Investigating Cardiomyocyte Biology and Function
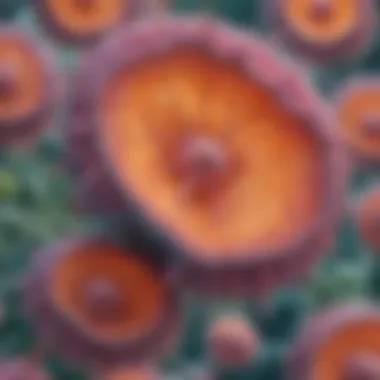
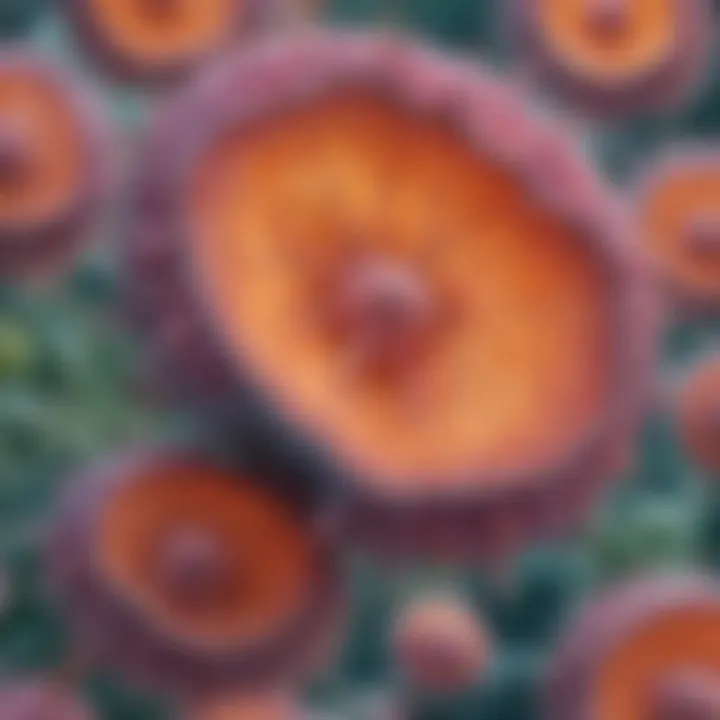
Intro
Cardiomyocytes, or cardiac muscle cells, are essential for the proper functioning of the heart. They orchestrate the contraction and relaxation cycles that pump blood throughout the body. Understanding their biology is key for grasping not only cardiac health but also the complex mechanisms that underlie various heart diseases. This article aims to provide an in-depth exploration of cardiomyocyte cells, focusing on their biology, function, and broader implications for cardiac health.
Research Overview
Key Findings
Recent studies reveal that cardiomyocytes have unique properties that distinguish them from other muscle types. They are huge, multi-nucleated cells specialized for contraction. One significant finding is their limited regenerative capability, which poses challenges for recovery from heart injuries. This has led to an increased interest in the potential of stem cell therapy to regenerate damaged heart tissue using cardiomyocytes.
Study Methodology
Investigations into cardiomyocyte biology utilize various methodologies including:
- Cell culture techniques to study cardiomyocyte growth and behavior in vitro.
- Animal models to assess how cardiomyocytes function in a living organism, especially following cardiovascular events.
- Molecular biology techniques to understand the genetic and biochemical pathways that regulate cardiomyocyte behavior.
Background and Context
Historical Background
The study of cardiomyocytes dates back to the 19th century when scientists began to explore muscle physiology. The identification of these unique cells significantly advanced with the invention of microscopy, allowing researchers to observe cardiac tissues in detail. Early research focused on the heart’s electrical properties, leading to the discovery of how cardiomyocytes generate and conduct electrical signals, which is fundamental to heart rhythm.
Current Trends in the Field
Presently, research is focused on understanding cardiomyocyte differentiation and maturation processes. There is also an emphasis on regenerative medicine, exploring how to harness the heart’s limited ability to heal. Key areas of interest include:
- The role of induced pluripotent stem cells in generating functional cardiomyocytes.
- Innovations in gene editing technologies, like CRISPR, to correct genetic defects in cardiomyocytes.
