Unraveling the Complexities of Organic Chemistry
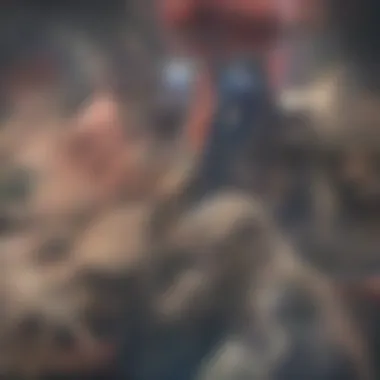
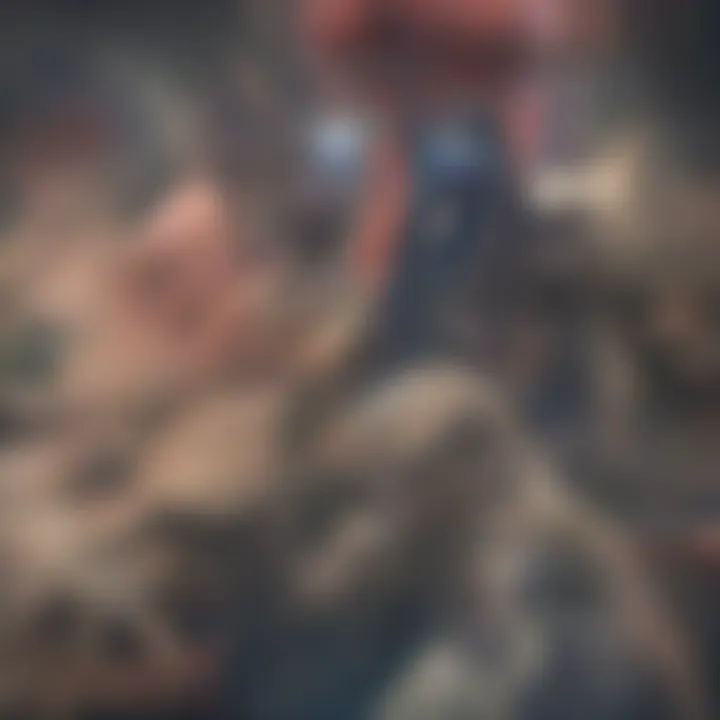
Research Overview
Organic chemistry is a vast field of study that focuses on the structure, properties, and reactions of organic compounds. These compounds fundamentally include carbon and often hydrogen, alongside other elements like oxygen, nitrogen, sulfur, and halogens. The significance of organic chemistry cannot be overstated, as it forms the backbone of various industries, including pharmaceuticals, plastics, and agriculture. Understanding this discipline is crucial for students, educators, and professionals alike.
Key Findings
Several key findings have emerged in recent years:
- An increase in the utilization of green chemistry principles, promoting sustainable practices in the production of organic compounds.
- Advances in synthetic methods leading to more efficient pathways for the creation of complex molecules.
- The expansion of organic chemistry applications in areas like materials science and nanotechnology.
These findings illustrate the ongoing evolution of the discipline and its implications for both theoretical research and practical applications.
Study Methodology
The methodologies applied in organic chemistry research are varied and sophisticated. Researchers often employ:
- Spectroscopy: Techniques such as NMR and infrared spectroscopy are used to analyze molecular structures.
- Chromatography: This separates components in a mixture, essential for purity analysis of substances.
- Computational Chemistry: Simulations aid in predicting molecular behavior and reaction outcomes.
By employing these methods, organic chemists can gain deeper insights into compound behavior and facilitate innovation in the field.
Background and Context
Historical Background
The discipline of organic chemistry has its roots in the 19th century. It began with the understanding of simple carbon compounds and gradually expanded to include more complex structures. Pioneers like Friedrich Wöhler, who synthesized urea from ammonium cyanate, challenged the notion that organic compounds could only come from living organisms. This marked a significant turning point, leading to the synthetic approaches we see today.
Current Trends in the Field
Today, organic chemistry is influenced by several current trends:
- Interdisciplinary Approaches: The interplay between chemistry, biology, and materials science is notable, especially in drug design and biomaterials.
