Exploring Electron Beam Crosslinking in Material Science
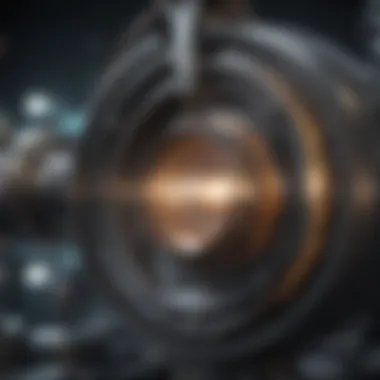
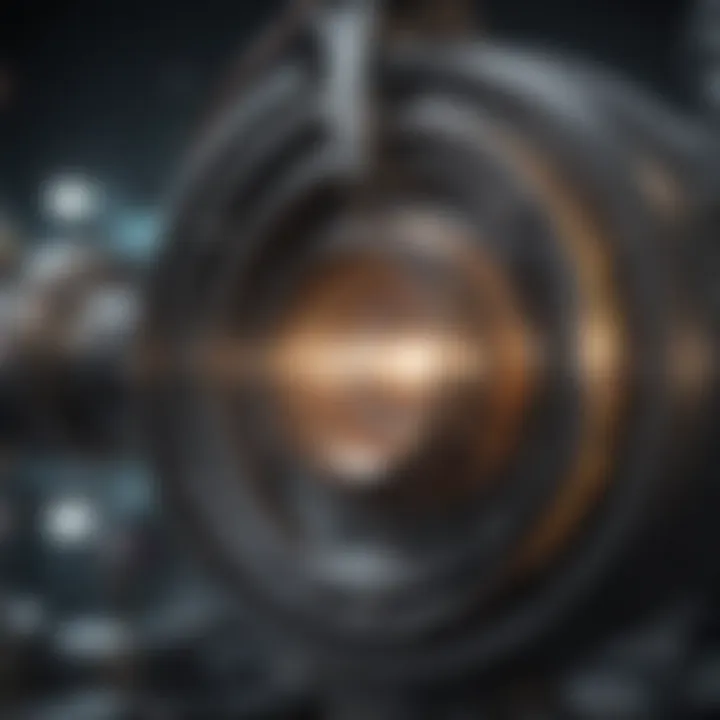
Intro
Electron beam crosslinking stands at the intersection of innovation and functionality in material science. It plays a crucial role in the improvement of polymer materials, enhancing their durability, chemical resistance, and overall performance. As industries increasingly strive for sustainable solutions, this technology emerges as a beacon of advancement, bringing forth possibilities that are both exciting and essential for future development. Understanding this science requires delving into its processes, applications, and significance in today’s world.
Research Overview
Key Findings
The study of electron beam crosslinking has unveiled several pivotal findings:
- Improved Mechanical Properties: Research indicates that polymers subjected to electron beam irradiation exhibit enhanced tensile strength and impact resistance. This can lead to applications in fields ranging from construction materials to biomedical devices.
- Environmental Benefits: Electron beam processes often reduce the need for chemical additives, thus minimizing the environmental footprint of producing or enhancing material properties.
- Diverse Applicability: Industries such as automotive, electronics, and packaging benefit immensely from crosslinked materials, which are more robust and versatile.
Study Methodology
The examination of electron beam crosslinking typically involves multifaceted techniques, including:
- Experimental Setup: Utilizing specifically designed electron accelerators to control the dose and energy of electron beams.
- Polymer Characterization: Techniques like Fourier-transform infrared spectroscopy (FTIR) and scanning electron microscopy (SEM) help in understanding molecular changes and polymer behavior post-irradiation.
Background and Context
Historical Background
The journey of electron beam crosslinking stretches back several decades, with its roots in the early research on radiation effects on polymers. Initially, methods employed for crosslinking were labor-intensive and relied heavily on chemicals. Over time, advancements in accelerator technology facilitated more accessible and effective irradiation techniques, giving rise to a new era in material science.
Current Trends in the Field
Today, electron beam crosslinking finds itself amidst rapid technological evolution and increasing environmental awareness. Some current trends include:
- Sustainable Manufacturing Practices: A growing emphasis is placed on processes that align with eco-friendly principles, leading to innovations that integrate electron beam crosslinking into circular economy models.
- 5G Applications: With the rise of the 5G network, electron beam-crosslinked materials are being developed to enhance electronic components' performance due to their superior thermal and mechanical stability.
"Around the world, scientists are exploring how this cutting-edge technology can revolutionize material production, blending ecological responsibility with groundbreaking performance."
Electron beam crosslinking is shifting paradigms in material properties and sustainability. Understanding its foundational aspects allows researchers and professionals to explore and harness its full potential effectively.
Prolusion to Electron Beam Crosslinking
Electron beam crosslinking stands as a crucial technology in the realm of materials science. The unique ability of electron beams to enhance the properties of polymers through radiation-induced crosslinking can't be overstated, for it opens doors to a spectrum of applications, from automotive components to medical devices. Understanding this technology is not just for material scientists; it impacts industries looking for stronger, more durable, and environmentally-responsible materials.
This method offers an efficient alternative to traditional chemical processes. It eliminates the need for solvents and toxic chemicals, which creates a compelling case for electron beam technology, especially in today's environmentally-conscious climate. In addition, it tends to improve the mechanical strength, thermal stability, and chemical resistance of polymers significantly.