- Investigation of the signaling pathways that lead to cardiomyocyte hypertrophy, a key factor in heart disease.
Cardiomyocytes are not just passive elements of the heart's structure; they play active roles in responding to physiological and pathological stimuli.
In summary, a comprehensive understanding of cardiomyocytes is essential for advancing knowledge in cardiac biology and therapy. As the field progresses, integrating cardiomyocyte research into broader cardiovascular studies will contribute to innovative treatments and fundamentally enhance our approach to heart health.
Prolusion to Cardiomyocytes
Understanding cardiomyocytes is essential in the realm of cardiovascular research and medicine. These cells are central to heart function, serving as the contractile units that enable the heart to pump blood. When we explore the biology and function of cardiomyocytes, we uncover critical insights into not just heart muscle function, but also the underlying mechanisms that lead to various cardiac diseases. The importance of cardiomyocytes extends to their role in health and regeneration, making them a focal point for therapeutic innovations.
Definition and Importance
Cardiomyocytes are specialized muscle cells that constitute the bulk of the heart's tissue. They exhibit a unique ability to contract rhythmically and spontaneously, which is essential for maintaining the heart's pumping action. A characteristic feature of cardiomyocytes is their striated appearance, which results from the organized arrangement of contractile proteins such as actin and myosin.
The importance of cardiomyocytes cannot be overstated. They are involved in the intricate processes of cardiac contraction and electrical conduction. Their functionality ensures effective blood circulation, oxygen delivery, and nutrient transportation to all parts of the body. Without healthy cardiomyocytes, the heart would not be able to perform its vital functions, which can lead to serious health issues, including heart failure and arrhythmias.
Historical Perspective
The study of cardiomyocytes has evolved over time. Early investigations focused primarily on the physiological aspects of heart function. Scientists observed the heart's rhythmic contractions and sought to determine the mechanisms behind these processes.
As technology progressed, research expanded into cellular and molecular levels. Advances in microscopy and biochemistry allowed for a clearer understanding of the cellular structure and functions. The identification of ion channels, signaling pathways, and the role of calcium ions in contraction revolutionized our comprehension of cardiac physiology.
In recent decades, the discovery of stem cell biology has opened new avenues for understanding cardiomyocyte development and regeneration. Historical advancements now set the stage for contemporary studies that seek to address cardiac disorders through innovative strategies such as regenerative medicine and gene therapy.
"The evolution of our understanding of cardiomyocyte biology reflects a journey from basic physiology to a complex network of cellular interactions essential for heart health."
In summary, cardiomyocytes are indispensable for heart function. Their historical study showcases how scientific inquiry has led to transformative insights, establishing a baseline for ongoing research that holds promise for improved therapies for heart disease.
Anatomy of Cardiomyocytes
Understanding the anatomy of cardiomyocytes is critical in grasping their functional contributions to the heart. It elucidates how these cells' specialized architecture supports their role in maintaining cardiac function and stability. Each element within these cells plays a part in their ability to generate and conduct electrical impulses, contract efficiently, and respond to physiological demands. This segment will break down the structure and important components of cardiomyocytes, touching upon cell structure, mitochondrial density and function, and the significance of intercalated discs.
Cell Structure
The cardiomyocyte presents a unique and highly specialized cell structure that is vital for its role in the heart. These cells are generally elongated and cylindrical in shape, which equips them for contraction. Each cardiomyocyte contains a centrally located nucleus, a defining feature that distinguishes them from skeletal muscle cells, which are multi-nucleated.
Cardiomyocytes are packed with myofibrils, which are the contractile apparatus of the cell. These myofibrils consist of repeating units known as sarcomeres, essential for muscle contraction.