- Sustainability Focus: There is a growing trend toward minimizing waste and using renewable resources in chemical processes.
Researchers are continuously finding new ways to apply organic chemistry principles in various fields, indicating its paramount importance in addressing global challenges.
"The evolution of organic chemistry has not only pushed scientific boundaries but also opened doors to practical solutions in everyday life."
Understanding this interplay is vital for any academic or professional engaged with the subject.
Foreword to Organic Chemistry
Organic chemistry is a field that warrants extensive exploration due to its significance across various scientific disciplines and practical applications. This section serves as a primer, unpacking the essence of organic chemistry and its crucial role in enhancing our understanding of the chemical world. By examining the fundamental principles that govern organic compounds, one can appreciate their relevance to both academic inquiries and everyday applications.
Through a detailed analysis of organic chemistry, we can understand how it impacts areas such as medicine, environmental science, and biotechnology. The insights gained from this examination are valuable for students, researchers, educators, and industry professionals alike.
Defining Organic Chemistry
Organic chemistry is fundamentally the study of carbon-containing compounds, encompassing a vast array of substances that include hydrocarbons, alcohols, acids, and polymers. The versatility of carbon is unmatched, as it can form stable bonds with various elements, leading to an infinite variety of molecular structures and functionalities.
To accurately define organic chemistry, one must consider both its composition and its functional aspects. It includes the study of:
- The structures of organic molecules
- The reactions they undergo
- The mechanisms by which these reactions occur
Understanding these elements is vital for progressing in fields such as pharmacology, where the design and reactivity of organic compounds play a key role.
Historical Context
The historical evolution of organic chemistry traces back to ancient practices, where the focus was on natural substances. From early medicinal practices to the isolation of compounds from plants, the roots of organic chemistry lie deeply embedded in human history. The differentiation from inorganic chemistry was marked by the 19th century, as pioneers like Friedrich Wöhler synthesized urea from ammonium cyanate, challenging the prevailing notion that organic compounds could only be derived from living organisms.
This pivotal moment paved the way for the modern understanding of organic chemistry. Since then, the field has expanded significantly, witnessing the introduction of structural formulas in the late 1800s, which allowed deeper insight into molecular architecture. The continuous progression of organic chemistry through various milestones reflects its dynamic nature and critical importance in scientific advancement.
"Organic chemistry is the study of compounds that contain carbon, and its principles are foundational for understanding the complexity of living systems."
In essence, the historical context of organic chemistry is not just about past developments; it also lays the groundwork for contemporary research and applications across a spectrum of fields.
Key Principles of Organic Chemistry
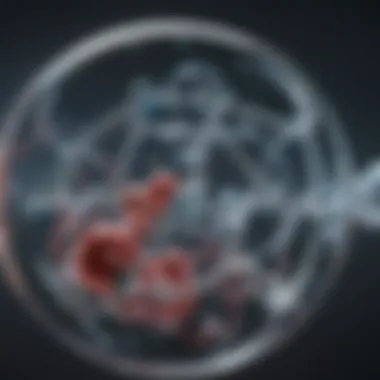
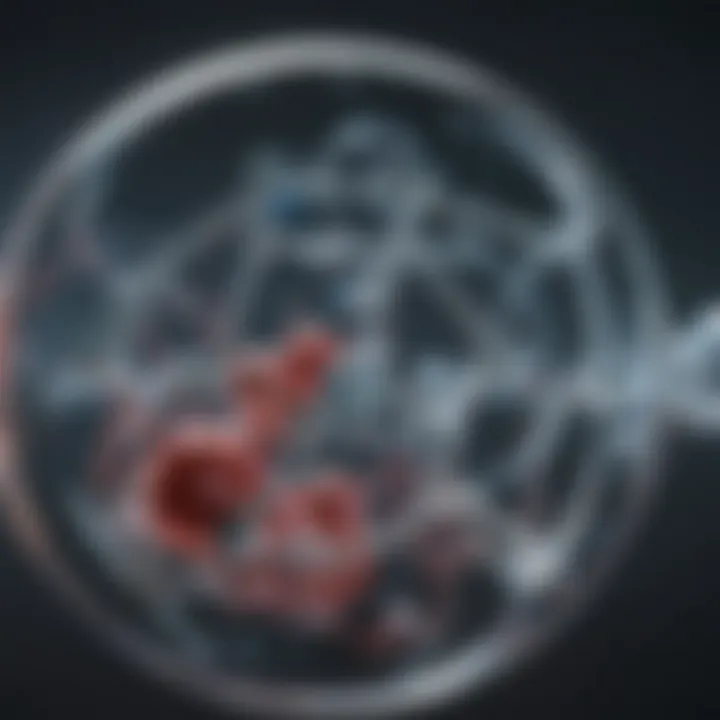
The study of organic chemistry is foundational to understanding how compounds behave and interact in various environments. This section elucidates key principles that govern organic chemistry, providing insight into the fundamental features such as bonding, functional groups, and isomerism. Grasping these principles is essential for students, researchers, and professionals who aim to navigate the complexities of chemical processes and applications.