Historical Context
The roots of electron beam technology can be traced back to the mid-20th century, when advancements in atomic physics began to intertwine with material science. Initially, the focus was primarily on radiation for sterilization and cancer treatment. However, researchers soon recognized its potential for enhancing material properties.
A notable advancement occurred in the 1960s, when scientists harnessed electron beams for the purpose of crosslinking polyethylene, which paved the way for its widespread application in various sectors. The technology has gradually evolved over the decades, growing in sophistication and efficiency. Companies and research institutions have since developed different electron beam systems, leading to more refined and controlled processes.
Economic factors also played a part in driving the development of this technology. As industries became attuned to the potential for increased production efficiency and material durability, investment in electron beam crosslinking technology surged during the late 20th century.
The Basics of Crosslinking
Crosslinking, in layman's terms, refers to the process of linking polymer chains together to form a more durable structure. Imagine cooking, where the ingredients meld together for a dish that is stronger and better in flavor than if they stood alone. The process can be a bit technical, but at its core, it alters the physical properties of materials, making them less prone to wear and tear.
In the context of electron beam crosslinking, the electron beams induce chemical reactions that create bonds between the polymer chains. When polymers are subjected to electron beams, high-energy electrons collide with atoms in the polymer, generating free radicals. These free radicals then react with nearby polymer chains, forming crosslinks.
The beauty of this method is its versatility. It can be applied to various materials, including elastomers, plastics, and even coatings. The degree of crosslinking achieved can be adjusted by tweaking operational parameters like beam energy and exposure time, enabling manufacturers to target specific material properties.
"Electron beam crosslinking offers a viable alternative to conventional crosslinking methods by enhancing material properties efficiently and sustainably."
In summary, electron beam crosslinking is far more than a mere technological curiosity. It's a game-changer that not only impacts the performance of materials but also aligns with sustainability goals by minimizing the use of harmful chemicals. Understanding its historical context and basic principles sets the stage for a deeper exploration of its mechanisms and applications.
Fundamental Principles of Electron Beams
Understanding the fundamental principles of electron beams is crucial to grasping how they function in crosslinking applications. The importance of these principles lies not only in explaining the science behind the technology but also in highlighting how effective electron beams can be in enhancing material properties. As we dive into this section, we will explore the nature of electron beams and the processes involved in generating them, both of which are critical to the success and efficiency of electron beam crosslinking.
Nature of Electron Beams
At their core, electron beams are streams of high-energy electrons that are generated and directed in a controlled manner. One of the most remarkable aspects of electron beams is how they can penetrate various materials, initiating chemical reactions at the molecular level. The energy carried by these electrons is what enables the crosslinking process to modify polymer properties significantly.
When electrons are accelerated, they can achieve kinetic energies that allow them to overcome the binding energy of electrons in atoms. As they collide with the target material, they may transfer energy that breaks chemical bonds, facilitating the formation of new linkages between polymer chains. This interaction is deeply rooted in the principles of radiation chemistry, emphasizing the need for understanding how both the physical properties of the beam and the chemical composition of the material influence crosslinking outcomes.
Several parameters define the nature of electron beams:
- Energy Levels: Varying energy levels determine how deeply the electron beam can penetrate materials.
- Current: The intensity of the beam affects dose rates; higher current means more electrons hitting the target per unit time, hence a more significant effect.
- Focus and Spot Size: Concentrating the beam can intensify the energy delivered to a specific area, while a broader spot allows for uniform treatment.
These parameters highlight that understanding the nature of electron beams isn't merely academic—it's necessary for effective application in industries like packaging, medical devices, and coatings.
Generation of Electron Beams
The process of generating electron beams generally involves several core components and technologies. Firstly, the generation usually requires devices known as electron guns, which create a controlled flow of electrons. One common type of electron gun is the thermionic emission gun that produces electrons by heating a filament, consequently enabling the emission.
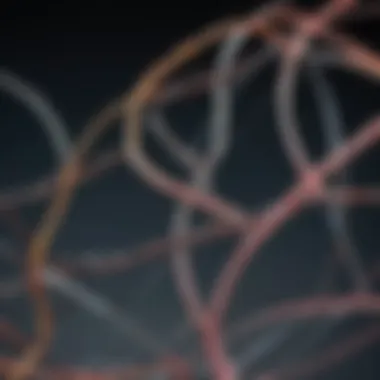
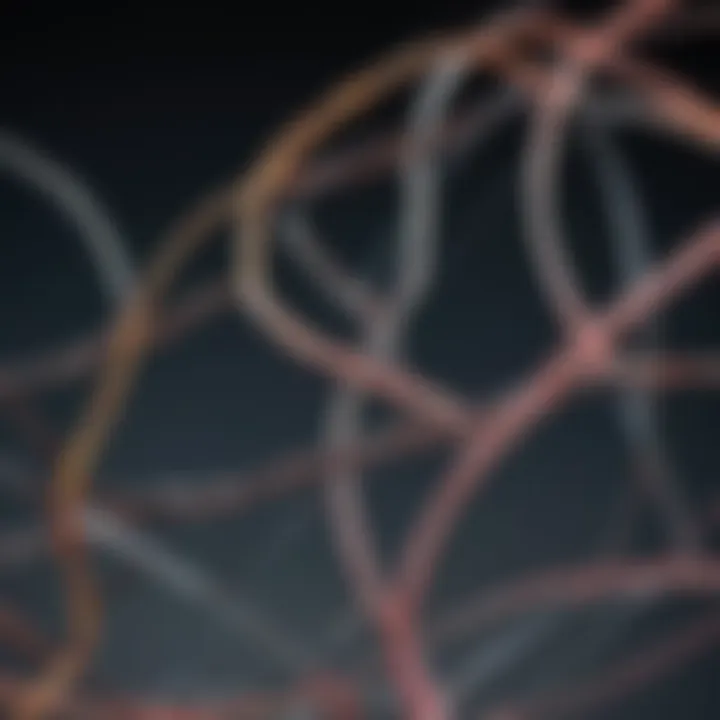
Another noteworthy method is the use of field emission guns, which operate by applying strong electrical fields to emit electrons. This technology is known for generating higher-current beams with finer resolution, making it particularly effective in scenarios requiring intricate crosslinking patterns.