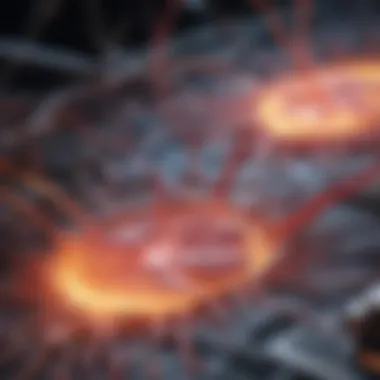
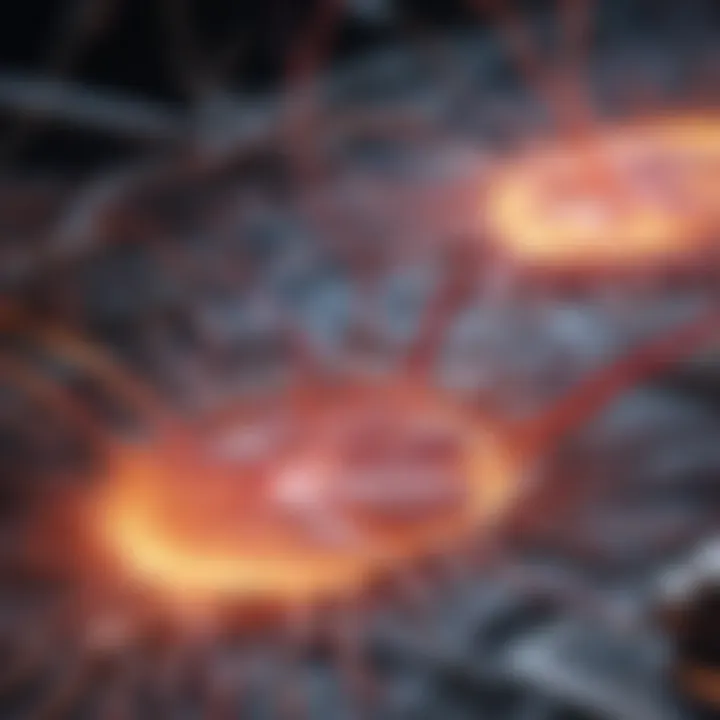
Key features of cardiomyocyte structure include:
- Striations: These are created by the orderly arrangement of actin and myosin filaments within the sarcomeres.
- Sarcoplasmic Reticulum: This organelle stores calcium ions, crucial for muscle contraction.
- T-Tubules: These tubular extensions of the cell membrane help in quickly spreading the action potential throughout the cell.
Mitochondrial Density and Function
Mitochondria are often referred to as the powerhouses of the cell, and this holds especially true for cardiomyocytes. These cells have a notably high density of mitochondria, approximately 30% of their total volume, which reflects their significant energy requirements.
Cardiomyocytes rely heavily on aerobic metabolism to generate adenosine triphosphate (ATP), essential for sustaining contraction over prolonged periods.
The role of mitochondria in cardiomyocytes includes:
- ATP Production: Mitochondria convert nutrients into ATP, fulfilling the energy demand during the contraction cycles.
- Calcium Handling: They also help regulate intracellular calcium levels, playing a role in cardiac signaling and muscle contraction.
- Apoptotic Pathway: Dysfunctional mitochondria can signal cell death, which has implications in heart disease.
Intercalated Discs
Intercalated discs are a defining characteristic of cardiomyocytes, setting them apart from other muscle cells. These structures are specialized connections between adjacent cardiomyocytes. They facilitate communication and synchronization, enabling the heart to function as a cohesive unit.
Intercalated discs contain two primary components:
- Desmosomes: These are responsible for mechanically linking cardiomyocytes, helping them withstand the stress of continuous contractions.
- Gap Junctions: These junctions permit direct electrical coupling between cells, allowing for rapid transmission of action potentials and coordinated rhythmic contractions.
Collectively, these features form a comprehensive package that allows cardiomyocytes to perform their essential roles efficiently. Understanding the anatomy of these cells is not only fundamental in basic biological research but also imperative when investigating cardiac pathologies and potential therapeutic strategies.
Developmental Biology of Cardiomyocytes
Understanding the developmental biology of cardiomyocytes is pivotal for comprehending how these cells form and function in the heart. This section highlights the processes by which cardiomyocytes originate and mature, offering insights into their unique characteristics that are essential for cardiac health. The study of cardiomyocyte development bridges the gaps between fundamental biology and clinical applications, especially in regenerative medicine. Learning about development can help find solutions to heart diseases, making this knowledge invaluable.
Origin and Differentiation
Cardiomyocytes originate from specialized progenitor cells in the early stages of heart development. These progenitor cells are known as mesodermal precursors, which play a crucial role in forming the cardiac tissue. During embryogenesis, signals from surrounding tissues instruct these precursors to differentiate into cardiomyocytes. This process involves complex gene regulatory networks that guide the cell's fate.
