatory considerations are essential in chemical identification as they ensure the safe use and handling of chemical substances across various industries. Understanding and implementing these regulations is vital for compliance, consumer safety, and environmental protection. Regulatory frameworks guide researchers and professionals in adhering to specific laws, standards, and best practices, which ultimately mitigate risks associated with the use of hazardous chemicals.
Safety Data Sheets
Safety Data Sheets (SDS) serve as a critical component in the regulatory landscape for chemical identification. An SDS provides detailed information about a chemical substance, including its properties, hazards, handling instructions, and emergency response measures. It must be accessible to all individuals who might come into contact with the substance, ensuring that adequate precautions are taken.
The benefits of having an effective SDS include:
- Risk Management: SDS help in identifying potential hazards associated with chemicals, allowing users to implement proper safety measures.
- Regulatory Compliance: Properly maintained SDS ensure compliance with regulations such as OSHA and REACH, critical for legal operations within many sectors.
- Training Tool: SDS is often used in training programs to educate employees about safe chemical handling procedures.
In many jurisdictions, compliance with SDS standards is not just recommended but mandated. This highlights the need for manufacturers and suppliers to provide accurate or up-to-date information continually.
Compliance Standards
Compliance standards refer to the established guidelines that govern the production, distribution, and use of chemical substances. These standards are set by national and international organizations to protect health, safety, and the environment. Familiarity with these standards is essential for any professional involved in the chemical identification process.
The significance of compliance standards can be outlined in several key areas:
- Uniformity: Standards provide a consistent framework for classifying and labeling chemicals, which helps in reducing confusion and promoting safety.
- Quality Assurance: Adhering to standards ensures the quality and reliability of chemical identification methods, which is vital for research and industrial applications.
- Global Trade: International compliance standards facilitate cross-border trade, ensuring that chemicals are safely handled and recognized in different markets.
Professionals must stay informed about changes in regulations to ensure ongoing compliance. Regular updates and periodic reviews of established standards are crucial, since new scientific developments may influence safety and regulatory measures.
"Understanding regulatory considerations is not only about compliance; it is about fostering a culture of safety and accountability in chemical practices."
Overall, regulatory considerations in chemical identification are multifaceted. They cover not only the practical aspects of safety and compliance but also echo broader implications for health, environmental protections, and ethical responsibilities in scientific endeavors.
Future Trends in Chemical Identification
Future trends in chemical identification are significant for advancing methodologies in various scientific fields. Understanding these trends enables researchers and professionals to stay ahead in their discipline, ensuring efficiency and accuracy in chemical identification processes. These trends revolve around two key components: the integration of digital technologies and the push for sustainable practices in chemical analysis.
Integration with Digital Technologies
The integration of digital technologies into chemical identification techniques represents a transformative shift in how compounds are analyzed. Advanced software and algorithms now assist in data interpretation, offering enhanced precision and speed. Such systems utilize machine learning to improve the identification process significantly.
Key elements of this integration include:
- Chemoinformatics: This blends chemistry and computation, utilizing databases and software tools. It provides valuable insights into chemical structures and properties without extensive laboratory work.
- Automated Systems: Automation enhances the throughput of chemical analysis, allowing for high-volume screening of substances. This is particularly beneficial in industries such as pharmaceuticals, where rapid identification can accelerate drug development.
- Real-Time Data Analysis: New tools enable real-time monitoring and analysis of samples, thus facilitating immediate decision-making. This is especially critical in environmental monitoring, where timely interventions can prevent ecological damage.
These advancements not only improve efficiency but also elevate the accuracy of chemical identification, which can lead to safer practices and innovations in various applications.
"The future of chemical identification lies in the merging of chemistry with digital technologies, catalyzing breakthroughs in efficiency and accuracy."
Sustainable Practices in Chemical Analysis
Sustainability in chemical analysis is gaining traction, driven by an increasing awareness of environmental impacts. Embracing sustainable practices is no longer optional; it is essential for future research and industrial operations. These practices focus on minimizing waste, using eco-friendly solvents, and implementing green chemistry principles.
Some beneficial aspects of sustainable practices include:
- Reduction of Hazardous Waste: By selecting greener reagents and methods, laboratories can significantly reduce their chemical waste footprint.
- Energy Efficiency: New methodologies aim to consume less energy while providing the same or improved results. This aligns with global sustainability initiatives aimed at lowering emissions and conserving energy.
- Lifecycle Analysis: Comprehensive studies on the full lifecycle of chemical processes help identify opportunities for sustainability. This includes everything from raw material sourcing to disposal of chemical byproducts.
Epilogue
Summary of Key Points
Chemical identification employs diverse methodologies ranging from classic techniques, such as spectroscopic and chromatographic methods, to modern advances like artificial intelligence and machine learning. This comprehensive examination highlights how these methodologies are applied in various sectors:
- Pharmaceutical Industry: Chemical identification ensures drug safety and efficacy by accurately determining chemical composition.
- Environmental Analysis: Accurate chemical identification aids in monitoring pollutants and ensuring compliance with environmental standards.
- Materials Science: In studying materials properties, precise identification of components is crucial for developing new technologies.
Each of these points illustrates how essential chemical identification is to advancing knowledge and practice within science and industry alike.
Implications for Future Research
Looking ahead, the realm of chemical identification is positioned for growth. Future research should focus on enhancing the accuracy and efficiency of identification techniques through
- Integration with Digital Technologies: Leveraging advances in technology can streamline processes, making identification more accessible and faster.
- Sustainable Practices: Emphasizing eco-friendly methods in chemical analysis could pave the way for less harmful practices and materials.
Investing in these developments may not only enrich scientific discovery but also ensure that industries adapt to modern challenges effectively.
To sustain advancements in this field, continuous collaboration among researchers, educational institutions, and industries is imperative. By fostering such connections, the impact of chemical identification can be maximized, thereby improving safety, compliance, and innovation across various disciplines.