Exploring Chemiluminescent Enzyme Immunoassays
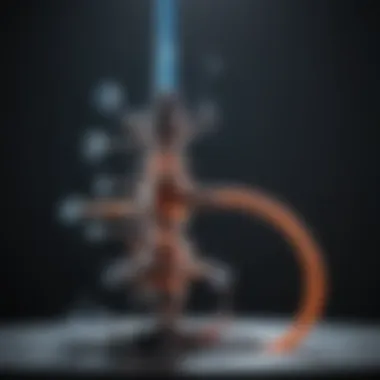
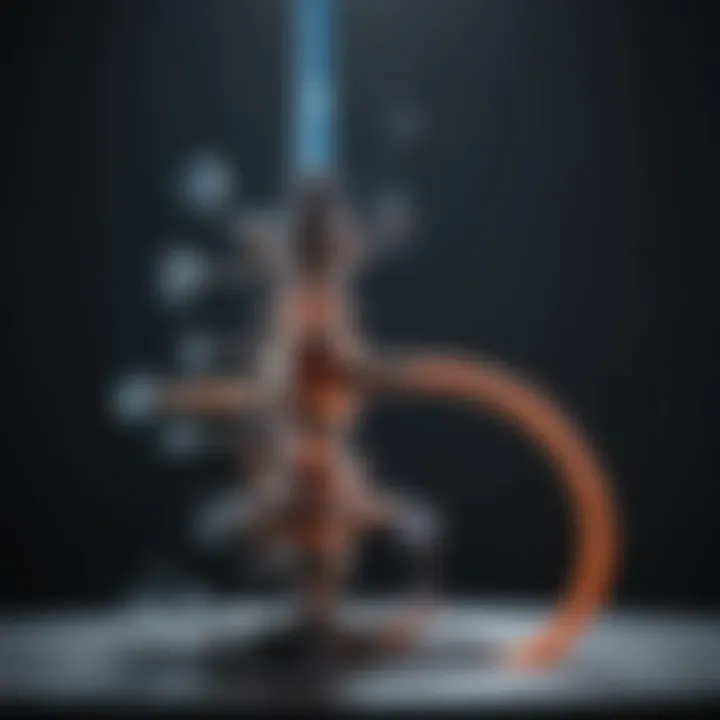
Intro
Chemiluminescent enzyme immunoassays (CLEIA) represent an innovative approach to biochemical analysis. The technique leverages the phenomenon of chemiluminescence to detect and quantify specific antigens or antibodies in a biological sample. By employing enzymes that catalyze reactions resulting in light emission, CLEIA provides an effective and sensitive method for diagnostics and environmental testing. The significance of this methodology lies in its versatility and enhancement over traditional techniques.
Understanding CLEIA requires not only familiarity with its mechanisms but also an awareness of its practical applications across multiple fields. As researchers and professionals in clinical diagnostics, environmental monitoring, and other areas continue to seek precise measurement tools, the relevance of CLEIA cannot be overstated. This article is structured to provide a thorough analysis of the principles, applications, and recent advancements within the landscape of chemiluminescent enzyme immunoassays.
Research Overview
Key Findings
- Precision and Sensitivity: CLEIA demonstrates high sensitivity, allowing for detection of low-abundance biomarkers that may be missed by conventional assays.
- Diverse Applications: Applications of CLEIA range from medical diagnostics to environmental testing, showcasing its adaptability.
- Technological Advancements: Recent developments have led to enhanced performance and reduced assay times, making CLEIA a robust choice for modern laboratories.
Study Methodology
The examination of CLEIA is based on a mix of literature review and case studies. The approach includes analyzing various published research articles and field reports to establish a comprehensive understanding. Methods employed in these studies typically involve comparative analysis with other assay techniques.
Background and Context
Historical Background
Chemiluminescent techniques have evolved since their inception, with immunoassays gaining popularity in the late 20th century. Early approaches relied heavily on radioimmunoassay methods, which have limitations in terms of safety and complexity. As researchers sought alternatives, the coupling of chemiluminescent reactions with enzyme-based technology marked a significant breakthrough in the field. This evolution highlights the transition toward safer and more user-friendly diagnostic tools.
Current Trends in the Field
Currently, the field of CLEIA is witnessing several trends:
- Integration with Automation: Many laboratories are adopting automated systems to enhance throughput and reduce human error.
- Miniaturization of Assays: Development of microfluidic devices presents opportunities for smaller sample volumes while maintaining sensitivity.
- Use of Nanoparticles: Incorporating nanoparticles increases surface area and enhances signal output, which can improve test performance.