Bonding and Structure
At the core of organic chemistry lies the bonding structure of carbon atoms. Carbon possesses a unique ability to form four covalent bonds with other atoms. This tetravalence allows for a vast diversity of molecules including chains, rings, and frameworks. The types of bonds—single, double, and triple—significantly influence the properties of a compound. Furthermore, hybridization plays a vital role in determining molecular geometry and reactivity. For instance:
- sp3 hybridization leads to tetrahedral structures, typical in alkanes.
- sp2 hybridization results in planar structures, common in alkenes and aromatic compounds.
- sp hybridization gives rise to linear arrangements found in alkynes.
Understanding these bonding types is critical for predicting the behavior of organic molecules in both theoretical and practical settings.
Functional Groups
Functional groups are specific groups of atoms within molecules that dictate the chemical reactions they undergo. Identifying these groups is crucial for the classification and understanding of organic compounds. Each functional group imparts distinct characteristics to the molecule, influencing solubility, acidity, and reactivity. Common functional groups include:
- Hydroxyl (-OH): characteristic of alcohols, influencing polarity.
- Carboxyl (-COOH): present in carboxylic acids, contributing to acidity.
- Amino (-N): found in amines, affecting basicity.
Each functional group can participate in various reactions, shaping the pathway for synthesis or degradation of compounds. By grasping the role of functional groups, chemists can strategically design new molecules for specific applications in fields such as pharmaceuticals and materials science.
Isomerism
Isomerism refers to the existence of compounds that have the same molecular formula but differ in structure or spatial arrangement. This principle introduces complexity and variety in organic molecules, leading to different properties and functions. There are two primary types of isomerism:
- Structural Isomerism: Compounds differ in the connectivity of atoms.
- Chain isomers: Variations in the carbon backbone.
- Position isomers: Different locations of functional groups.
- Stereoisomerism: Compounds have the same connectivity but differ in spatial orientation.
- Geometric isomers (cis/trans): Differ in the arrangement around a double bond.
- Enantiomers: Non-superimposable mirror images, significant in biological activity.
Understanding isomerism is crucial because it affects the reactivity and interactions within biological systems and industrial applications.
In summation, these key principles—bonding and structure, functional groups, and isomerism—form the backbone of organic chemistry. Mastery of these concepts provides the tools necessary to explore the intricate world of organic molecules and their applications in various scientific disciplines.
Reactions in Organic Chemistry
Organic chemistry is fundamentally defined through the reactions that occur among organic compounds. These reactions underlie all of chemistry and are critical to understanding how molecules interact and transform. This section will present a thorough exploration of various types of reactions as well as mechanisms that govern these transformations. Understanding the nuances of organic reactions leads to improved synthesis strategies and application in diverse fields, ranging from pharmaceuticals to materials science.