Several key transcription factors, such as Nkx2.5 and GATA4, are critical in regulating the development of cardiomyocytes. They activate the expression of specific genes necessary for heart muscle formation. Understanding these mechanisms is fundamental, as any disruption can lead to congenital heart defects. Moreover, the proper differentiation of these cells is vital for the efficient functioning of the heart throughout the life of an organism.
The differentiation process occurs in distinct phases. Initially, mesodermal cells aggregate to form a heart tube, where they begin to specialize into cardiomyocytes. Eventually, these cells undergo further maturation, acquiring the ability to contract and generate electrical signals necessary for heart rhythm. The sequence of events leading to cardiomyocyte differentiation is a rich area for research, especially for potential therapeutic applications in regenerative medicine.
Growth and Maturation
After differentiation, cardiomyocytes enter the growth and maturation phase. This phase involves significant increases in both cell size and contractile protein synthesis. Unlike many other cell types in the body, cardiomyocytes have limited regenerative capacity. Thus, understanding how they grow and mature is essential for developing therapies for heart injuries or diseases.
The maturation of cardiomyocytes is dependent on several factors, including mechanical load, electrical stimulation, and the biochemical environment. During this phase, cardiomyocytes establish their characteristic structure, including the formation of intercalated discs, where cells connect and communicate. These connections are critical for synchronized contraction across the heart muscle.
During development, cardiomyocytes can undergo hypertrophy, a compensatory response to increased workload. This is important for maintaining heart function during growth and response to stress. However, prolonged hypertrophy can lead to heart disease, highlighting the delicate balance required in the growth and maturation of these cells.
"The understanding of cardiomyocyte growth and maturation is key to addressing the challenges posed by heart disease and injury."
Research in this area aims to mimic the intrinsic growth signals in the laboratory, which could pave the way for cell-based therapies treating heart disease. Advances in stem cell technology and tissue engineering are particularly promising, as they might allow for the generation of functional cardiomyocytes that can integrate with existing cardiac tissue.
Functional Role of Cardiomyocytes
Cardiomyocytes play a critical role in the proper functioning of the heart. They are not just muscle cells; they are the powerhouse of the heart's mechanics. Understanding their functional role is essential for both basic biology and the study of cardiovascular diseases. This section delves into the aspects that define their importance in cardiac health.
Electrophysiology
Electrophysiology refers to the electrical properties and activities of cardiomyocytes. These cells possess unique ion channels that generate action potentials, essential for heart contractions. Key ions such as sodium, potassium, and calcium flow in and out of the cells, leading to depolarization and subsequent repolarization. This process allows cardiomyocytes to contract rhythmically, which is crucial for maintaining an effective heartbeat.
The sinoatrial node orchestrates the initial electrical signals that spread through the heart. Deviation in these signals due to damaged cardiomyocytes can result in arrhythmias, which affect overall heart function. Research continues to explore how electrical signaling within cardiomyocytes can be harnessed or corrected to address heart rhythm disorders.
Contractile Mechanisms
The contractile mechanisms of cardiomyocytes involve complex interactions between different proteins, primarily actin and myosin. When calcium ions enter the cells during the action potential, they bind to troponin, initiating the contraction process. This interaction causes the myosin heads to pull on actin filaments, shortening the muscle fiber and resulting in heart contraction.
Proper functioning of these contractile proteins is vital for cardiac output. Any disruption, whether by genetic mutations or external factors, can lead to diminished heart function and may manifest as diseases like cardiomyopathy. Exploring these mechanisms provides insights into potential therapeutic targets for heart disease treatment.
Role in Cardiac Rhythm and Contractility
Cardiomyocytes not only contract but also play a significant role in maintaining the heart's rhythm and overall contractility. The coordinated contraction of heart chambers ensures optimal blood flow throughout the body, and cardiomyocytes are key players in this process. The balance between contraction and relaxation phases is crucial for effective heart performance.
Disruptions in this rhythm can lead to serious conditions such as atrial fibrillation. Researchers aim to understand how cardiomyocytes process signals to maintain this rhythm and how to mitigate adverse effects during rhythm disturbances. Techniques like gene therapy are being explored to repair faulty rhythmic signaling, showing promise for future treatments.