The generation of electron beams also requires meticulous controls to manage the energy and current output, ensuring that the beams are stable during operation. Generally, the steps to generate electron beams are as follows:
- Heating: In thermionic guns, a filament is heated to emit electrons.
- Acceleration: Electrons are accelerated through a high-voltage potential, typically ranging from tens to several hundred kilovolts.
- Focusing and Deflection: Magnetic or electric fields are employed to focus the beam to the desired spot and direct it accurately.
The entire generation process is fundamental. The energy and precise control over the beam are crucial for successful crosslinking, directly impacting the properties of the finished materials. Overall, exploring the intricacies of how electron beams are generated deepens our understanding of how they can be applied effectively across various industries, thus enhancing material performance and sustainability.
Mechanisms of Crosslinking
Understanding the mechanisms of crosslinking is pivotal in grasping the full landscape of electron beam technology and its far-reaching effects in materials science. This section will delve deeply into the intricate processes that occur when electron beams interact with polymers, transforming them in unexpected and beneficial ways. By illuminating the fundamental radiation chemistry and the dynamic polymer chain reactions initiated during crosslinking, we can appreciate the technical prowess and innovative applications of this method.
Radiation Chemistry Underlying Crosslinking
The foundational layer of crosslinking lies in the principles of radiation chemistry. When polymers are exposed to electron beams, they endure a series of physical and chemical changes that are not merely superficial. The high-energy electrons are capable of knocking out electrons from the polymer chains, creating reactive free radicals. This initial reaction is quite a showstopper; these free radicals can then engage with neighboring polymer chains, leading to a web-like structure that enhances the material's properties.
Understanding the types of bonds formed during this process is crucial. Here’s a breakdown:
- Covalent Bonds: These are the strongest and form a stable linkage between polymer chains.
- Ionic Bonds: While not as strong, they can introduce flexibility into the material.
- Hydrogen Bonds: Although weak, their numbers can influence physical properties significantly.
The choice of dose rates and energy levels significantly affects the crosslinking efficiency. An optimal dose can create enough radicals without causing excessive chain scission, ensuring that the beneficial properties are maximized while minimizing degradation. It's crucial for scientists and engineers to fine-tune these parameters in various applications.
Polymer Chain Reactions
Once free radicals have formed, the next stage—polymer chain reactions—kicks into gear. This stage can be likened to a chain reaction, where one event triggers another, leading to a cascading series of reactions within the material. The key here is that these reactions can lead to significant alterations in the physical characteristics of the polymer, which is the crux of crosslinking.
In essence, as free radicals combine and link different polymer chains, a matrix of highly interconnected molecules emerges, enhancing the material’s strength and stability. Moreover, this interconnectedness typically leads to improvements in thermal and chemical resistance. For instance, in elastomers or plastics, a well-executed crosslinking process can result in:
- Improved Tensile Strength: A direct benefit of the molecular network created.
- Enhanced Elasticity: This is crucial for applications in items like tires and seals.
- Better Chemical Resistance: Good for items exposed to solvents or varying temperatures.
This interconnected network must be tailored to specific requirements, meaning different polymer compositions or conditions will yield varying results. Thus, a thorough understanding of chain reactions upon electron beam exposure stands as the backbone for innovations in material applications.
"The path from free radicals to robust materials is not just a technical necessity but a journey of molecular evolution that significantly enhances the functionality of polymers."
As scientists continue to explore this fascinating intersection of chemistry and materials science, they unlock not just new applications but also more efficient and sustainable practices. This comprehensive grasp of radiation chemistry and polymer chain reactions illustrates the transformative power of electron beam crosslinking in the ever-evolving landscape of polymer technology.
Equipment and Technology in Electron Beam Crosslinking
Understanding the equipment and technology involved in electron beam crosslinking is pivotal to grasping how this advanced technique enhances material performance. This section discusses the core components that define reliable crosslinking processes, emphasizing the significance of adopting efficient technologies in the industry. The advancements in electron beam systems not only directly impact performance but also the overall scalability of manufacturing processes, paving the way for versatile applications in various sectors.
