Exploring Axions: Theoretical Underpinnings and Experimental Pursuits

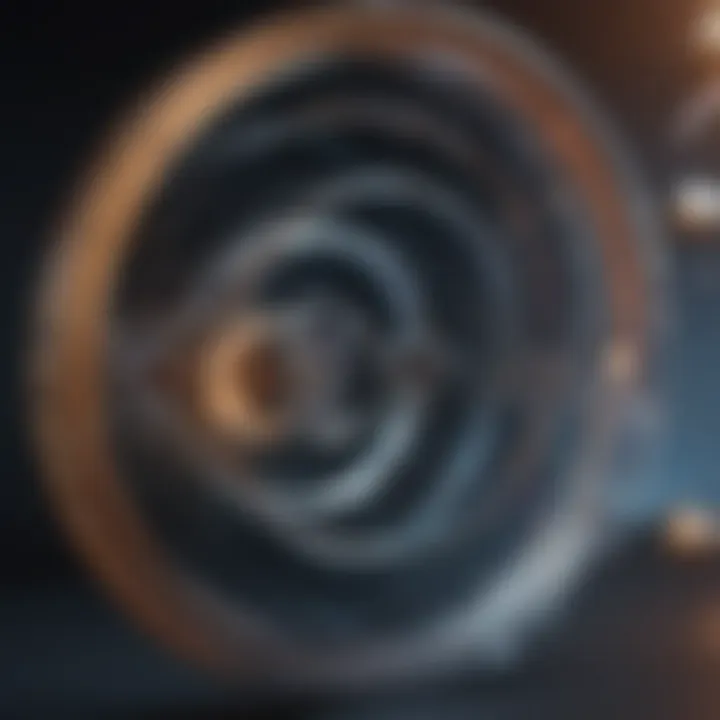
Intro
The investigation of axions presents a unique opportunity to understand some of the fundamental questions in physics. This elementary particle, theoretically proposed to resolve the strong CP problem in quantum chromodynamics, has commanded attention from both theoretical physicists and experimentalists alike. As we delve into axions, one may wonder about their origin, implications in dark matter research, and the ongoing experimental work aimed at detecting them. This article seeks to explore these aspects in detail and examine the broader ramifications of axion research.
Research Overview
Understanding the preliminary findings in axion research is vital. Researchers postulate that axions could play a significant role in resolving cosmic mysteries, particularly those surrounding dark matter.
Key Findings
- Axion hypothesis: Theoretical frameworks assert that axions could exist and contribute to dark matter.
- Experimental evidence: Several experiments, such as ADMX (Axion Dark Matter Experiment), are in motion to find these elusive particles.
- Interdisciplinary impact: Research on axions not only enriches particle physics but also deepens our understanding of cosmology and other domains.
Study Methodology
Numerous approaches are employed in axion research. Experimental methodologies mainly focus on indirect detection of axions through their interactions with photons. Various techniques include:
- Magnetic field exposure: Creating a high magnetic field environment can help convert axions into detectable photons.
- Statistical analysis: Rigorous analyses to measure the characteristics of phenomena predicted by axion theories.
- Collaboration: Interdisciplinary teams collaborate across institutions to share data and create comprehensive research strategies.
Background and Context
Historical Background
The axion was first introduced in the 1970s as a theoretical solution to issues in quantum chromodynamics. It was proposed by Roberto Peccei and Helen Quinn, leading to what is known as the Peccei-Quinn theory. The term 'axion' was subsequently coined by Frank Wilczek in the early 1980s. Over the decades, this particle has intrigued physicists looking for answers about the universe's make-up.
Current Trends in the Field
The field of axion research has experienced renewed vigor in recent years. As advancements in technology and experimental methods have emerged, the pursuit of axion detection intensifies. Notable current trends include:
- Increased funding for research initiatives focusing on dark matter and axions.
- Broader collaboration among institutions in academia and industry.
- Development of new experimental setups to enhance detectors’ sensitivity.