Types of Reactions
In organic chemistry, reactions can be categorized into several types based on their characteristics and processes. Fans of organic chemistry must grasp these classifications, as they provide insight into how compounds behave under different conditions. Key reaction types include:
- Substitution Reactions: One functional group in a molecule is replaced by another. For example, in the halogenation of alkanes, hydrogens are substituted with halogens like chlorine or bromine.
- Elimination Reactions: These reactions lead to the removal of a small molecule, such as water or hydrogen halide, resulting in the formation of a double or triple bond. Dehydrohalogenation of alkyl halides is a common example.
- Addition Reactions: Characteristic of unsaturated compounds, these involve addition of elements or groups across a double or triple bond, leading to a saturated compound.
- Redox Reactions: In these, electron transfer occurs between chemical species, leading to oxidation and reduction. Important in both biological and industrial contexts, this type encompasses a wide array of organic transformations.
- Rearrangement Reactions: Atoms in the molecules reorganize to form different structures, often providing isomers. An example includes the conversion of cyclohexanol to methylcyclopentyl alcohol.
Each of these reaction types possesses unique characteristics and implications, influencing organic synthesis and application in various industries. Understanding these reactions expands the scope of organic chemistry to realms like drug design and environmental science.
Mechanisms of Reactions
The mechanism of a reaction describes the step-by-step process by which reactants transform into products. This details not only what happens but also how it occurs. A significant aspect of organic chemistry, studying mechanisms allows chemists to predict reaction outcomes and design new reactions more effectively.
Key considerations in reaction mechanisms include:
- Nucleophiles and Electrophiles: Understanding the roles these species play in reactions is fundamental. Nucleophiles donate electron pairs, while electrophiles accept them.
- Reaction Intermediates: Many reactions proceed through unstable intermediates, such as carbocations or carbanions, which are critical for determining reaction pathways.
- Energy Profiles: The energy required to convert reactants to products, often illustrated through reaction coordinate diagrams, indicates whether a reaction is favorable.
- Catalysis: Catalysts alter the pathway of a reaction, often lowering the energy barrier to increase reaction rates. The role of enzymes in biological systems exemplifies the importance of catalysts in organic reactions.
"A deep understanding of organic reactions unlocks the potential for innovation across various scientific fields."
By focusing on these concepts, an organic chemist can more effectively communicate findings and generate solutions to emerging challenges in the world.
Organic Compounds and Their Applications
Organic compounds play a vital role in a broad range of fields, such as medicine, agriculture, and materials science. Their unique properties stem from carbon's ability to form stable bonds with a variety of elements. Understanding these compounds is not just academic; it directly affects everyday life and future innovations.
The significance of organic compounds can be highlighted through several key aspects:
- Diversity: The sheer variety of organic compounds is astonishing. From simple hydrocarbons to complex biomolecules, organic chemistry encompasses a wide range of structures and functions.
- Functional Applications: Many organic compounds are essential in pharmaceuticals. They serve as the basis for many drugs, as they can be designed to interact with biological systems effectively.
- Environmental Impact: Organic chemistry also encompasses compounds relevant to sustainability and environmental science. Research in this area focuses on biodegradable materials and green chemistry.
In this section, we will explore three main classes of organic compounds: alkanes and alkynes, aromatic compounds, and their biological significance.