Insights into these trends point to a future where CLEIA remains central to innovations in diagnostics. The growing demand for precise and rapid laboratory tests confirms the enduring relevance of this technique.
Intro to Chemiluminescent Enzyme Immunoassays
Chemiluminescent enzyme immunoassays (CLEIA) represent a significant advancement in the field of analytical biochemistry. This technique integrates the specificity of immunological assays with the sensitivity of chemiluminescent detection, making it applicable in diverse areas, from clinical diagnostics to environmental assessments. As public health concerns rise and the demand for rapid testing increases, understanding CLEIA becomes vital.
Definition and Overview
CLEIA is a method where an enzyme catalyzes a reaction that produces light, used to detect and quantify specific substances in a sample. The enzymatic reaction generates luminescence that can be measured, offering high sensitivity and specificity through tightly controlled conditions. CLEIA generally utilizes antibodies that bind to the target analyte, thus providing a measurable signal correlating to the concentration of that analyte in the sample.
The fundamental principle behind CLEIA involves a chemiluminescent substrate and an enzyme, most commonly alkaline phosphatase or horseradish peroxidase. When these two are combined in the presence of the target of interest, a measurable light signal is emitted. This property allows for the detection of very low levels of analytes, making CLEIA a preferred choice in many scientific fields.
Historical Development
The history of chemiluminescence dates back to discoveries in the early 19th century. However, the specific application of these principles to enzyme immunoassays emerged during the 1970s. With the introduction of enzyme-linked immunosorbent assays (ELISA), researchers began exploring alternatives that offered enhanced detection capabilities.
In the years that followed, the combination of chemiluminescent detection with enzyme immunoassays gained momentum. Early studies demonstrated the advantages of this hybrid approach, particularly in improving the sensitivity of immunoassays. By the late 1990s, CLEIA began receiving recognition as a powerful tool in immunodiagnostics. Further research and innovations led to the development of various substrates and detection systems, dramatically expanding its applications in clinical, environmental, and research settings. Today, CLEIA stands as a valuable instrument in addressing complex challenges in health and safety.
Fundamentals of Chemiluminescence
Understanding the fundamentals of chemiluminescence is essential for grasping its application within chemiluminescent enzyme immunoassays (CLEIA). Chemiluminescence involves the emission of light as a result of a chemical reaction. This property is widely explored in various scientific disciplines due to its ability to provide sensitive and specific detection capabilities. Knowing the different types of chemiluminescence and their mechanisms enables scientists to utilize this powerful technique effectively in diagnostics and environmental monitoring. Thus, the significance of this section lies in establishing a solid foundation that underpins the concepts addressed throughout the article.
Chemical Reactions and Light Emission
Chemiluminescence occurs through diverse chemical reactions where energy is released in the form of light. In these reactions, the reactants, often in an excited state, release photons as they return to a stable ground state, thus emitting visible light. Understanding the kinetics of these reactions, including the factors that influence the emission intensity and duration, is critical for the optimization of assays like CLEIA. Moreover, specific substrates and enzymes can enhance sensitivity, making it crucial to consider the chemistry behind the light-emitting process.
Types of Chemiluminescence
Exploring the types of chemiluminescence reveals various methods that can be applied in CLEIA.
Bioluminescence
Bioluminescence refers to the light produced by living organisms through biochemical reactions, commonly observed in marine animals and some fungi. Its major contribution to immunoassays is its innate biological integration, allowing for the development of assays that mimic natural processes. A key characteristic of bioluminescence is its high specificity and sensitivity, making it an excellent choice for detection purposes. However, its dependency on biological components can also be a limitation, as environmental factors might influence the luminescent output, requiring careful control in practical applications.