Electron Beam Systems
Electron beam systems are the backbone of the crosslinking process, where finely focused beams of electrons bombard polymer structures. These systems consist of several integral parts:
- Electron Gun: This device generates high-energy electrons. Utilizing a heated filament or a field-emission source, it injects electrons into the acceleration structure. The efficiency of electron generation is crucial, as it influences the system's overall effectiveness.
- Accelerator Structures: Acceleration tubes are designed to impart substantial kinetic energy to electrons, enabling them to reach the desired energies necessary for inducing crosslinking. Two significant types are continuous wave and pulsed systems, each with particular use cases.
- Colimation and Focusing Equipment: Coils and magnets are employed to focus and direct the electron beam precisely. Proper collimation is essential as it not only ensures that the beam reaches the target but also minimizes scattering, enhancing precision in the crosslinking process.
Utilizing high-quality electron beam systems leads to more uniform crosslinking, which is essential for maintaining the integrity of materials. A poorly focused beam might result in uneven treatment, leading to quality inconsistencies in the final product.
"The success of any electron beam process largely hinges on the precision of the transport and focus of the electron beam system."
Furthermore, manufacturers must consider the scalability of the electron beam systems, as advances in technology support increased throughput. For instance, systems designed for larger scale applications can handle more extensive batches without compromising quality.
Instrumentation and Measurement
Instrumentation and measurement are critical for monitoring and controlling the electron beam crosslinking process. A suite of various tools plays an indispensable role in ensuring crosslinking efficiency and product quality:
- Dosimetry Systems: These devices measure the dose of radiation delivered during the crosslinking process. Accurate dosimetry is vital to ensure that polymers receive the optimal dose for desired crosslinking while avoiding under or over-exposure, which could adversely affect material properties.
- Temperature Monitoring: Temperature variations can impact the crosslinking reactions and are thus monitored closely. Temperature measurement devices must be integrated into the system to maintain optimal conditions throughout the process.
- Quality Control Sensors: Sensors that monitor surface and bulk properties in real-time ensure that the resultant materials meet required standards. By implementing automated measurement systems, inconsistencies can be promptly identified and rectified.
The right combination of instrumentation allows operators to fine-tune processes, adapt to different materials, and ensure consistent results.
In summary, understanding the equipment and technological landscape surrounding electron beam crosslinking reveals the complexity and the meticulous nature of the process. Investing in advanced electron beam systems and precise measurement instruments not only enhances the quality of crosslinked materials but significantly contributes to the overall efficiency of production.
Operational Parameters Affecting Crosslinking
Understanding the operational parameters affecting the electron beam crosslinking process is akin to tuning the strings of a fine instrument. Each setting, from dose rates to beam energy and exposure time, plays a crucial role in determining the effectiveness and efficiency of crosslinking. Variations in these parameters can significantly influence the properties of the final polymer material. When all elements are in harmony, the resultant materials boast improved durability, heat resistance, and flexibility.
Dose Rates
Dose rates represent a pivotal factor in the electron beam crosslinking process. Essentially, the dose rate refers to the amount of radiation delivered per unit of mass of the material being treated. For example, a higher dose rate can lead to more rapid crosslinking; however, too high a dosage might result in excessive degradation of the polymer chains, a bit like overcooking a fine meal.
Optimizing dose rates requires careful consideration. Each material type reacts differently under radiation exposure. For instance, elastomers may require a different approach compared to plastics. Understanding these nuances means researchers and manufacturers can tailor their processes to achieve the best material properties. Too low a dose won't give the desired crosslink density, leading to weak materials, while too high a dose may cause unwanted effects like embrittlement.
Highlights of dose rates include:
- Efficiency: Finding an optimal dose can maximize crosslinking while minimizing waste.
- Cost-effectiveness: Achieving high performance without overspending on energy or materials.
- Safety: Managing exposure levels to ensure worker safety and environmental protection.
Beam Energy and Exposure Time
Next, beam energy and exposure time are two intertwined elements that dictate the penetration depth of the electron beam into the material. Beam energy, measured in mega-electronvolts (MeV), affects the crosslinking development significantly. Higher beam energy allows deeper penetration, which can be beneficial for thicker materials but could also lead to uneven crosslinking if not controlled well.
Exposure time, on the other hand, is the duration for which the material is exposed to the beam. This is where timing becomes everything; too little time might not allow for sufficient crosslinking, while too much can lead to thermal effects that damage the material. In practice, timing and energy settings often interact, requiring operators to strike the right balance.