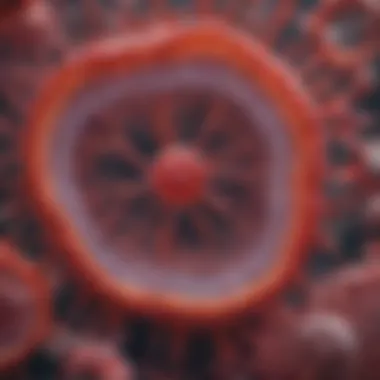
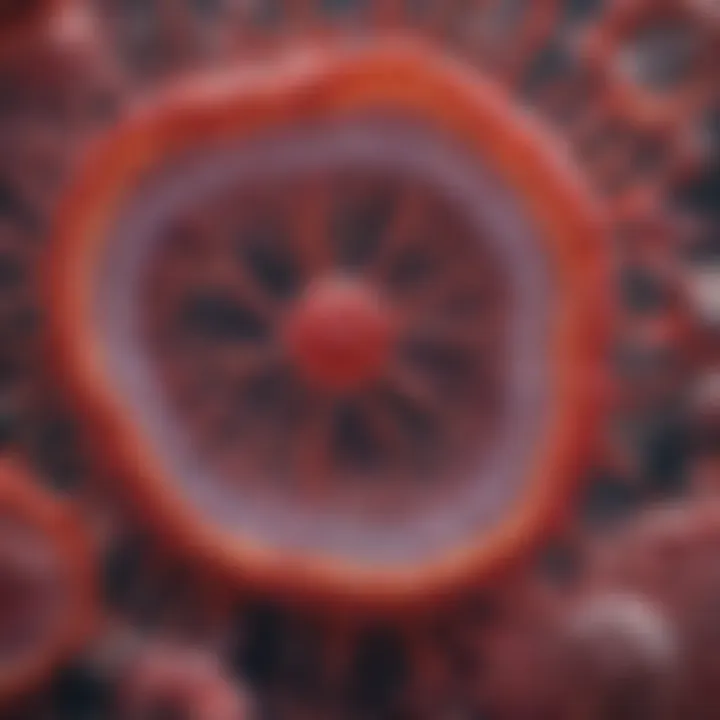
Understanding the functional roles of cardiomyocytes is vital for unraveling the complexities of cardiac physiology and pathology.
In summary, cardiomyocytes are indispensable for the heart's electrical and mechanical functions. Their electrophysiological properties, contractile mechanisms, and ability to maintain cardiac rhythm make them a central focus of cardiac research. Further exploration of these elements holds the potential for innovative treatment strategies aimed at improving heart health.
Cardiomyocytes in Health and Disease
Understanding cardiomyocytes' role in health and disease is crucial for comprehending the complexities of cardiac function and dysfunction. These specialized muscle cells are central to maintaining a healthy heart and play pivotal roles when disease processes occur. By delving into specific elements like cardiac hypertrophy, ischemic heart disease, and heart failure mechanisms, we gain insights into how these cells contribute to both normal physiology and pathological conditions.
Role in Cardiac Hypertrophy
Cardiac hypertrophy is the process by which cardiomyocytes increase in size, often in response to increased workload or stress. This adaptation is a double-edged sword. Initially, it allows the heart to cope with increased demands, such as during intense exercise. However, prolonged hypertrophy can lead to detrimental effects, including heart failure.
In hypertrophy, regulatory pathways involving cytokines and growth factors become activated. There is an increase in protein synthesis and a shift in gene expression toward fetal isoforms of contractile proteins. This process may result in the heart trying to adapt to pathophysiological stress but can ultimately lead to a decrease in functional efficiency.
"Understanding cardiac hypertrophy provides crucial insights into preventive measures against heart failure."
Ischemic Heart Disease
Ischemic heart disease arises when cardiomyocytes do not receive sufficient blood supply, often due to coronary artery blockage. The inadequate oxygen supply leads to cell injury and, potentially, cell death. Ischemia profoundly affects cardiomyocyte function, reducing their ability to contract effectively and leading to arrhythmias and other complications.