A comprehensive understanding of what axions represent in both theoretical and experimental physics opens new doors in unraveling the universe's intricate tapestry.
Preface to Axions
The exploration of axions represents a fascinating intersection of theoretical physics and experimental research. As hypothetical particles, axions are proposed solutions to longstanding problems in quantum chromodynamics, specifically the strong CP problem. This problem relates to the apparent absence of CP violation in strong interactions, a concept that implies the fundamental symmetries of physics could be violated in certain conditions. Axions, emerging from the work of Roberto Peccei and Helen Quinn, could play a crucial role in our understanding of matter at a fundamental level.
In this section, we will define axions, and also provide a historical backdrop that outlines significant developments leading to their conceptualization. Engaging with the introduction to axions is essential, as it lays the groundwork for appreciating their implications not only in particle physics but also in the broader context of cosmology and dark matter research. This understanding opens up discussions about the nature of the universe and the building blocks of matter.
Defining Axions
Axions are theoretically predicted elementary particles, characterized by their light mass and weak interaction with ordinary matter. They arise from a theoretical framework aimed at resolving specific anomalies in particle physics. The defining property of axions is their role as a pseudoscalar field, which can be linked with the dynamics of quantum fields.
One of the distinctive features of axions is their predicted coupling with photons. This coupling means that axions could potentially interact with electromagnetic radiation, thereby allowing for various experimental detection strategies. The lightness of axions contributes to their postulation as dark matter candidates, hinting at their potential abundance in the universe.
Moreover, the Peccei-Quinn theory introduces axions not merely as particles but as a mechanism that could fill gaps in current theoretical understanding. Their discovery could validate significant aspects of quantum field theory while reshaping our approach to nuclear interactions.
Historical Context and Development
The journey toward the formulation of axions began in the 1970s when the issue of CP violation in strong force interactions became apparent. Particle physicists observed a contradiction within the standard model, which suggested that CP symmetry should hold but showed signs of breaking in weak interactions.
Roberto Peccei and Helen Quinn proposed that introducing a new symmetry could lead to the resolution of this conundrum. They introduced what is now known as the Peccei-Quinn Symmetry, which required a new elementary particle: the axion. Subsequently, further theoretical work established the concept of axions as viable candidates to balance the equations governing particle physics.
As research progressed into the late 20th century, the axion gained traction not just as a puzzle piece in particle physics, but as a potential component of dark matter. This evolution underscored the interdisciplinary nature of axion research, linking it to cosmology and astrophysics.
Overall, the historical context forms a significant part of understanding axions, providing insights into how theoretical physics can evolve and influence various fields of study.
Theoretical Framework
The exploration of axions necessitates a detailed discussion of their theoretical framework. Understanding the principles of particle physics, specifically in the context of quantum chromodynamics (QCD), provides essential insights into not only the nature of axions but also their role in resolving longstanding questions in physics. A clear grasp of these theories is vital as it establishes the groundwork from which axion research evolves, addressing their predicted properties and interactions.