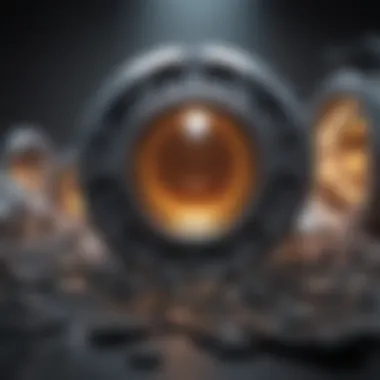
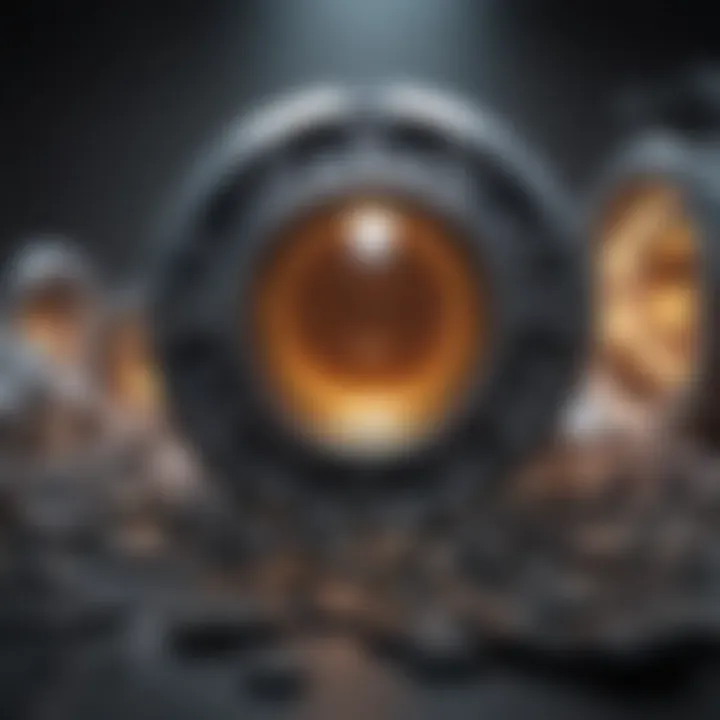
Key points to consider:
- Optimization: Different materials require different setups; testing is essential for fine-tuning.
- Uniformity: Ensuring consistent quality across the surface and throughout the thickness of the material.
- Application-Specific Needs: Different industries have varying requirements, from medical devices needing stringent certifications to automotive parts requiring durability under stress.
"The balance of beam energy and exposure time is critical; getting it right can yield materials that outperform traditional methods in the field of material science."
Applications in Material Science
The role of electron beam crosslinking within material science cannot be overstated. It's a transformative approach that boosts the performance of polymers, making them suitable for a myriad of applications across different industries. By enhancing physical properties and introducing new functionalities through crosslinking, materials can fulfill specific requirements suited for diverse environments. Moreover, this technique paves the way for sustainability, often using less energy compared to traditional methods.
One striking benefit of electron beam crosslinking is its ability to tailor material properties. Crosslinked polymers exhibit improved mechanical strength, chemical resistance, thermal stability, and elasticity. This is particularly relevant when considering the demands of modern industrial applications, where durability and reliability are paramount.
"Electron beam crosslinking allows for an unmatched level of customization in polymer science, enabling materials to meet the rigorous specifications of various sectors."
An essential consideration in adopting this technology is its versatility. From automotive parts to medical devices, the applications are as varied as they are impactful. But getting too comfortable with this technique can also present challenges such as economic implications and the necessity for comprehensive understanding of the material's compatibility with e-beam treatment.
Elastomers and Plastics
Elastomers and plastics represent prominent areas benefiting from electron beam crosslinking. The method enhances properties such as heat resistance and tensile strength, making these materials ideal for applications ranging from automotive seals to insulation in electrical wiring.
Unlike conventional methods where chemiclas are used to link chains, e-beam does this effortlessly through radiation. This reduction in additives not only improves the purity of the final products but also diminishes environmental concerns that arise with traditional crosslinking agents.
The economic viability of this technology also makes it appealing. Companies can produce lightweight yet robust components that stand the test of time. For instance, in the automotive sector, weight reduction is a key driver for improving fuel efficiency. Crosslinked plastics and elastomers are lighter and often fulfill strength and durability requirements without the need for heavy metal reinforcements.
Medical Devices and Souffles
In the sphere of medical devices, electron beam crosslinking plays an essential role. It facilitates the creation of biocompatible and sterilizable materials which are critical in medical applications. Crosslinking enhances the performance of polymers used in implants, catheters, and other medical tools, making them more suited to withstand bodily conditions.
The method also allows for the production of 'souffles'—thin, flexible sheets of crosslinked polymer used in dressings and other medical applications. These souflle products can be engineered for permeability and moisture management, which are vital for patient comfort and wound healing. Furthermore, the absence of harmful chemicals during the manufacturing process ensures a safer product for patients and healthcare providers alike.
Coatings and Adhesives
Lastly, coatings and adhesives benefit significantly from electron beam crosslinking. Enhanced surface properties lead to improved adhesion, which is crucial in industries ranging from construction to electronics. Coating materials treated with e-beams showcase better resistance to scratches, chemicals, and UV light damage.
Moreover, the ability to fine-tune these properties by adjusting the energy levels and the dose of electron beams further aids manufacturers in tailoring products to better meet the needs of their markets. In adhesives, crosslinking can enhance tackiness while ensuring the bond strength is ample for heavy-duty applications.
Advantages of Electron Beam Crosslinking
Electron beam crosslinking is a technique that sparks considerable interest, especially when evaluating the performance and durability of materials. The technology lays a solid foundation for numerous applications, fostering an avenue for materials that can withstand harsher environments. Let's delve deeper into the specific advantages this methodology provides.
Improved Material Properties
One of the most significant benefits of electron beam crosslinking is its ability to enhance material properties. By subjecting polymers to controlled doses of electron beams, a series of chemical reactions occur. This process links the polymer chains more tightly together, leading to a stronger and more resilient product. For instance, when elastomers are crosslinked through this method, the final material exhibits increased tensile strength, superior elasticity, and resistance to wear.