Recent studies have demonstrated that cardiac ischemia also triggers a complex, adaptive response at the cellular level. Cardiomyocytes may attempt to protect themselves by activating survival pathways, but extended ischemic conditions can overwhelm these mechanisms. Therapeutic strategies involving reperfusion and cellular protection are being researched to restore blood flow and limit damage to cardiomyocytes in ischemic conditions.
Heart Failure Mechanisms
Heart failure is a multifactorial condition where the heart is unable to pump enough blood to meet the body’s needs, and cardiomyocyte function is deeply compromised. Various mechanisms contribute to heart failure, including altered calcium handling, increased apoptosis, and changes in energy metabolism within cardiomyocytes.
In heart failure, cardiomyocytes exhibit hypertrophy, fibrosis, and metabolic shifts. This complexity highlights the necessity for targeted therapeutic interventions. Current research is exploring strategies to rejuvenate cardiomyocytes through cell-based therapies and gene editing, aiming to restore their function and improve cardiac output.
Through understanding these challenges and mechanisms, researchers can develop more effective treatments for cardiovascular diseases, ultimately benefitting patient outcomes.
Regenerative Potential of Cardiomyocytes
The regenerative potential of cardiomyocytes is a focal point in cardiac research. Given the heart's essential role in maintaining circulation, understanding how these cells can regenerate is vital for developing therapies to treat heart diseases. Cardiomyocytes, once damaged, have a limited innate ability to repair themselves. Thus, it becomes critical to explore enhancing their regenerative capabilities, which could lead to substantial advancements in clinical practices regarding heart repair.
Natural Regeneration Limitations
Cardiomyocytes have a notably low intrinsic capacity for regeneration. After heart injury, such as myocardial infarction, the surviving cardiomyocytes undergo hypertrophy rather than true regeneration, leading to heart muscle remodeling. The formation of scar tissue results in loss of function, contributing to heart failure. Several factors inhibit cardiomyocyte regeneration:
- Cell Cycle Arrest: Mature cardiomyocytes are largely post-mitotic. Most cardiomyocytes exit the cell cycle soon after birth, which limits their ability to proliferate.
- Regenerative Microenvironment: The heart's environment post-injury is typically not conducive for regeneration. Inflammatory responses and fibrotic tissue formation can hinder healing processes.
- Oxidative Stress: Ischemic events lead to increased oxidative stress, which can damage cardiomyocytes further and inhibit their regenerative capacity.
Addressing these limitations is essential for improving cardiac repair mechanisms and requires an in-depth understanding of the underlying biology of cardiomyocytes.
Induced Pluripotent Stem Cells
Induced pluripotent stem cells (iPSCs) present a promising avenue for overcoming the regenerative limitations of cardiomyocytes. Researchers can reprogram somatic cells, such as skin or blood cells, into iPSCs. These cells exhibit pluripotency, meaning they can differentiate into any cell type, including cardiomyocytes.
- Differentiation: The ability to derive cardiomyocytes from iPSCs allows for the generation of patient-specific cells that can be used for personalized therapies.
- Novel Therapies: iPSCs can serve as a source for cell therapy, potentially replacing damaged cardiomyocytes and restoring heart function.
- Research Models: iPSCs also provide valuable models for studying cardiac diseases and drug testing, leading to better understanding and treatment options.
Despite these advantages, challenges remain in ensuring that iPSC-derived cardiomyocytes integrate effectively into the host heart and function appropriately.
Tissue Engineering Approaches
Tissue engineering offers a multifaceted strategy to augment cardiomyocyte regeneration. By creating biologically compatible scaffolds, researchers attempt to mimic the natural extracellular matrix that supports cell growth and tissue formation.
- Scaffolds: These structures can be designed to provide support for cardiomyocytes, aiding in their survival and function after transplantation.
- Biomaterials: The choice of materials impacts cell attachment, proliferation, and differentiation. Materials such as hydrogels and decellularized matrices are being explored for their potential to support cardiomyocytes.
- Combined Approaches: Integrating growth factors or stem cells within engineered tissues can enhance the regenerative capacity of cardiomyocytes. This could lead to improved outcomes in cardiac repair.
Current Research Directions
Research in cardiomyocyte biology is rapidly evolving, reflecting a growing recognition of their essential roles in cardiovascular health and disease. Understanding current research directions not only directs future studies but also helps in translating findings into viable therapies. Each avenue explored contributes significantly to the landscape of cardiac medicine and unveils new potential for intervention.