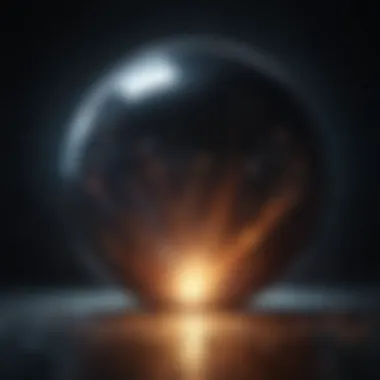
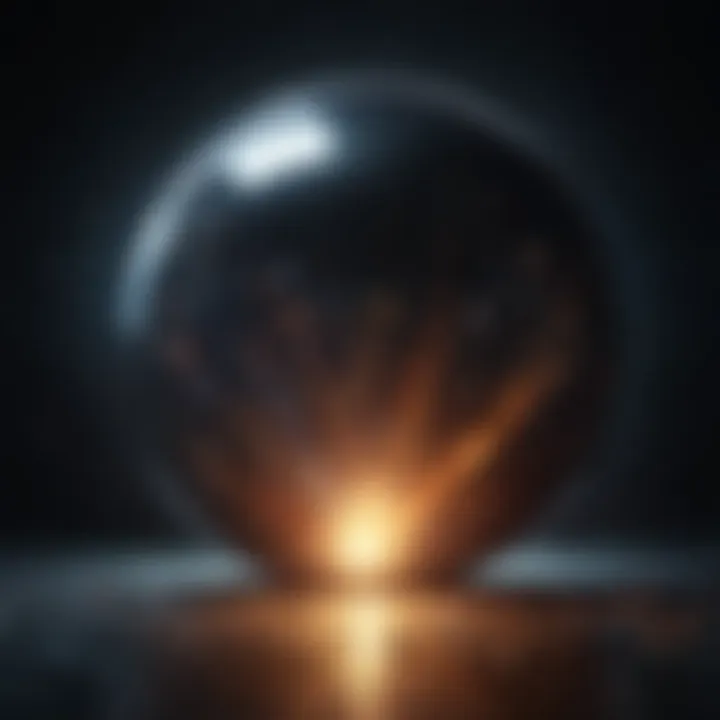
Quantum Chromodynamics and CP Violation
Quantum Chromodynamics, the theory describing the strong interaction between quarks and gluons, plays a fundamental role in the investigation of axions. Central to this theory is the concept of CP (Charge Parity) violation, which is pivotal for explaining baryogenesis—the process by which matter came to dominate over antimatter in the universe.
In the context of QCD, theoretical frameworks suggest that if CP violation occurs at a fundamental level, it could leave detectable signals, influencing the behavior of particles. However, the Standard Model does not provide a satisfactory explanation for the observed level of CP violation, leading to the strong CP problem.
The potential resolution lies in the original proposition that axions could offer a solution. The absence of observable consequences from strong CP violation suggests a need for new physics, which axions can potentially fulfill. By introducing this new particle, a more comprehensive model reconciling strong interactions with CP symmetry can be formulated.
The Axion Hypothesis
The axion hypothesis emerged as a promising solution to the complexities of QCD and CP violation. It theorizes the existence of a light, neutral particle that could influence the strong CP problem significantly. The core of this hypothesis lies in its connection to the Peccei-Quinn symmetry.
Connection to Peccei-Quinn Symmetry
The Peccei-Quinn symmetry provides a theoretical framework for why strong CP violation is not observed. According to this model, the symmetry allows for dynamic behavior of the QCD vacuum, which in turn gives rise to the axion as a pseudo-Nambu-Goldstone boson. This connection is paramount as it suggests that if axions exist, they would dynamically shield the effects of CP violation, explaining the current observations.
One key characteristic of this symmetry is that it preserves the underlying principles of quantum chromodynamics, while introducing additional parameters to account for observed phenomena. This trait makes it a favored theoretical avenue in the article—it presents a coherent addition to existing models of particle physics.
Its unique feature lies in the potential for axions to decay into photons, making them detectable through various experimental setups. This characteristic provides a tangible advantage in pursuing methods to uncover the presence of axions in our universe.
Implications for Strong CP Problem
The implications of axions for the strong CP problem are profound. If the axion hypothesis holds true, it not only resolves the discrepancies within QCD but also highlights the limitations of the current Standard Model.
The major characteristic of this implication is its capability to link the realms of particle physics and astrophysical observations. In a broader sense, it alters our understanding of the universe's fundamental forces and particles.
Moreover, the axion's potential of being a dark matter candidate adds a valuable layer to its relevance. This makes it an attractive subject for both theoretical and experimental physicists, connecting the investigation of particle physics with cosmological implications. However, debates persist about the specifics of axion properties and the pertinence of their detection in the context of established physics. Addressing these challenges is crucial for harnessing the axion hypothesis effectively in resolving the strong CP problem.