Characteristics like thermal stability and chemical resistance see substantial improvements as well. Polyethylene, for example, can become less susceptible to thermal degradation, which extends its lifespan considerably in demanding applications. Moreover, the crosslinking process can mitigate issues related to dimensional stability. Materials are less likely to deform under stress, making them ideal for demanding industrial applications. Therefore, companies looking to produce high-performance components may find electron beam crosslinking to be not just beneficial, but essential.
Environmental Benefits
Apart from material advantages, electron beam crosslinking also tantalizes with its environmental benefits. Given the increasing focus on sustainability, this technology provides a greener alternative compared to traditional methods of crosslinking, which often rely on harmful chemicals. Traditional crosslinking agents may generate toxic by-products, impacting both human health and the environment. In contrast, the electron beam process does not require such additives, making it a cleaner option.
This technology drastically reduces the carbon footprint associated with manufacturing processes. Because it accomplishes crosslinking without the need for heat, energy consumption is lower than many thermal processes. It also opens doors to recycling opportunities; materials treated with electron beams can sometimes be reprocessed without significant loss of properties.
The following points summarize key environmental considerations:
- Lower chemical usage: Less reliance on harmful substances
- Reduced energy requirements: Efficient processing with minimized thermal load
- Enhanced recyclability: Potential for reprocessing without compromising quality
"Sustainable practices not only cater to eco-conscious consumers but also enhance a business's market competitiveness."
In a world increasingly driven by the need to adopt sustainable practices, electron beam crosslinking proves to be a relevant choice. As this field continues to advance, the advantages—both in material performance and ecological impact—underscore its promising role in material science.
Limitations and Challenges
Examining the limitations and challenges of electron beam crosslinking is crucial for grasping its complete framework and how it fits within the broader scope of material science. Despite its remarkable advantages, such as improved material properties and environmental benefits, there are factors that can impede its widespread adoption and effective implementation. Let's explore these elements in detail.
Economic Considerations
When it comes to the economic aspects, electron beam crosslinking poses some significant financial hurdles. The initial investment required for electron beam equipment is not small potatoes. These systems can run into hundreds of thousands, if not millions, depending on the scale and capabilities. This high upfront cost can act as a deterrent for many smaller enterprises or research teams with tighter budgets.
It's also necessary to weigh the operational costs. Unlike some traditional crosslinking methods which utilize chemicals or heat, the energy consumption for electron beam technology does not come cheaply, leading to an increased ongoing expenditure. The complexity of the technology can demand specialized training for personnel, making labor costs another important factor to consider.
Additionally, while electron beam crosslinking can result in high-quality materials, its economic viability often comes down to scale. In smaller productions, the cost per unit can skyrocket in comparison to chemical crosslinking methods, potentially off-setting the quality gains. In many cases, it has been seen that companies evaluate their return on investment carefully before committing to electron beam technologies.
"A well-rounded understanding of costs is essential when evaluating new technologies in production settings."
Material Compatibility
Material compatibility presents another layer of challenges with electron beam crosslinking. Not all polymers react favorably to electron beams, and this could mean limiting the range of materials that can be effectively processed using this technique. For example, certain soft thermoplastics may not achieve the desired crosslinked network structure, reducing the enhancements in material performance that make electron beam technologies appealing.
Moreover, additives and fillers present in some polymers might absorb the electron energy unevenly. This could lead to inconsistent crosslinking and variable material properties across the batch. Consequently, thorough pre-testing and optimization are necessary to ensure that both the base polymer and any incorporating additives stand up to the rigors of the electron beam process.
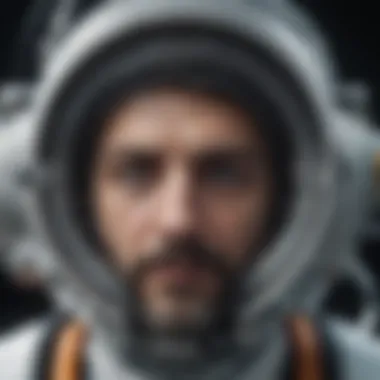
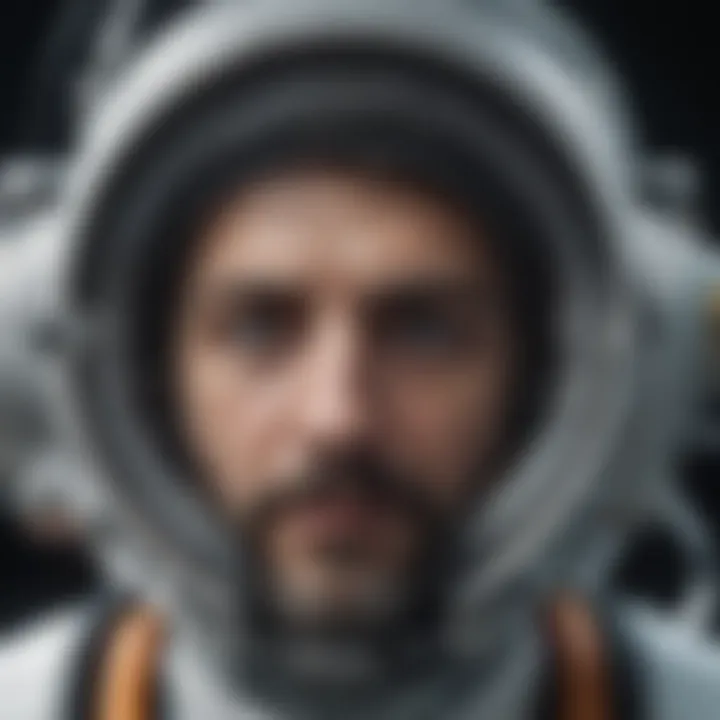
In some cases, the presence of moisture in materials can lead to complications during the crosslinking process. The electron beam can cause unwanted reactions if water is present, leading to degradation and other adverse effects on the final product.