Luminol-based Reactions
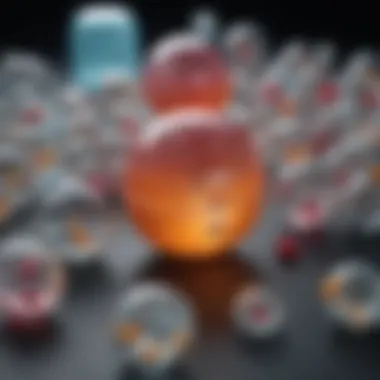
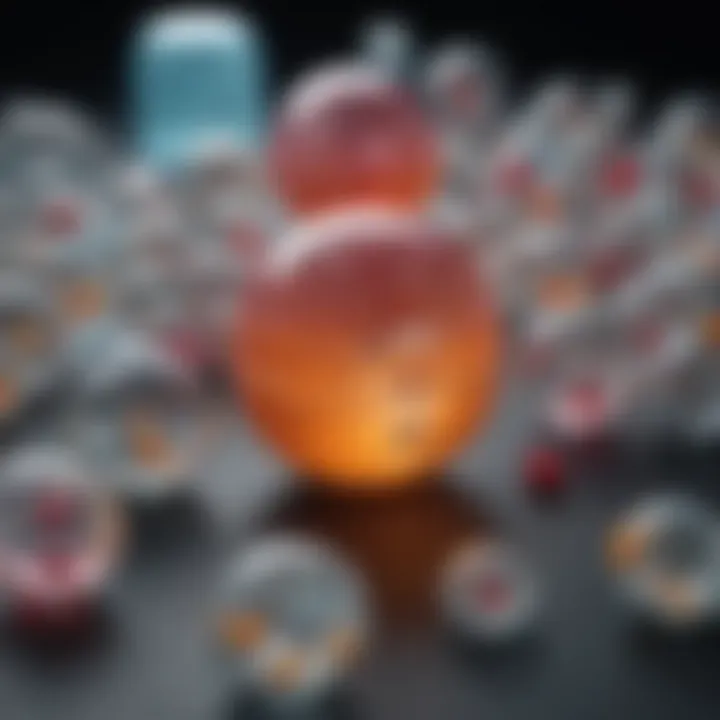
Luminol-based reactions involve the oxidation of luminol, producing a blue light visible during the decay of the excited state. This method is prevalent in various scientific assays due to its strong and consistent light emission, providing reliable detection of target molecules. One key characteristic of luminol is its simplicity and efficiency in reaction with peroxidase enzymes. Nonetheless, its sensitivity may be affected by the presence of inhibitors or competing substances, which can lead to variations in assay performance.
Flash Chemiluminescence
Flash chemiluminescence represents a rapid emission of light when substrates are introduced to a reaction medium, typically resulting in a short-lived yet intense burst of light. This type of chemiluminescence is notable for its instantaneous response and can significantly improve assay throughput. A unique feature is its adaptability to high-throughput screening formats, allowing for quick analysis of numerous samples. On the other hand, the transient nature of the light emission may necessitate precise timing in detection, adding complexity to the measurement process.
Mechanism of Chemiluminescent Enzyme Immunoassays
The mechanism of chemiluminescent enzyme immunoassays (CLEIA) is fundamental to understanding how these assays operate and their application in detecting various biological markers. This section elucidates the intricate processes that underlie CLEIA, emphasizing the roles of enzymes and substrate interactions. Understanding this mechanism is essential for researchers who wish to optimize assay conditions for enhanced sensitivity and specificity.
Role of Enzymes in CLEIA
Enzymes are vital components in chemiluminescent enzyme immunoassays. Within these assays, they act as catalysts that promote chemical reactions without being consumed in the process. Enzymes such as alkaline phosphatase and horseradish peroxidase are commonly used because of their high turnover numbers and ability to produce a substantial signal.
- Enzymatic Activity: The efficiency of the enzymatic reaction directly affects the overall sensitivity of the assay. A single enzyme molecule can convert many substrate molecules into products, thus amplifying the signal generated.
- Specificity: The choice of enzyme also influences the specificity of the assay. Different enzymes react with distinct substrates, and this selectivity is crucial for reducing background noise and increasing the integrity of the results.
- Optimization: Factors such as pH, temperature, and incubation time can significantly impact enzymatic activity. Careful optimization of these variables is necessary to achieve the desired performance of the assay.
Substrate Conversion and Signal Generation
The conversion of the substrate by the enzyme is critical for signal generation in CLEIA. This process begins when the enzyme encounters its specific substrate in the presence of a target analyte, usually an antibody or antigen.
- Binding: When the analyte binds to the antibody, it creates a complex that allows the enzyme to interact with the substrate.
- Catalysis: The enzyme then catalyzes the conversion of the substrate. During this step, a chemical reaction occurs that produces light as a byproduct. The intensity of this emitted light correlates with the amount of target analyte present in the sample.
- Measurement: The emitted light is measured using specialized detectors, providing quantitative data that allows for the determination of target concentration.
"The signal generated through substrate conversion is directly proportional to the analyte concentration, making system calibration critical for accurate results."
Types of Enzymes Commonly Used in CLEIA
The choice of enzyme in chemiluminescent enzyme immunoassays (CLEIA) is pivotal for the overall performance and effectiveness of the assay. Enzymes play a fundamental role, serving as the biocatalysts that facilitate the chemiluminescent reaction, leading to the generation of a measurable signal. In this section, we will explore two of the most commonly used enzymes in CLEIA: Alkaline Phosphatase and Horseradish Peroxidase. Understanding their properties, advantages, and application contexts allows researchers and practitioners to make informed decisions when designing and optimizing assays.