Axions and Dark Matter
Understanding the role of axions in the context of dark matter is essential for both theoretical and experimental physics. Axions are proposed as candidates for dark matter due to their unique properties. Given that ordinary matter accounts for a small fraction of the universe's total mass-energy content, the existence of dark matter is crucial for explaining numerous cosmological phenomena, such as galaxy formation and gravitational lensing. Integrating axions into the dark matter framework offers a compelling narrative that not only addresses theoretical issues but also drives experimental searches in the field.
The Role of Axions in Cosmology
In cosmology, axions might address the mysterious components of the universe beyond what is observed. They emerge from theories related to quantum chromodynamics and are connected to the strong CP problem. Dark matter axions are theorized to interact only weakly with normal matter, which makes them particularly challenging to detect but plausible as a substantial component of dark matter.
The contribution of axions to the universe's energy density could follow a Bose-Einstein condensation, forming a coherent cloud that influences structure formation. This means that as the universe expanded and cooled, axions may have taken on a quantum state that helps explain large-scale structures we observe today.
"In theoretical astrophysics, solving the dark matter conundrum is one of our highest priorities. Axions present an enticing solution."
Axion Dark Matter Candidates
Axion dark matter falls into specific categories based on their predicted mass and behavior. Various models propose different mass ranges for axions, typically between nano-eV to micro-eV. Here are some notable candidates:
- Standard Axions: These arise from the Peccei-Quinn theory and are the most widely discussed.
- Axion-Like Particles (ALPs): They include a broader range of particles that share some properties with standard axions but do not directly address the strong CP problem.
Each candidate has distinct implications for cosmology and particle physics. For instance, high-mass axions might influence structure formation differently than lighter candidates, which may exhibit wave-like properties that could lead to observable effects in the Cosmic Microwave Background.
Current searches for axion dark matter involve sophisticated experimental designs aimed at exploring these candidates in varying mass ranges. Understanding the precise nature of axions remains critical for advancing both theoretical frameworks and empirical methodologies in astrophysics and cosmology.
Detection Methods
Detection methods are a crucial part of the search for axions. Their elusive nature poses a significant challenge. The goal is to identify signs of axions through various experimental techniques. Understanding these methods provides insights into the practicalities and complexities we face in this research area.
Experimental Techniques for Axion Detection
Experimental techniques for axion detection can be categorized into specific strategies. Two notable methods are photon regeneration experiments and searches for axion-like particles. Both approaches aim to uncover evidence of axions and their properties.
Photon Regeneration Experiments
Photon regeneration experiments utilize the interaction between photons and axion-like particles. The fundamental aspect of these experiments is to detect changes in light under specific conditions. These experiments rely on the premise that axions can convert photons into other forms of energy. This unique feature allows researchers to explore axion parameters indirectly.
The key characteristic that makes photon regeneration experiments popular is their capability to test a wide range of axion properties. They offer a relatively simple design compared to other methods, making them accessible. However, they face drawbacks as well. The experimental setup can be sensitive to various background noises, which may hinder accurate readings.