Understanding these compatibility issues allows scientists and engineers to better design materials that are more amenable to electron beam processing, fostering advancements in this field.
Comparative Analysis with Other Crosslinking Techniques
Understanding the various crosslinking techniques is crucial for appreciating the unique position occupied by electron beam crosslinking in the vast landscape of material science. Crosslinking isn't merely a method of enhancing the properties of polymers; it's a significant factor in determining the performance, longevity, and environmental impact of materials across many applications. Thus, a comparative analysis sheds light on the distinct strengths and weaknesses of electron beam technology contrasted against others, which is essential for informed decision-making in research and industry.
Chemical vs. Physical Crosslinking
Chemical crosslinking methods typically involve the introduction of reactive chemical agents that facilitate the linking of polymer chains. Examples of these agents include peroxides, isocyanates, or acids. Upon exposure, they initiate a series of reactions that effectively ‘stick’ polymer molecules together through covalent bonds. While this method can be highly effective, it also raises concerns regarding toxicity and environmental impact. Materials treated with chemical crosslinkers may require additional processing steps to eliminate hazardous residues.
In contrast, physical crosslinking relies on non-covalent interactions such as hydrogen bonding, Van der Waals forces, or ionic interactions. While these physical methods are often safer and more environmentally friendly, the ultimate performance of the resulting materials may be less reliable as these interactions can be influenced by temperature and solvent conditions, leading to potential degradation over time.
Comparatively, electron beam crosslinking presents a compelling case. It does not require the use of additional chemicals, thus eliminating residual toxic substances. Furthermore, it enables precise control over crosslinking density, which can be tailored for specific applications. It’s worth noting that the speed of crosslinking, often an issue with physical methods, is significantly improved due to the instantaneous nature of electron beam technology. This Fast and reliable response positions electron beam crosslinking strongly in discussions of efficient manufacturing processes.
Heat-Induced Crosslinking
Heat-induced crosslinking is another common method involving the application of heat to promote polymer chain interactions and subsequent linking. This traditional approach, however, is not without its kinks. For instance, overheating can lead to material degradation or deformation, fundamentally compromising the integrity of the product. Moreover, the energy consumption associated with extended heating cycles may negatively impact the overall sustainability metrics of the processes, particularly in industries that are strongly leaning towards green methodologies.
On the other hand, electron beam crosslinking sidesteps many of these hurdles. The process is achieved at room temperature, significantly reducing the risk of thermal-induced damage. The high energy density of electron beams allows for a quick, often single-pass treatment, minimizing energy use and maintaining the mechanical properties of materials throughout the procedure. It’s especially noteworthy that electron beam crosslinking can address limitations common to heat-induced processes, making it viable for heat-sensitive materials.
In summary, viewing electron beam crosslinking through the prism of other techniques reveals its multifaceted advantages. This includes safety from toxic chemicals, the ability to fine-tune material properties swiftly, and a reduction in energy waste during production. As researchers and professionals continue to explore the potential of various methods, electron beam technology’s unique benefits position it as a forefront player in the development of advanced materials.
Current Research Trends
The relevance of current research trends in electron beam crosslinking cannot be overstated. As industries strive for improved efficiency and performance in polymer materials, the role of electron beams is increasingly critical. This section delves into innovations in beam technology and emerging applications that highlight the evolving landscape of electron beam crosslinking. Research trends not only illuminate advances but also address various challenges that can arise. The findings contribute significantly to optimizing processes and expanding the scope of applications, ultimately impacting sustainability and material science.
Innovations in Beam Technology
Recent advancements in electron beam technology have been nothing short of revolutionary. One of the key areas of innovation is the development of miniaturized electron beam accelerators. These compact devices promise to enhance accessibility in various settings, allowing smaller businesses to adopt this technology and reaping its benefits without substantial capital investment.
Moreover, high-throughput systems have emerged, enabling faster processing times for crosslinking applications. This improvement paves the way towards more efficient production lines, significantly reducing downtime and increasing output. Furthermore, there’s growing interest in real-time monitoring techniques that help in ensuring the consistency and effectiveness of the crosslinking process. By integrating sensors and smart technology, researchers can gain precise insights into the interactions happening within the material as it is being processed.
These innovations illustrate the shift towards automation and smart integration, demonstrating how new technologies can enhance the traditional electron beam process. For instance, using machine learning algorithms to analyze data from the crosslinking process can lead to better material formulations and predict outcomes with greater accuracy.
Emerging Applications
Emerging applications for electron beam crosslinking are vastly diverse, showcasing the versatility of the technology. One significant area of interest is bioengineering. Here, electron beam crosslinking is being explored for its potential in creating biocompatible materials that are essential for medical devices. The ability to enhance the properties of polymeric materials through radiation can lead to significant advancements in surgical implants and drug delivery systems.
