Exploring the Evolution of Genome Sequencing Technology
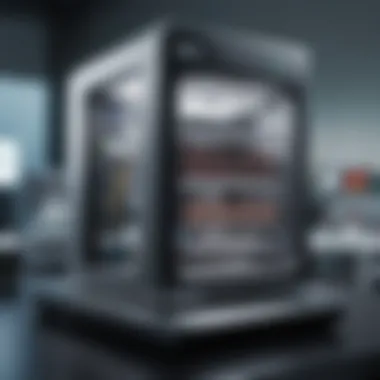
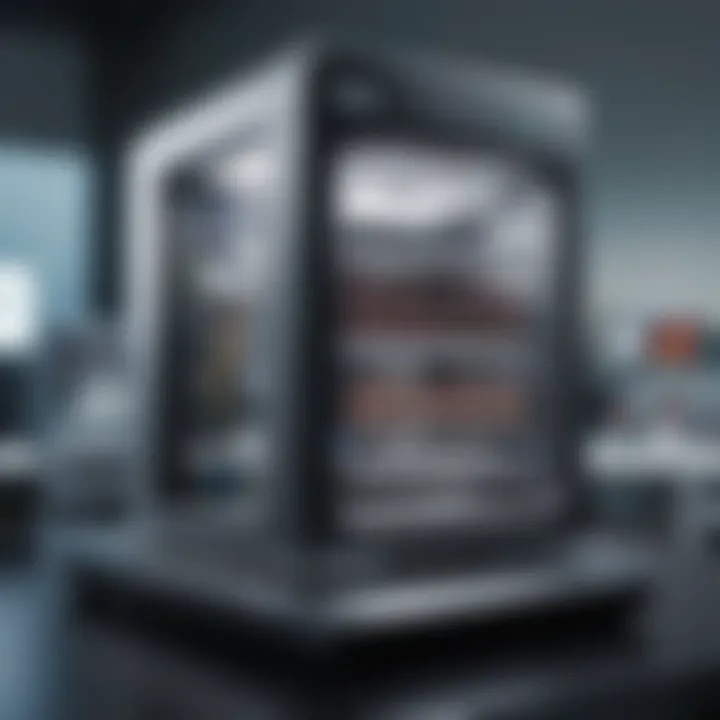
Intro
Genome sequencing machines represent a revolution in how we understand and interact with biological systems. The technology behind them has evolved significantly over the years, driven by advances in genomics and bioinformatics. These machines enable scientists to decode the entire genetic makeup of organisms, from the simplest bacteria to complex human genomes. This capability has profound implications in areas like medicine, agriculture, and fundamental biological research.
The goal of this article is to provide a thorough exploration of genome sequencing machines. By delving into their development, applications, and significance, we aim to present a comprehensive overview. The following sections will guide readers through the historical context of genome sequencing, current innovations, methodologies, and future trajectories of this essential technology.
Prologue to Genome Sequencing Machines
Genomic sequencing machines are crucial instruments in modern molecular biology and genetics. They enable the detailed analysis of an organism’s genome, which is the complete set of its DNA, including all its genes. Understanding these machines is vital as they transform our approach to health care, agriculture, and environmental science. The rapid advancements in this field have created a pressing need to delve into the mechanisms and implications of genome sequencing technology.
Defining Genome Sequencing
Genome sequencing refers to the process of determining the complete DNA sequence of an organism's genome, including all of its genes. This process can be performed using various methods, each suited to different applications and research questions.
There are two major types of sequencing approaches: short-read sequencing and long-read sequencing. Short-read sequencing involves reading small fragments of DNA, while long-read sequencing can sequence longer segments, providing more context regarding structural variations in the genome.
In essence, genome sequencing machines facilitate the precise capture of genetic information, which can be utilized for further analysis and interpretation.
The Importance of Genome Sequencing
The significance of genome sequencing lies in its various applications in research and medicine.
- Medical Diagnostics: Genome sequencing plays a crucial role in diagnosing genetic disorders and allows precision medicine, where treatment can be tailored to an individual's genetic makeup.