Alkaline Phosphatase
Alkaline Phosphatase (ALP) is widely used in CLEIA due to its stability and broad substrate range. ALP catalyzes the hydrolysis of phosphate esters in an alkaline environment and converts substrates into detectable products, often producing light as a byproduct.
Some important considerations when using Alkaline Phosphatase include:
- Substrate Compatibility: ALP can work with several substrates, like 4-Methylumbelliferyl Phosphate (MUP), which produces a fluorescent compound that can enhance detection sensitivity.
- Reaction Conditions: The enzyme operates effectively in a pH range of 8 to 10, making it optimal for alkaline reactions. This feature is integral when designing buffer systems.
- Signal Generation: The direct correlation between enzyme concentration and luminescence output is crucial. Higher enzyme activity leads to increased light output.
The benefits of using ALP extend to its reliability in producing consistent results. When used correctly, assays can achieve high specificity and sensitivity, making ALP a preferred choice in clinical diagnostics.
Horseradish Peroxidase
Horseradish Peroxidase (HRP) is another prominent enzyme used in CLEIA. It catalyzes oxidative reactions and can utilize a variety of substrates, most notably hydrogen peroxide.
Key characteristics and advantages of HRP include:
- Versatile Substrate Use: HRP can engage with substrates like luminol, producing measurable light during the catalytic process. This versatility expands its applications across multiple fields, from clinical to environmental areas.
- Enhanced Sensitivity: The signal amplification through HRP can often lead to lower detection limits, outperforming other assays. This enhancement is significant in detecting low-concentration biomolecules or contaminants.
- Operational Flexibility: HRP functions effectively in a range of conditions, which interprets to adaptable experimental designs.
According to numerous studies, HRP remains one of the most effective enzymes for detection in CLEIA, attributed to its ability to produce a robust signal even in complex biological samples.
"The choice of enzyme can greatly influence the sensitivity, specificity, and overall success of chemiluminescent assays."
Understanding the attributes of Alkaline Phosphatase and Horseradish Peroxidase equips researchers with essential insights for the development and implementation of effective CLEIA methodologies.
Design and Optimization of CLEIA
The design and optimization of chemiluminescent enzyme immunoassays (CLEIA) play a crucial role in ensuring the reliability and accuracy of outcomes. This section highlights integral elements that must be considered while developing these assays, along with the associated benefits these optimizations can provide. A well-designed CLEIA can effectively amplify signals, reduce background noise, and yield precise quantification of analytes.
Assay Components and Their Roles
Every assay consists of specific components that work synergistically to produce reliable results. Understanding each component's role offers insight into how to optimize the entire process.
- Antigen or Antibody: The choice of the primary or secondary antibody influences specificity. High-affinity antibodies are essential for minimizing cross-reactivity and enhancing the accuracy of the assay.
- Enzyme: Enzymes like alkaline phosphatase or horseradish peroxidase catalyze the reaction leading to light emission. Selecting the right enzyme based on its reaction kinetics is vital for maximizing signal sensitivity.
- Substrate: The substrate must be chosen carefully to ensure effective conversion by the enzyme and optimal signal output. The kinetic properties of substrates can impact the speed and intensity of chemiluminescence, influencing overall result quality.
- Buffer Solutions: Buffers help to maintain pH stability and ionic strength, which can affect the reaction efficiency and the stability of the components. Careful buffer selection correlates directly with assay performance.
Optimizing these components improves not only the sensitivity but also the reliability of the detection method. It is also necessary to focus on interactions between components. A good understanding can lead to improved assay designs and reduced variables.