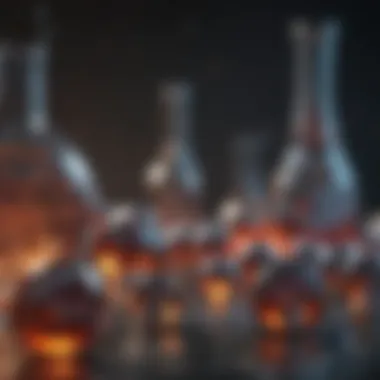
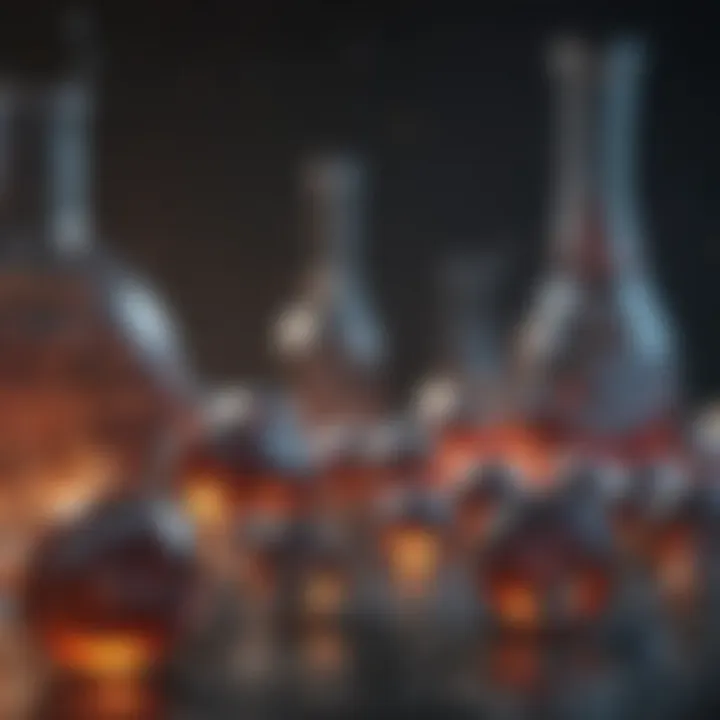
Alkanes and Alkynes
Alkanes are saturated hydrocarbons, meaning they contain only single bonds. They are generally less reactive than other organic compounds, which makes them quite stable. Common examples include ethane and propane, both of which have significant applications as fuels.
In contrast, alkynes are unsaturated hydrocarbons with at least one triple bond, making them more reactive. Acetylene is a well-known alkyne, primarily used in welding and as a chemical building block. The distinct structural characteristics of these compounds lead to various applications, such as:
- Energy Sources: Both alkanes and alkynes are crucial in energy production. They serve as fuels in household heating and transportation.
- Synthetic Intermediates: Alkenes can be used to synthesize a variety of other chemical compounds, expanding their utility in chemical manufacturing.
Aromatic Compounds
Aromatic compounds are characterized by their stable ring structures and delocalized pi electrons. Benzene is the most notable example, valued for its stability and unique chemical behavior. The applications of aromatic compounds are extensive, including:
- Industrial Chemicals: Many aromatic compounds are used in the production of plastics, dyes, and detergents due to their stability and chemical properties.
- Pharmaceuticals: Several drugs are derived from aromatic compounds, demonstrating their importance in medicinal chemistry. For instance, aspirin is synthesized from salicylic acid, an aromatic compound.
"Aromatic compounds exhibit unique properties that make them indispensable in a variety of applications, particularly in designing new materials and medications."
Biological Significance
The relevance of organic compounds in biological systems cannot be overstated. Organic molecules are at the core of life itself, as they form the backbone of biomolecules such as proteins, nucleic acids, carbohydrates, and lipids. Their functions include:
- Metabolism: Organic compounds play a central role in cellular metabolism. They are involved in energy production and the synthesis of key biological molecules.
- Structural Components: Many organic compounds provide structural support for cells and tissue, enabling their organization and function.
- Genetic Information: Nucleic acids, which are organic compounds, carry genetic information essential for heredity and cellular function.
In summary, the study of organic compounds and their applications is crucial to advancing science and technology. By understanding these compounds' structures, properties, and functions, researchers can develop new materials and medications that improve quality of life. This exploration of organic compounds provides a stepping stone to future innovations that continue to shape our world.
Analytical Techniques in Organic Chemistry
Analytical techniques play a crucial role in organic chemistry. They provide methods to analyze, identify, and quantify organic compounds. Understanding these techniques is key for anyone working in chemistry, as they bridge the gap between theory and practical application. The reliability of results achieved through these methods impacts research outcomes and product development across various fields.
Techniques such as spectroscopy, chromatography, and mass spectrometry are essential tools in laboratories. They reveal insights not only about the composition of substances but also about their structure, properties, and potential uses. This section delves into each method, discussing their principles, applications, and significance in the biochemical landscape. Using the right technique often determines the success of a project, highlighting the importance of these skills for students, researchers, educators, and industry professionals alike.