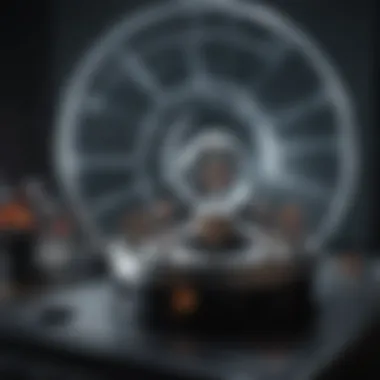
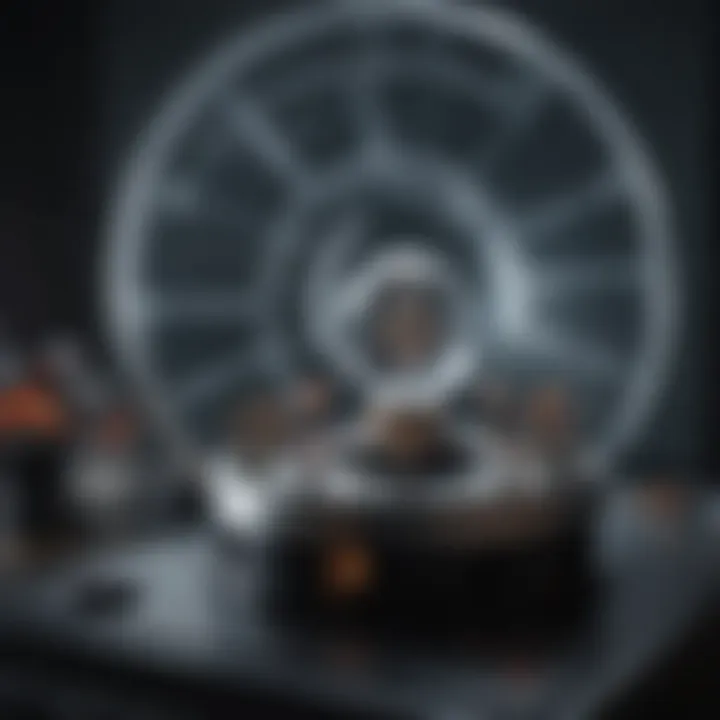
Search for Axion-like Particles
The search for axion-like particles employs a different tactic. This method focuses on direct detection of particles that exhibit similar properties to axions. It often involves high-energy environments, similar to conditions in the early universe. The main advantage of this approach is that it opens new avenues to search for particles beyond traditional axions, potentially yielding significant discoveries.
One distinctive feature of these searches is their ability to identify a broader spectrum of particles. This feature is beneficial because it increases the likelihood of discovering new physics phenomena. However, the experiments require advanced technology and may be limited by existing experimental facilities or budget constraints.
Current Experiments and Their Progress
Several experiments are currently in progress that focus on detecting axion particles. Among the notable projects are CAPP, ADMX, and CAST. Each experiment has unique characteristics and methodologies that contribute to the overall understanding of axion physics.
CAPP
CAPP, located in South Korea, is focused on the development of axion search experiments. Its contribution lies in employing innovative techniques to significantly improve sensitivity. A key characteristic of CAPP is its collaboration with universities, enhancing research capacity. The facility uses unconventional methods that can provide new insights. This collaboration is advantageous; however, it may also mean a reliance on external funding sources.
ADMX
ADMX, or the Axion Dark Matter Experiment, is aimed specifically at detecting axion dark matter. One main feature that gives ADMX its prominence is its use of a high-Q superconducting resonator. This design enhances the ability to detect low energy signals. ADMX has made substantial progress, with iterations improving its technology over time. The challenge is still present; increasing the sensitivity means more complexity in the experimental setup.
CAST
The CAST experiment, situated at CERN, focuses on solar axions. It uses a unique approach by tracking axions produced from the sun and analyzing them. A distinctive feature of CAST is its innovative use of a magnet to detect axions. This method, while innovative, can limit the range of axion masses that can be probed. The results obtained so far from CAST provide valuable arguments for or against various axion theories.
Understanding the methods and current experiments is key to advancing axion research. With each development, we gain a clearer view of the potential nature and role of axions in the universe.
Implications of Axion Research
The exploration of axions carries significant implications for both theoretical physics and our understanding of the universe. These implications extend beyond basic research, impacting various scientific disciplines and paving the way for potential breakthroughs in multiple fields. In this section, we discuss the influence of axion research on particle physics and cosmological models, both of which are critical for enhancing our knowledge of the fundamental components and mechanisms underlying the universe.
Impact on Particle Physics
Axions present a unique challenge to current particle physics models. Their existence could validate certain theoretical frameworks that explain strong interactions. The strong CP problem is one of the mystery areas where axion theories provide insights. Addressing this issue through axion research may refine or reformulate existing particle physics theories, offering new avenues for exploration.
The integration of axions into particle physics leads to
- New physics predictions: If deteced, axions could suggest the presence of additional particles or forces, enriching our current understanding of reality.