Calibration and Sensitivity Enhancements
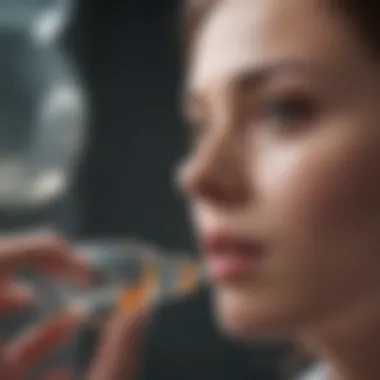
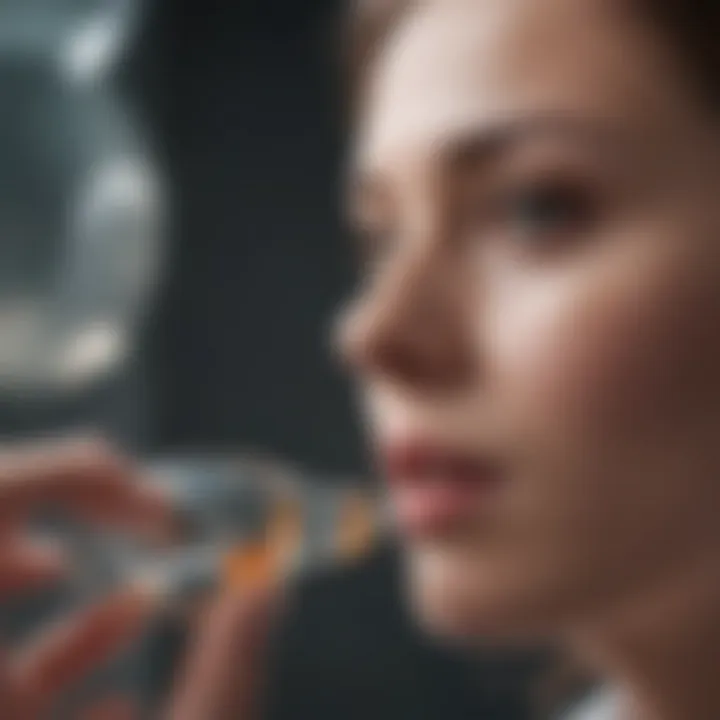
Calibration is essential in ensuring accurate and reproducible measurements in clinical and environmental settings. Proper calibration techniques provide a framework for quantifying analytes with high precision. Here are strategies for enhancing calibration and sensitivity in CLEIA:
- Standardization of Procedures: Establishing a protocol with consistent assay conditions leads to improved reproducibility. This includes standardizing times, temperatures, and incubation periods.
- Use of Calibration Curves: Generating calibration curves with known concentrations allows for accurate quantification of unknown samples. By assessing a range of concentrations, a more refined curve can be created, thus improving the use of assay results.
- Dilution Factors: Adjusting the concentrations of the assay components can help to fine-tune sensitivity. Initial tests may require a variety of dilutions to find the optimal balance for accurate detection.
- Advanced Signal Detection Techniques: Implementing advanced detection technologies, such as photomultiplier tubes or optimized imaging systems, enhances the ability to detect low signals. Investments in better detection equipment may increase the sensitivity range significantly.
- Environmental Controls: Monitoring conditions such as temperature, light, and humidity can impact performance. Implementing controls to minimize variability in these factors aids reproducibility and sensitivity.
"Optimizing CLEIA design and carefully calibrating assays can translate to significant advancements in diagnostic capabilities."
In summary, the design and optimization of CLEIA not only enhance assay performance but also strengthen the validity of findings in various applications. Each component's careful selection combined with meticulous calibration techniques ensures chemiluminescent assays maintain their value in both research and clinical diagnostics.
Applications of Chemiluminescent Enzyme Immunoassays
The applications of chemiluminescent enzyme immunoassays (CLEIA) are numerous and diverse, encompassing various fields such as clinical diagnostics and environmental monitoring. Understanding these applications is crucial, as they illustrate how CLEIA contributes to both research and practical healthcare solutions. This section will explore the specific advantages of CLEIA in different contexts, emphasizing its effectiveness in diagnosing diseases and monitoring environmental health.
Clinical Diagnostics
Detection of Infectious Diseases
Detection of infectious diseases through CLEIA is one of the most significant applications in clinical diagnostics. The method allows for rapid and sensitive detection of pathogens, which is vital in controlling outbreaks. The key characteristic of this application is its ability to identify specific antigens or antibodies related to infectious agents, providing accurate results quickly. This speed is crucial in clinical settings where timely information can affect treatment decisions.
Moreover, CLEIA's high sensitivity allows it to detect low concentrations of pathogens, which is often necessary in early disease stages. A unique feature of this detection method is its adaptability, allowing it to be applied to various infectious agents, including viruses, bacteria, and fungi. However, it is essential to consider possible false positives due to cross-reactivity, which may lead to unnecessary treatments or anxiety for patients, demanding thorough validation.
Monitoring Hormones and Biomarkers
Monitoring hormones and biomarkers is another vital application of CLEIA. This area is particularly beneficial for diagnosing hormonal imbalances and various health conditions such as diabetes and thyroid dysfunction. A notable aspect of monitoring hormones is its ability to provide quantitative measurements, which facilitate the assessment of an individualโs health status over time.