Gene Therapy Innovations
Gene therapy holds promise for correcting genetic defects that contribute to cardiac diseases. Advances in delivery systems have improved how therapeutic genes can be introduced into cardiomyocytes. These innovations include viral vectors and nanoparticles, which can enhance the efficiency of gene transfer.

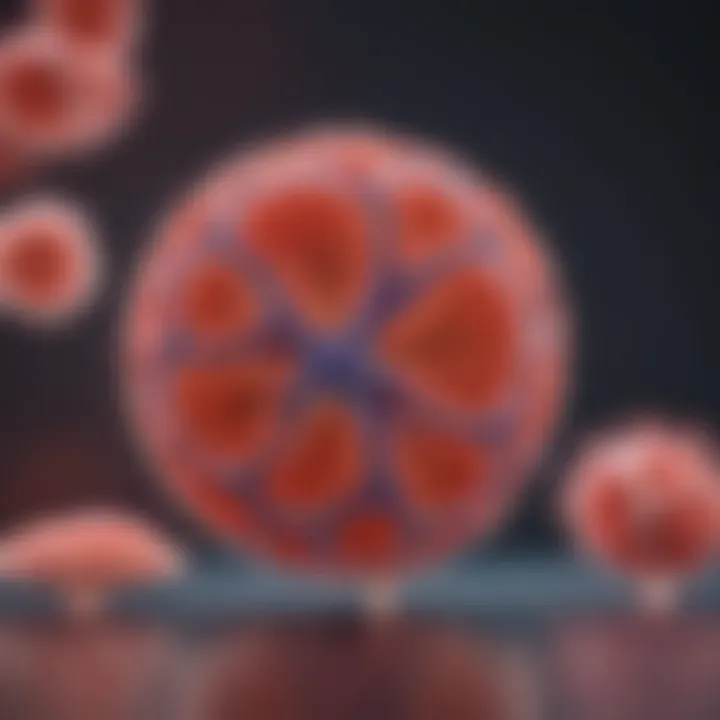
Recent studies have shown potential in using CRISPR-Cas9 technology to edit genes linked to common heart conditions. By targeting specific mutations, researchers aim to restore normal function in diseased myocardial tissue. Such targeted approaches can also facilitate the study of the relationships between genetic variations and cardiac function, providing insights that were previously unattainable.
Biomaterials in Cardiomyocyte Research
The integration of biomaterials in cardiomyocyte research has opened new pathways for understanding cellular behavior and tissue engineering. Materials such as hydrogels and scaffolds can mimic the native extracellular matrix, offering a platform for studying cardiomyocyte interaction and drug responses.
These biomaterials can be engineered to release growth factors or other bioactive compounds, promoting cardiomyocyte survival and proliferation in vitro. This approach is critical for tissue engineering applications; it helps create viable cardiac patches for patients afflicted by heart injuries.
Immunomodulation in Cardiac Repair
Immunomodulation presents a transformative strategy for cardiac repair. It involves modifying immune responses to improve healing after myocardial infarction or injury.
Research indicates that targeting immune cells can influence the inflammatory response, potentially reducing damage to cardiomyocytes during the repair process. For instance, therapies aimed at promoting a reparative or anti-inflammatory environment can enhance the regenerative capacity of the heart.
Understanding the complex interplay between cardiomyocytes and the immune system continues to be an essential focus of research. Studies suggest that enhancing this relationship can lead to innovative treatments that bolster recovery post-cardiac events.
"Addressing the immune component in cardiac repair can significantly influence outcomes following tissue injury."
Challenges in Cardiomyocyte Research
The investigation of cardiomyocytes is crucial for advancing our understanding of heart diseases and their treatment. However, researchers face significant challenges that can hamper progress in this field. Challeneges in cardiomyocyte research include technical limitations and ethical considerations, both of which necessitate careful navigation to improve therapeutic interventions and enhance scientific knowledge.
Technical Limitations
Cardiomyocyte research is often hindered by various technical restrictions. These include limitations in models used for experimentation. For example, while animal models provide insight into cardiac function, they may not fully replicate human cardiomyocyte behavior. This discrepancy can lead to variances in research outcomes and assumptions based on those findings.