- Validation of symmetries: The study of axions tests the validity of the Peccei-Quinn symmetry, a significant theoretical construct in quantum chromodynamics.
- Interactions with other particles: Understanding how axions interact with photons or other particles is crucial for building comprehensive frameworks within particle physics.
Researchers are also interested in how axions might influence other theoretical models, such as Grand Unified Theories (GUTs). By examining the implications of axions, scientists may gain insights into the unification of the fundamental forces.
Influence on Cosmological Models
The potential role of axions in cosmology is profound. As candidates for dark matter, their discovery could reshape cosmological models that describe the universe's structure and evolution. Current models struggle to account for the mysterious nature of dark matter, and axions, if found to be a valid component, could provide a crucial piece of that puzzle.
Key points regarding the influence of axions on cosmological models include:
- Understanding galaxy formation: Axions could help explain the distribution of matter in the universe and the formation of galaxies. Their properties may align with dark matter's behavior, offering resolutions for anomalies in current models.
- Cosmic Microwave Background (CMB) radiation: The interaction of axions with other cosmic components may have implications on how we interpret observations of the CMB. This may advance our understanding of the universe's infancy and the processes that led to its current state.
- Insights into the universe's fate: The characteristics of axions can influence theories about the universe's ultimate fate, including scenarios related to expansion and collapse.
The exploration of axions is not merely an academic exercise; it has the potential to reshape our grasp of fundamental physics and the origins of the cosmos.
Interdisciplinary Connections
In the field of axion research, the exploration of interdisciplinary connections serves as a critical lens through which the complexities of these theoretical particles can be appreciated. Axions straddle multiple domains, including particle physics, cosmology, astrophysics, and even aspects of string theory. This blending of disciplines not only enriches the context in which axions are studied but also broadens the implications of findings across varied scientific realms.
Axions and Astrophysics
The interplay between axions and astrophysics is a compelling aspect of contemporary research. Astrophysical phenomena, such as cosmic microwave background radiation and the structure formation of the universe, raise significant questions that axions may help answer. For instance, if axions exist, they could play a substantial role in the makeup of dark matter, which constitutes a large portion of the universe's mass-energy content. Understanding the distribution and properties of dark matter through the lens of axion physics invites new methodologies and approaches in astrophysics.
Some critical points regarding axions and astrophysics include:
- Cosmic Implications: If axions are a form of dark matter, their interactions and effects would influence galactic formation and behavior.
- Observation Opportunities: Axions could have subtle footprints in cosmic background radiation, leading to potential methods for detection from astronomical observations.
- Unification of Concepts: Examining axions within astrophysical frameworks allows for a merging of theories that were previously considered separate.
Collaboration with Other Scientific Disciplines
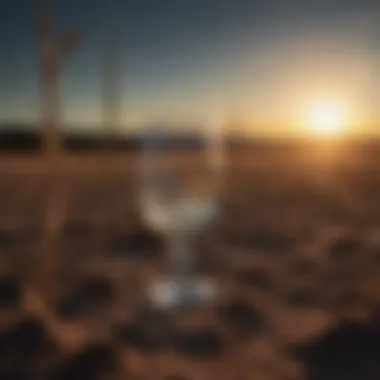
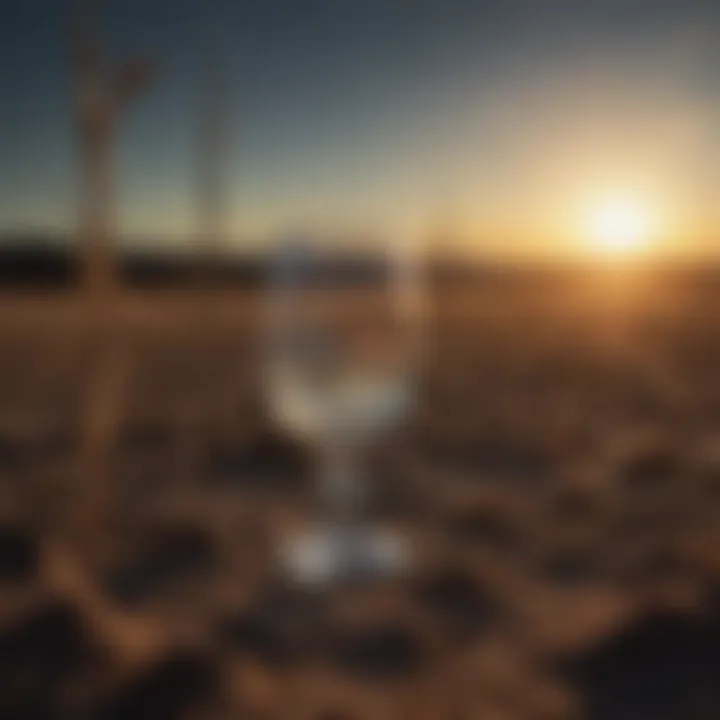