The primary advantage of using CLEIA in this context is its specificity and sensitivity, enabling the detection of hormones at very low levels. This enables clinicians to monitor subtle changes that could be informative for managing patient care. Furthermore, CLEIA can measure multiple biomarkers simultaneously, enhancing its efficiency in clinical labs. Despite these benefits, the potential for assay interference from other substances presents a challenge that must be carefully controlled to ensure accuracy.
Environmental Monitoring
Assessment of Contaminants
In the realm of environmental monitoring, the assessment of contaminants using CLEIA is significant. This application allows for the detection of various chemicals and biological agents in water, soil, and air samples. The key advantage is the method's high sensitivity, which makes it possible to identify harmful levels of contaminants that could affect public health or ecosystems.
This type of monitoring is particularly beneficial for regulatory compliance and safety assessments. The unique feature of CLEIA in environmental contexts is its rapid analysis capability, allowing for real-time monitoring of contaminants. However, a challenge faced in this area includes the complexity of environmental samples, which may contain substances that interfere with assay results and require sophisticated sample preparation.
Detection of Pathogens
The detection of pathogens in environmental samples is increasingly crucial, especially in the context of food safety and water quality. This application leverages the same principles seen in clinical diagnostics, with the added complexity of environmental matrices. The ability of CLEIA to provide quick and specific results makes it a valuable tool for monitoring pathogenic organisms that pose health risks.
An important aspect of this application is its capability to test for multiple pathogens simultaneously. This offers a comprehensive view of a sample's safety and quality. However, ensuring the validity of results in such complex mixtures can be challenging and may require extensive method development and validation. Nevertheless, these challenges underline the importance of ongoing research and innovation in CLEIA technology.
Advantages of CLEIA Over Traditional Assays
Chemiluminescent enzyme immunoassays (CLEIA) present significant advancements over traditional assay methods. The unique properties of chemiluminescence, combined with enzymatic reactions, provide an effective framework for highly sensitive and specific detection of various analytes. Understanding the benefits of CLEIA is crucial for researchers, clinicians, and educators seeking efficient diagnostic tools.
Sensitivity and Specificity
One of the standout features of CLEIA is its enhanced sensitivity. Compared to traditional immunoassays, this method can detect lower concentrations of target substances. This increased sensitivity is due to the amplified signal that arises from the enzymatic conversion of substrates, generating a luminescent output that is measurable with high precision. For instance, assays that utilize alkaline phosphatase or horseradish peroxidase as enzymes can achieve detection limits in the picogram to femtogram range.
In terms of specificity, CLEIA benefits from the remarkable selectivity of antibodies used in these assays. High specificity reduces the chances of cross-reactivity, which can lead to false positives in traditional assays. This property is crucial in clinical diagnostics, especially in complex biological samples where numerous substances may interfere with the assay results. The tailored design of CLEIA allows it to identify specific biomarkers with confidence.
Speed and Efficiency
Speed is another major advantage of CLEIA. These assays can often be completed in a fraction of the time required for comparable traditional methods. The rapid reaction kinetics associated with chemiluminescent reactions can lead to quicker results, which is essential in settings such as emergency diagnostics or infectious disease detection.
Furthermore, CLEIA enables automation, significantly streamlining laboratory processes. Automated platforms can conduct multiple assays simultaneously, reducing labor time and minimizing human error. This efficiency not only improves throughput but also facilitates routine testing in clinical laboratories and research facilities.
"The fusion of speed and efficiency in CLEIA addresses the immediate needs of diagnostics, paving the way for better healthcare outcomes."
Challenges and Limitations of Chemiluminescent Assays
Chemiluminescent enzyme immunoassays (CLEIA) represent a significant advancement in analytical techniques. However, like any complex system, they do come with their own set of challenges and limitations. Understanding these aspects is crucial for researchers and practitioners who utilize these assays in clinical and environmental settings. Addressing the challenges can lead to improvements in method development, enhancing the reliability and accuracy of results.