"Analytical chemistry forms the bedrock of organic chemistry studies, providing clarity in compound identification and characterization."
Spectroscopy
Spectroscopy is a technique that analyzes the interaction of light with matter. It allows chemists to gather information about the molecular structure of compounds. Various forms of spectroscopy exist, including infrared (IR), nuclear magnetic resonance (NMR), and ultraviolet-visible (UV-Vis) spectroscopy.
- Infrared Spectroscopy (IR) helps identify functional groups by measuring molecular vibrations. This information can be pivotal when distinguishing between similar compounds.
- Nuclear Magnetic Resonance (NMR) provides detailed information about the structure of organic molecules, including the arrangement of atoms. It uses the magnetic properties of atomic nuclei, offering insights into molecular dynamics and environment.
- Ultraviolet-Visible Spectroscopy (UV-Vis) is useful for assessing the electronic transitions in molecules. It often provides data on concentration and purity.
Employing spectroscopy significantly reduces the time it takes to analyze a sample. The insights gained can lead to quicker conclusions, which is vital in research and development settings.
Chromatography
Chromatography encompasses numerous techniques designed for separating mixtures. This method is based on the principle of partitioning, where compounds are distributed between two phases, typically a stationary phase and a mobile phase.
Some widely used forms of chromatography include:
- Gas Chromatography (GC) is appropriate for volatile substances. It allows for high-resolution separation and is useful in various fields including environmental testing and forensics.
- Liquid Chromatography (LC), such as High-Performance Liquid Chromatography (HPLC), is essential for analyzing more complex compounds, including pharmaceuticals and biological samples.
- Thin-Layer Chromatography (TLC) is a simple and cost-effective method for monitoring reactions and assessing compound purity.
The ability to efficiently separate components in a mixture broadens research capabilities. It can elucidate complex reactions or identify unknown substances, making chromatography indispensable in organic analysis.
Mass Spectrometry
Mass spectrometry is another critical analytical technique. It measures the mass-to-charge ratio of ions. This technique enables the precise determination of molecular weights and structural information of compounds.
The primary steps in mass spectrometry involve:
- Ionization of the sample, converting it into charged particles.
- Analysis of the resulting ions based on their mass-to-charge ratios.
- Detection of ions, yielding a spectrum that interprets the sample composition.
Mass spectrometry can be coupled with chromatography to enhance its capabilities. For instance, using GC-MS allows for comprehensive analysis of volatile organic compounds. Insights from mass spectrometry can lead to critical developments in drug testing and environmental monitoring.
Interdisciplinary Connections
In the realm of science, few fields create bridges to other disciplines as effectively as organic chemistry. The interplay between organic chemistry and other scientific domains is essential, enriching both theoretical knowledge and practical applications. By connecting organic chemistry to materials science and pharmaceutical development, researchers are not only furthering their understanding of chemical processes but also generating innovative solutions to complex challenges.