Collaboration is essential when tackling the multifaceted nature of axion research. Input from various scientific disciplines can promote a constructive dialogue, facilitating breakthroughs that are beyond the scope of any one field. This holistic approach applies not only to theoretical considerations but also to technological advancements in detection methods.
Key benefits of interdisciplinary collaboration include:
- Innovative Detection Techniques: Insights from engineering and technology can lead to the development of advanced experimental setups, maximizing the chances of axion detection.
- Cross-Pollination of Ideas: Theories from different domains can complement each other, fostering creativity and presenting unique avenues of exploration.
- Broadening the Research Community: Inclusion of experts from other fields can widen the pool of knowledge, thereby enhancing the academic discourse and leading to a more enriched understanding of axions.
"Engaging scientists from diverse fields not only empowers axion research but also fosters a community capable of tackling complex scientific challenges effectively."
Ultimately, the interdisciplinary connections within axion research illuminate the interconnectedness of modern science. Bridging gaps across various domains can uncover new insights and advance our understanding of fundamental questions regarding the universe.
Future Directions and Challenges
The exploration of axions presents not only fascinating theoretical implications but also a myriad of challenges that must be addressed in future research. Understanding these challenges is crucial as they influence the trajectory of discovery in the field of particle physics. This section discusses unresolved questions in axion physics and the technological hurdles in axion detection, which must be overcome to further our knowledge and understanding.
Unresolved Questions in Axion Physics
Axions, while compelling in theory, still evoke several unanswered questions that invite investigation. A primary inquiry revolves around the mass of these particles. Current estimates suggest a wide range, yet precision in this measurement is essential for both experimental searches and theoretical models. Several interpretations of quantum chromodynamics predict different mass ranges, leading to uncertainty.
Moreover, understanding the coupling constants for axions with photonic fields remains another significant challenge. Establishing precisely how axions interact with other particles could illuminate their role in cosmic evolution and contribute significantly to dark matter research.
Some additional unresolved questions include:
- The Nature of Axion-like Particles: Are there particles resembling axions in behavior that could also address the strong CP problem?
- Thermal History of Axions: How did axions form in the early universe? Understanding their production mechanism is key to their role in cosmology.
Finally, researchers must grapple with the broader implications of possible axion existence. If they do exist, how could they reshape our understanding of fundamental physics? Addressing these questions is vital for a comprehensive grasp of axions and their implications in our universe.
Technological Hurdles in Detection
Detecting axions requires sophisticated technology and innovative methodologies, often presenting significant hurdles to researchers. One of the most pressing challenges is the sensitivity required in detection experiments. Axions interact with electromagnetic fields at incredibly low rates, necessitating detectors that can minimize noise and other sources of interference.
Another challenge lies in the design of experimental setups. Current methods include converting axions into detectable photons via strong magnetic fields, which requires precise alignment and calibration. The feasibility of these experiments hinges not just on technology but also on the computational capacity to analyze extensive datasets generated during experiments.