- Research Advancements: In research, genome sequencing enables scientists to understand the genetic basis of diseases, evolutionary biology, and population genetics.
- Agricultural Improvements: In agriculture, it supports the development of genetically modified organisms that can withstand environmental pressures and improve food security.
Genome sequencing machines stand at the forefront of the genomic revolution, pushing the boundaries of what is possible in research and application. Their impact is widespread, affecting fields from oncology to environmental restoration, which highlights the necessity for a nuanced understanding of these instruments.
History of Genome Sequencing Technology
Understanding the history of genome sequencing technology is crucial for comprehending its current applications and future potential. This narrative unfolds the evolution of methods that have revolutionized genetic research and interdisciplinary sciences. The historical context not only informs on technological milestones but also illuminates the ongoing challenges and breakthroughs that are pivotal for genetic research today. By analyzing past developments, we can appreciate the significance of genome sequencing in the advancement of personalized medicine, biotechnology, and our understanding of complex diseases.
Initial Techniques
The earliest techniques for genome sequencing emerged in the 1970s. Sanger sequencing, developed by Frederick Sanger and his team, marked a significant leap in genetic analysis. This method, referred to as the chain-termination method, enabled researchers to determine the nucleotide sequences in DNA with unprecedented accuracy. The ability to sequence individual genes paved the way for advances in molecular biology. While Sanger's method was powerful, it was also labor-intensive and time-consuming.
Moreover, Capillary electrophoresis further improved the Sanger method. It enhanced the separation of DNA fragments based on size, allowing for higher throughput. Despite its limitations, this foundational technique laid the groundwork for future innovations in sequencing technologies and showcased the importance of accuracy in genomic research.
The Human Genome Project
The Human Genome Project (HGP), initiated in 1990 and completed in 2003, was a global endeavor that aimed to map the entire human genome. This monumental project involved scientists from various countries and disciplines. The HGP provided a reference for sequencing and analyzing human DNA, opening pathways for various applications in medicine and biology.
One of the remarkable outcomes of the HGP was the identification of approximately 20,000-25,000 protein-coding genes and millions of disease-related mutations. The data produced by the HGP has since become a resource for researchers worldwide, facilitating studies in genetics, evolutionary biology, and medicine.
The impact of the Human Genome Project is evident in personalized medicine approaches, where genomic information informs treatment decisions tailored to individual patients. As a result, the HGP stands as a landmark achievement, illustrating the power of organized scientific efforts in unlocking the complexities of human biology.
Types of Genome Sequencing Machines
Understanding the different types of genome sequencing machines is crucial for appreciating their diverse applications and potential impact in various fields. Each type offers unique methodologies, strengths, and setbacks, influencing decisions in research and clinical practices. This article explores Sanger Sequencing, Next-Generation Sequencing (NGS), and Third-Generation Sequencing. These technologies serve as pillars in genomics and have transformed how researchers approach genetic analysis.
Sanger Sequencing
Sanger Sequencing, named after Frederick Sanger, is one of the earliest and still widely used sequencing methods. It primarily relies on chain-terminating inhibitors known as dideoxynucleotides. The process begins with the amplification of the DNA sample using polymerase chain reaction (PCR). In this method, a single strand of DNA is synthesized, making use of ddNTPs, which terminate DNA elongation. The resulting fragments are then sorted by size through capillary electrophoresis.
This technique provides high accuracy and allows for sequencing relatively short regions of DNA, typically up to 1000 base pairs. Some of its significant advantages include:
- High accuracy: Sanger sequencing boasts error rates lower than 0.1%, making it reliable for precise applications.
- Gold standard: It is often used for validation of NGS results due to its reliability.
- Less computationally demanding: The output of Sanger sequencing is less complex, requiring less computational power compared to some NGS methods.
However, Sanger Sequencing does have its limitations, particularly in scalability. It can be time-consuming and costly when sequencing large genomes or when targeting numerous samples, often making it less ideal for high-throughput requirements.