Organic Chemistry and Materials Science
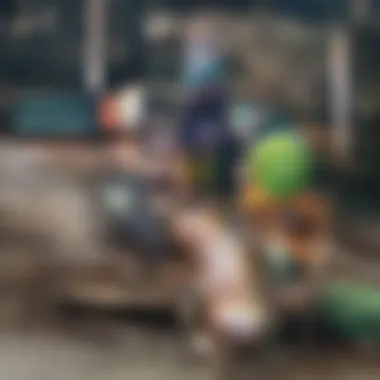
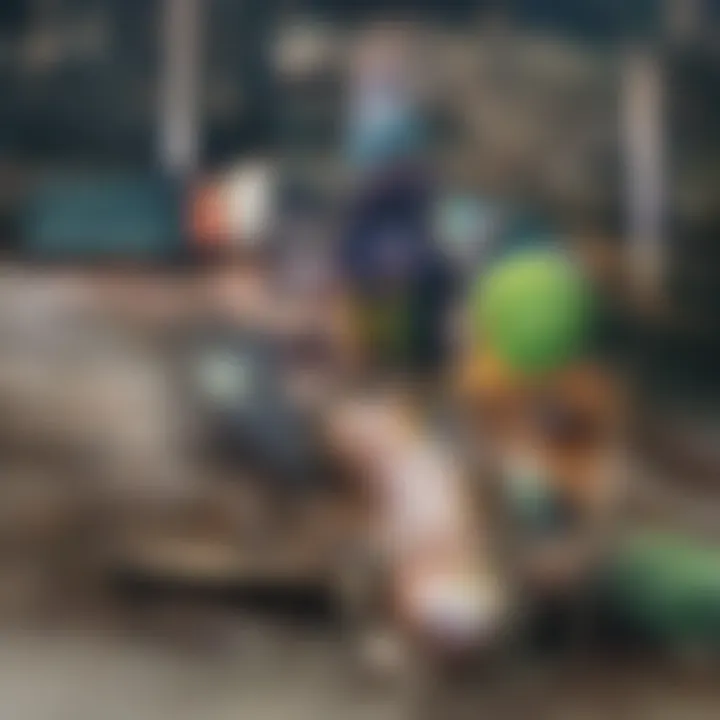
Organic chemistry provides the foundational principles required in materials science, especially in the development of polymers, nanomaterials, and other advanced materials. Materials made from organic compounds exhibit unique properties, such as flexibility, lightweight characteristics, and the potential for chemical modification. These materials find their uses in countless applications, from everyday products to cutting-edge technologies.
For instance, polymers like polyethylene and polystyrene are central to various industries, including packaging and construction. Their design depends heavily on understanding the underlying chemistry of polymer formation. Organic chemists work on synthesizing new materials that have improved performance or reduced environmental impact.
- Key roles of organic chemistry in materials science:
- Design and synthesis of new polymers.
- Analysis of polymer properties to improve performance.
- Development of bio-based materials to reduce reliance on fossil fuels.
Moreover, advancements in organic solar cells and organic light-emitting diodes (OLEDs) demonstrate how this interdisciplinary connection is paving the way for novel technologies that can change the industry landscape. By understanding the chemical interactions within materials, chemists can develop products that meet modern needs for sustainability and efficiency.
Role in Pharmaceutical Development
The intersection of organic chemistry and pharmaceutical development is one of the most impactful. This connection covers multiple facets including drug design, synthesis, and testing. Organic compounds are the heart of many drugs and understanding their chemistry allows scientists to design molecules that can interact selectively with biological systems.
The research in this area leads to the development of drugs with enhanced efficacy and reduced side effects. For example, the process of drug discovery often involves modifying organic compounds to improve their biological activity or pharmacokinetic properties.
"Through innovative chemical synthesis, the potential of organic chemistry in drug development is boundless."
Key contributions from organic chemistry to pharmaceutical development include:
- Synthetic pathways for drug creation.
- Identification of active pharmaceutical ingredients.
- Mechanism of actions studies to predict drug behavior.
Challenges in Organic Chemistry Research
Organic chemistry research faces a multitude of challenges that can hinder progress and innovation. Understanding these challenges is crucial for both researchers and practitioners in the field. By recognizing what these hurdles are, scientists can devise strategies to overcome them and enhance the impact of their work.
One significant concern in organic chemistry is sustainability. As the world grapples with climate change and resource depletion, the demand for environmentally friendly practices in chemical synthesis has never been more urgent. Traditional methods often rely on hazardous solvents and reagents that generate toxic waste. This not only raises safety issues but also poses serious environmental threats. Sustainable chemistry advocates prioritize the development of processes that use less hazardous materials, promote recyclability, and minimize waste. Researchers are exploring greener alternatives, such as using biomass or designing catalysts that can operate under milder conditions.
"The future of organic chemistry must align with the principles of sustainability to ensure that the field contributes positively to ecological preservation while advancing science and technology."