Current detectors, such as the Axion Dark Matter Experiment (ADMX), are already advancing in sensitivity, but scaling these efforts effectively is a formidable task. Addressing this challenge demands not just improved technology but also increased collaboration among interdisciplinary teams to develop novel detection schemes.
In summary, addressing both the unresolved questions in axion physics and the technological hurdles in their detection will be crucial for advancing our understanding of these intriguing particles. Progress in these areas holds the potential to not only unravel the mysteries of axions but also contribute significantly to our grasp of dark matter and the fundamental fabric of the universe.
"Understanding the challenges in axion research not only shapes future inquiries but also contributes to a more profound comprehension of the universe's underlying principles."
Research in this domain is ever-evolving, and continual advancements in technology and methodology will likely lead to breakthroughs that were once deemed unattainable.
Culmination
The conclusion serves a crucial role in synthesizing the vast discussions presented in this article. As a comprehensive exploration of axions and their theoretical underpinnings, the conclusion allows readers to grasp the significance of axions not only as a theoretical concept but also in their potential application to unresolved queries in modern physics.
Summary of Current Understanding
In recent years, axions have emerged as a pivotal element in understanding the dynamics of the universe. They are proposed as candidates for dark matter and may also provide insights into conventions in quantum chromodynamics. Their existence would help resolve the long-standing strong CP problem, affirming theoretical frameworks while simultaneously influencing experimental physics. Current theoretical efforts and models provide a coherent landscape for axion properties, parameters, and their potential interactions. Researchers believe that discovering axions could redefine our understanding of gravity and cosmology. The synthesis of historical context, theoretical frameworks, and experimental pursuits showcases how important axions are in bridging different realms of physics. As such, comprehending the implications of axions is imperative not only for physicists but for anyone interested in the structure and history of the universe.
The Future of Axion Research
Looking forward, axion research continues to navigate through various challenges while holding promise for revolutionary breakthroughs in particle physics. Current unanswered questions about axion properties remain at the forefront. For example, researchers still debate the exact mass of axions, their couplings to photons, and whether they possess particle-like traits or behave more like waves. Technological advancements are pivotal; with growing investments in groundbreaking detection methods, including photon regeneration and specialized particle colliders, the landscape may shift dramatically in the near future. Collaborative efforts across multiple disciplines, such as astrophysics and cosmology, further augment these investigations. The intricacies of axions might eventually unlock new paths to understanding dark matter, potentially unveiling phenomena that could redefine physics as we know it.
In summary, axions represent not just a theoretical curiosity but a key to understanding the universe better. Their exploration encompasses theoretical and experimental domains, encouraging further research and collaboration.
Key Publications and Articles
- Peccei, R.D., and Quinn, H.R. (1977): The foundational paper that introduced the concept of axions. This publication is essential for understanding the theoretical basis of axions and their connection to the strong CP problem.
- Wilczek, F. (1978): Expanding on the axion model, Wilczek's work elaborates on the implications of axions in cosmology, making it a crucial read for grasping their potential role in dark matter.
- ADMX Collaboration (2000-2023): Ongoing reports and findings from the Axion Dark Matter Experiment provide invaluable insights into the current experimental techniques and challenges faced in detecting axions.
These publications are not just academic pieces; they embody the evolution of thought and technological advancement within the realm of particle physics.
Further Reading Suggestions
To gain a broad perspective on axions and related topics, the following reading materials are recommended:
- "Axions: Theory and Experiment" by A. Ringwald et al.
This book outlines the theoretical aspects of axions, their implications, and details about experimental strategies. - "The Strong CP Problem and Axion Cosmology" by R. D. Peccei and H. R. Quinn.
A comprehensive exploration of the origins and implications of axions in cosmological models. - Wikipedia article on Axions
A good starting point for understanding the basics, with links to primary sources and further references.
Visit Wikipedia
Each of these resources offers unique insights that complement the information presented in this article, encouraging readers to explore the subject matter with greater depth.