Interference and False Positives
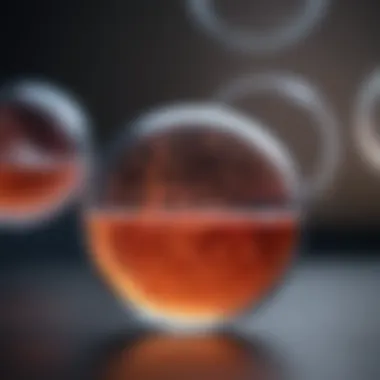
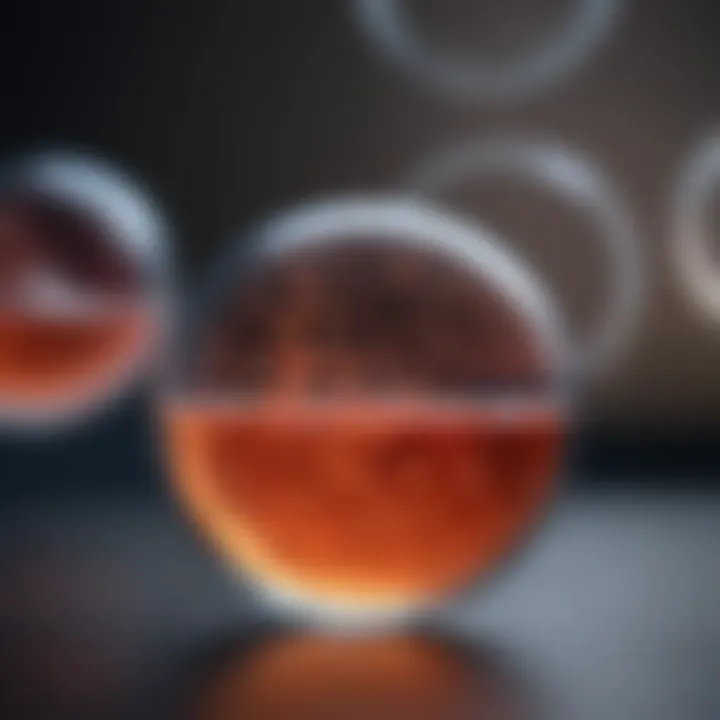
One of the most pressing issues in chemiluminescent assays is the risk of interference from various substances present in samples. Interference can arise from intrinsic components of the sample, such as proteins, lipids, and other biomolecules. These components can interact with assay reagents, leading to erroneous results.
False positives are particularly concerning as they can result in misleading interpretations. For instance, in clinical diagnostics, a patient may be falsely identified as having a condition due to such interference. This can lead to unnecessary stress, additional testing, and even invasive procedures. It is essential to implement rigorous controls and optimization procedures during assay development to mitigate these risks.
"The accuracy of chemiluminescent enzyme immunoassays can be severely compromised by unexpected interactions, necessitating extensive validation protocols."
Strategies to minimize interference include the use of purification techniques before assay applications and optimizing assay conditions like pH and temperature. Furthermore, deliberate controls, such as known sample concentrations, are vital in assessing assay robustness.
Cost and Resource Considerations
While chemiluminescent enzyme immunoassays provide high sensitivity and specificity, they may also impose significant costs on laboratories and clinics. The cost of reagents, enzymes, and specialized detection equipment can add up quickly. Additionally, there is often a need for expensive equipment that can facilitate accurate chemiluminescence detection, such as luminometers.
Resource limitations can be a considerable barrier, especially for smaller laboratories or research facilities with limited budgets. The continuous advancements in CLEIA technology may require frequent updates to equipment and reagents, posing another financial challenge. This necessitates careful planning and assessment of available resources prior to adopting these assays.
Emerging Trends in CLEIA Technology
Chemiluminescent enzyme immunoassays (CLEIA) have witnessed significant advancements over recent years, shaping the future of diagnostics and environmental monitoring. Understanding these trends is crucial for researchers and professionals alike. Key aspects include improvements in detection methodologies and the integration of microfluidic systems, which enhance both efficiency and accuracy of assays.
Advancements in Detection Methods
Recent years have seen important progress in detection methods used in CLEIA. These improvements are critical for enhancing assay sensitivity and specificity. Some notable advancements include:
- Use of Novel Chemiluminescent Substrates: Researchers have developed new substrates that increase the light output, allowing for lower concentrations of analytes to be detected effectively. This boosts the overall sensitivity of assays, making them more reliable in clinical and environmental settings.
- High-Throughput Screening: Advancements in automation technology now allow for high-throughput screening of samples. This not only speeds up the testing process but also improves the reproducibility of results. It enables laboratories to handle a larger volume of samples with consistent quality.
- Real-time Detection Techniques: Incorporating real-time luminescence detection methods helps monitor reactions as they occur. This can provide immediate insights into the assayโs progress and potential complications, allowing for quicker adjustments.
"The evolution in detection methodologies has transformed CLEIA into a more robust tool capable of meeting modern diagnostic needs."
Each of these advancements contributes to increasing the applicability of CLEIA across various domains, from clinical diagnostics to food safety testing.