Emerging environmental issues also pose significant obstacles in organic chemistry research. The rapid pace of industrialization and population growth has led to increased pollution levels. Organic compounds, particularly volatile organic compounds (VOCs), contribute to air and water pollution. Researchers must navigate regulations and public concerns regarding health impacts associated with these substances. Efforts to analyze the behavior and fate of organic contaminants in the environment motivate the development of advanced analytical techniques. Enhanced methods enable the detection of trace levels of pollutants, facilitating better understanding and management of environmental risks.
Several strategies can be employed to address these challenges:
- Reassessment of synthesis pathways to identify areas for improvement
- Integration of multidisciplinary approaches to broaden solutions
- Collaboration between academia and industry to translate research findings into practice
Future Trends in Organic Chemistry
The field of organic chemistry is always adapting to advancements in science and technology. Understanding future trends is vital for students, researchers, manufacturers, and those in academia. These trends often shape the way organic compounds are synthesized, analyzed, and applied in daily life and industrial processes. Key areas to focus on include innovation in synthesis techniques and the integration of digital technologies in data analysis, promising significant benefits and new opportunities.
Innovation in Synthesis Techniques
Innovations in synthesis techniques are changing how organic chemistry is practiced. Traditional synthetic methods often have limitations in terms of efficiency and sustainability. New strategies are being embraced, such as green chemistry, which focuses on minimizing waste and energy consumption. Techniques like continuous flow synthesis allow for higher precision and better control over reaction conditions, leading to more efficient processes.
The emergence of biocatalysis is also notable. This approach leverages naturally occurring enzymes to catalyze reactions, providing an environmentally friendly alternative to chemical catalysts. Furthermore, the development of automated synthesis platforms enables rapid exploration of chemical space, which allows for faster discovery of new compounds.
"Embracing innovation in synthesis is not just an option; it is a necessity to meet the challenges of modern demands in organic chemistry."
Digitalization and Data Analysis
In our increasingly digital world, organic chemistry is not left behind. Digitalization and data analysis are proving transformative in the field. The rise of artificial intelligence and machine learning has created new possibilities in chemical research. By analyzing vast datasets, these technologies can predict reaction outcomes and identify new synthesis pathways much faster than traditional methods.
Computational chemistry tools are becoming more robust, enabling chemists to simulate and predict the behavior of molecules. This capability aids in hypothesis testing and reduces the need for extensive laboratory work. Sophisticated software applications can also help manage experimental data, offer insights into chemical identities, and streamline research processes.
The collaboration between data scientists and chemists will be essential for maximizing the benefits of these innovations. Creating interdisciplinary teams can lead to breakthroughs that were previously unattainable.
Ending
The examination of organic chemistry is vital for understanding the complexities of molecules that constitute life as we know it. This field encompasses various principles, methodologies, and applications that provide insights into the structure and behavior of organic compounds. In this concluding section, we reflect on where this article has taken us and the significance of the insights offered.
Summation of Key Insights
Throughout this article, we explored fundamental concepts such as bonding, functional groups, and isomerism. Each of these aspects plays a critical role in the behavior of organic molecules.
- Bonding and Structure: The way atoms connect significantly influences the properties of organic compounds.
- Functional Groups: These distinct groupings of atoms define chemical behavior and reactions.
- Reactions: Understanding various reactions, from addition to elimination, allows chemists to predict the outcomes of complex interactions.
- Applications: Beyond-theory discussions highlighted real-world usage, from pharmaceuticals to materials science.
The amalgamation of these insights shows that organic chemistry is not merely academic. It has practical implications that resonate across different sectors of our society. The integration of analytical techniques like spectroscopy and chromatography enables researchers to reveal details at the molecular level, further enriching our comprehension of material properties.
The Importance of Ongoing Research
Research in organic chemistry is crucial. As our societal needs evolve, the demand for innovative solutions grows. New challenges arise, particularly in sustainability and environmental concerns. Therefore, continuous investigation into organic compounds and their transformations remains essential. Here are some key considerations:
- Innovative Solutions: Discoveries in synthesis techniques can lead to more efficient production processes.
- Environmental Impact: Research can help mitigate risks associated with organic waste and pollution.
- Interdisciplinary Approach: Collaborations among chemists, biologists, and material scientists can open new pathways for development.