Another technical issue involves the isolation and culture of cardiomyocytes. Primary cardiomyocytes from human tissues are notoriously difficult to obtain, leading researchers to rely on cell lines or induced pluripotent stem cells. While these alternatives show promise, they might not exhibit the same physiological characteristics as native cardiomyocytes.
The complexity of cardiomyocyte signaling pathways adds another layer of difficulty. A comprehensive understanding of these pathways is necessary for developing targeted therapies. Yet, the intricate networks governing cardiomyocyte function are still not fully elucidated.
"Understanding the technical nuances of cardiomyocyte research can bridge the gap between lab findings and clinical applications."
Ethical Considerations
In addition to technical challenges, ethical concerns persist in cardiomyocyte research. The use of human cells for research purposes raises questions about consent and the potential exploitation of donors. It is imperative that researchers adhere to strict guidelines to ensure the ethical treatment of tissue donors and providers.
Moreover, the advent of induced pluripotent stem cell technology has opened new ethical debates. These stem cells, derived from adult somatic cells, offer hopes for regenerative medicine but also confront inquiries regarding the source and manipulation of human cells. Researchers must prioritize transparency and establish clear protocols that uphold ethical standards in their pursuits.
Another consideration is the impact of research on vulnerable populations, particularly in clinical trials. Strategies to balance scientific advancement with the protection of participant rights must be foundational in any cardiomyocyte research endeavor.
In summary, tackling the challenges associated with cardiomyocyte research is essential for future advancements. Researchers must navigate technical barriers while also addressing the ethical implications their work entails, thus ensuring a responsible approach to uncovering the full potential of cardiomyocytes in therapeutic applications.
Finale and Future Perspectives
Understanding cardiomyocytes is essential due to their critical role in cardiac health. This article illustrates the structure, function, and significance of these cells, while highlighting the advancements in research and therapeutic approaches related to them. Cardiomyocytes are not only foundational to the heart's anatomy but also serve key physiological roles such as contraction and impulse conduction. It is important to recognize their involvement in various cardiac conditions, where their impairment often leads to severe consequences for overall heart function.
Future perspectives in cardiomyocyte research invite optimism. As technological advances and innovative methodologies become available, researchers can further explore the pathways that dictate cardiomyocyte behavior. The promise of regenerative medicine could transform our approach to managing heart disease, tapping into the cellular capabilities that have yet to be fully understood and harnessed.
Summation of Key Points
This article has covered several significant aspects regarding cardiomyocytes, focusing on their
- Unique cellular structure and associated functions
- Role in heart development and pathology
- Implication in various heart diseases, such as ischemic heart disease and heart failure
- Possible advancements in cardiomyocyte-based therapies, including tissue engineering and gene therapy
The exploration conducted herein serves as a foundation for future inquiries into cardiomyocyte biology, stressing the necessity for ongoing research.
Implications for Clinical Practice
The implications of cardiomyocyte research extend significantly to clinical practice. Understanding these cells can guide the development of novel treatments that address heart diseases effectively. For instance, insights into cardiomyocyte function can encourage the creation of targeted therapies that mitigate injury or boost regeneration after myocardial infarction.
Furthermore, as new treatments arise from regenerative medicine, clinicians will have alternative strategies for managing heart conditions. The shift toward personalized medicine requires a deep knowing of cardiomyocyte biology, elevating the importance of continued research in this area.
Future Research Avenues
Future research avenues in cardiomyocyte studies are diverse and promising. Key areas poised for exploration include:
- Gene editing technologies: Assess the potential of CRISPR and other gene therapy tools to correct genetic defects in cardiomyocytes.
- Three-dimensional cardiac tissue models: Focus on developing in vitro systems that accurately mimic heart tissue for drug testing and studying disease pathology.
- Biomimetic materials: Explore materials that closely resemble the natural environment of cardiomyocytes to enhance regeneration and integration of stem cells or engineered tissues
- Longitudinal studies: Investigate the long-term effects of therapies targeting cardiomyocytes to establish their efficacy and safety.
Research in these domains could yield valuable insights that not only advance fundamental biology but also pave the way for groundbreaking clinical treatments.