Integration with Microfluidics
The combination of CLEIA with microfluidic technology marks a significant trend in the field. Microfluidics allows for the precise control of fluid movements across tiny channels, which enhances assay performance. Significant benefits arise from this integration:
- Reduced Reagent Consumption: Microfluidic systems require smaller volumes of reagents. This not only lowers costs but also minimizes waste, contributing to more sustainable laboratory practices.
- Improved Sensitivity and Speed: The miniaturization of assays facilitates quick reactions and lower limits of detection. This is particularly advantageous in urgent clinical scenarios where rapid results are essential.
- Point-of-Care Testing: Microfluidic devices can be designed for point-of-care applications, allowing for testing to be conducted outside traditional laboratory settings. This promotes accessibility to diagnostics, especially in remote areas.
- Automation Potential: Microfluidics offers significant automation capabilities, which streamline processes and reduce human error. This leads to more reliable outcomes and enhanced reproducibility.
Future Perspectives on Chemiluminescent Enzyme Immunoassays
The realm of chemiluminescent enzyme immunoassays (CLEIA) is evolving rapidly. This section will explore notable trends and future directions that may impact its application in research and healthcare. Given the increasing demand for accurate and rapid diagnostics, understanding these developments is essential.
Potential for Personalized Medicine
Personalized medicine represents a significant shift in healthcare, emphasizing tailored treatment strategies based on individual patient profiles. CLEIA stands poised to play a critical role in this transformative approach. By utilizing specific biomarkers, CLEIA can aid in the identification of personalized therapeutic regimens. This is particularly relevant in oncology, where tumor-specific markers can guide targeted therapy.
With advancements in bioinformatics and genomics, chemiluminescent enzyme immunoassays can be adapted to analyze more complex patient data. These adaptations could include leveraging genetic information to enhance assay specificity and sensitivity. Integrating CLEIA with next-generation sequencing may also allow for broader applications, enabling healthcare providers to make informed decisions based on comprehensive patient insights.
Overall, the potential for CLEIA in personalized medicine could lead to improved patient outcomes and more effective management of diseases.
Impact on Global Health Initiatives
Global health initiatives often focus on combating prevalent diseases, especially in under-resourced areas. The adaptability and efficiency of CLEIA offer substantial benefits in this context. It is already used in various public health campaigns targeting infectious diseases such as HIV and malaria. As technology progresses, we can expect more efficient, cost-effective CLEIA platforms to emerge.
One noteworthy aspect of this impact is the potential for deploying CLEIA technologies in remote regions. The simplicity and portability of chemiluminescent assays can facilitate point-of-care testing. This allows healthcare workers to conduct necessary diagnostics without access to fully equipped laboratories. Such advances can improve disease surveillance, resulting in timely interventions and better management of health crises.
Finale
The conclusion of this article serves a vital role in encapsulating the essential aspects of chemiluminescent enzyme immunoassays (CLEIA). To synthesize the findings presented, we need to reaffirm the significant advantages that this innovative technique holds in both clinical diagnostics and environmental monitoring.
Summarizing Key Takeaways
Chemiluminescent enzyme immunoassays have emerged as a preferred analytical method due to several key benefits:
- High Sensitivity: CLEIA can detect minute concentrations of target analytes, making it invaluable in clinical settings where precision is critical.
- Broad Application Spectrum: Whether for diagnosing infectious diseases or monitoring environmental contaminants, its applicability spans various fields.
- Rapid Results: The speed of obtaining results is advantageous, especially in time-sensitive situations such as medical emergencies.
- Cost-Effectiveness: Over time, although initial setups may be expensive, the benefits and efficiency of CLEIA methods often lead to reduced operational costs.
- Minimal Sample Volume Required: This feature is particularly important when working with limited samples, such as pediatric cases or rare specimens.
In summary, CLEIA not only streamlines processes but also enhances the analytical capabilities of laboratories.
The Importance of Continued Research
As we navigate forward, continued research in the domain of chemiluminescent enzyme immunoassays is imperative.
- Innovative Approaches: New methodologies and technologies are evolving. Enhancing the instrument's capabilities could further refine the sensitivity and specificity of these assays.
- Integration with Emerging Technologies: The synergy between CLEIA and advancements in fields such as microfluidics presents opportunities for miniaturized and more efficient assays.
- Global Health Impact: Ongoing research can yield insights that address pressing health challenges across various populations, helping to bridge gaps in healthcare access and quality.
- Educational Formats: Developing training and resources for future scientists and researchers ensures that knowledge is disseminated effectively.
"The advancements in CLEIA will be a cornerstone in future medical and environmental diagnostics, contributing to improved health and safety outcomes."