Additionally, in the realm of renewable energy, electron beam crosslinking is being investigated for applications in creating efficient photovoltaic devices. By optimizing polymeric materials used in solar panels, efficiency and durability can be considerably improved, contributing to smarter energy solutions.
Lastly, another exciting avenue lies in eco-friendly packaging. As the industry pivots toward sustainable practices, the capacity of electron beams to modify polymer structures efficiently allows for the production of biodegradable materials that maintain durability without compromising environmental standards.
"Current research trends in electron beam applications indicate a clear pivot toward sustainability, efficiency, and adaptability across various industries."
Through ongoing investigations and innovations in these sectors, the trajectory of electron beam crosslinking continues to evolve, pointing toward a future that promises enhanced materials with greater functionality and ecological responsibility.
Future Perspectives
Understanding the future of electron beam crosslinking is essential for researchers and professionals aiming to remain at the forefront of material science. The technology's evolution intertwines with changing industrial demands and environmental considerations, thus shaping its trajectory. Enhanced processing methods, combined with sustainable practices, hold the potential to revolutionize how industries utilize this innovative technique.
Integrating Sustainability Practices
Sustainability in technology is no longer just a buzzword; it’s a necessity. Electron beam crosslinking, with its unique ability to crosslink polymers efficiently, presents a golden opportunity for integrating sustainable practices. This process significantly reduces the use of harmful chemicals often required in traditional methods. For instance, instead of using solvent-based systems that can be detrimental to both health and the environment, electron beam technology can operate without them, leading to a smaller environmental footprint overall.
The emphasis on reducing emissions in production processes aligns perfectly with the benefits of electron beam crosslinking. Not only does it limit volatile organic compounds (VOCs) in emissions, but it also enhances the recyclability of materials by ensuring they maintain their integrity better through various processes.
However, the success of integrating sustainability practices relies on collaboration among various stakeholders. Researchers need to work closely with industry leaders to pivot towards these greener practices. If stakeholders can establish standards and guidelines, electron beam technology could take a step toward broader acceptance in industries such as packaging, automotive, and construction. There’s plenty of work to be done, yet the potential gains are substantial.
Expanding Industrial Applications
The horizons of electron beam crosslinking are broadening as industries begin to understand its versatility. Beyond traditional applications in polymers, emerging fields continuously seek innovative solutions. For instance, in the energy sector, crosslinked polymers are becoming indispensable for applications such as cable insulation and components in renewable energy technologies.
Another area with significant promise lies within the realm of medical applications. As healthcare demands rise, there’s growing interest in developing biocompatible materials using electron beam techniques. This could potentially lead to advancements in implants and drug delivery systems that utilize crosslinked polymers which are not only reliable but also safe for human use.
Furthermore, as industries push toward fully automated processes, electron beam crosslinking systems have the potential to be integrated into automated production lines, lending efficiencies not currently present in traditional crosslinking methods.
Interestingly, the incorporation of this technology spans well into emerging sectors like nanotechnology, where crosslinked polymers might facilitate the development of advanced materials for electronics or supercapacitors that require high durability and performance.
Culmination
The closing discussion of this article encapsulates the multifaceted significance of electron beam crosslinking within the realm of material science. As we traverse the landscape of polymer technology and sustainability, this method stands as a beacon of innovation, offering remarkable advancements in material properties. The understanding we have gained about the operational parameters, benefits, and challenges of this technique provides valuable insight for both academia and industry.
Summarizing Key Insights
At the heart of this exploration lies a few fundamental observations regarding electron beam crosslinking:
- Efficiency in Modifying Polymer Structures: Electron beam crosslinking has proven efficient at enhancing the physical and chemical properties of polymers. This is particularly evident in the durability and strength of materials, which find applications across diverse fields, from medical devices to automotive components.
- Reduction of Harmful Solvents: Unlike traditional chemical crosslinking methods, electron beam technology minimizes or eliminates the need for harmful solvents, presenting notable environmental advantages.
- Rapid Processing: The speed at which electron beams can induce crosslinking allows for quicker production cycles, which is particularly appealing in mass manufacturing settings.
- Emerging Research Trends: Recent innovations in beam technology suggest a breadth of future applications, widening the horizons for current and future research endeavors.
These insights underscore the importance of electron beam crosslinking—not just in terms of material enhancement but also its potential for driving sustainable practices in manufacturing.
The Road Ahead for Electron Beam Technology
Looking toward the future, several pivotal pathways seem promising for the ongoing advancement of electron beam technology:
- Integration with Other Technologies: There is potential for hybrid methodologies, merging electron beam crosslinking with other processing techniques for enhanced functionality and performance of materials.
- Broader Industrial Adoption: As industries continue to emphasize sustainability, electron beam crosslinking could become a standard practice, especially in sectors that prioritize eco-friendly processes.
- Innovations in Electron Beam Equipment: The evolution of more compact and cost-effective electron beam systems will likely stimulate wider usage, making the technology accessible to smaller enterprises and research laboratories.
- Research on New Polymers: Future research is likely to unveil new polymers that are better suited for electron beam crosslinking, expanding the material palette and potential applications.