Next-Generation Sequencing (NGS)
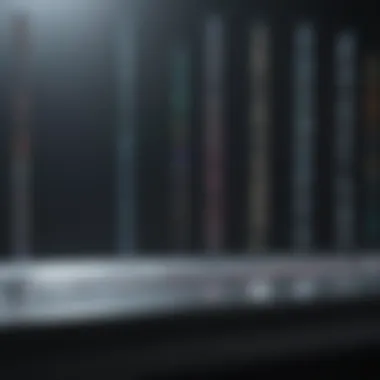
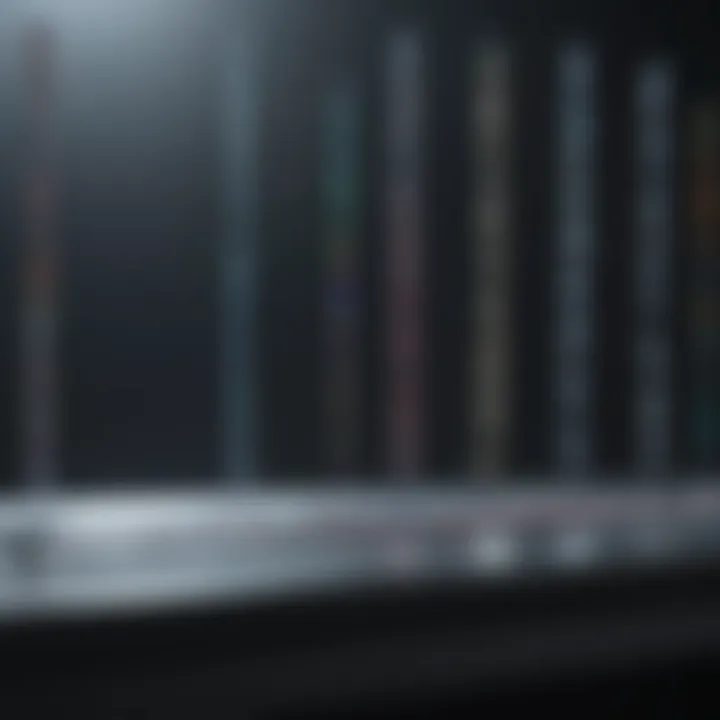
Next-Generation Sequencing revolutionized genomic research with its capacity to sequence millions of fragments simultaneously. NGS enables researchers to conduct whole-genome sequencing, targeted resequencing, and transcriptome analysis efficiently and cost-effectively. Key components of NGS include:
- Massive parallel processing: Multiple DNA fragments are sequenced at once, drastically increasing throughput.
- Short reads: Typically, NGS produces short reads, which may range from 50 to 600 base pairs, depending on the platform used.
The technology has several advantages, such as:
- Cost-effectiveness: It provides lower cost per base compared to Sanger sequencing for large datasets.
- Speed: NGS can sequence an entire human genome in a matter of days.
- Diverse applications: From epigenomics to metagenomics, NGS serves various fields of research, making it a versatile tool.
Despite its advantages, NGS is not without challenges. The data generated is enormous, requiring substantial storage and processing capabilities. Additionally, its error rate can be higher than that of Sanger sequencing, particularly in homopolymeric regions. These factors necessitate careful analysis and validation of results.
Third-Generation Sequencing
Third-Generation Sequencing encompasses newer technologies designed to sequence long DNA molecules in real-time. Notable platforms include Pacific Biosciences' SMRT sequencing and Oxford Nanopore Technologies. These methods allow reads that can reach several kilobases, making them valuable for resolving complex genomic regions. Key features of Third-Generation Sequencing include:
- Real-time sequencing: Data can be analyzed as it is generated, reducing time from sequencing to results.
- Long reads: Extended read lengths facilitate the assembly of repetitive regions in genomes, as well as improve variant detection.
The benefits of this technology bring several advantages:
- Improved assembly accuracy: Long reads help produce complete and accurate representations of genomic sequences.
- Enhanced variant detection: The ability to detect structural variants is significantly improved with long reads.
However, challenges exist. Third-Generation Sequencing often has higher error rates than Sanger or NGS methods. This necessitates further advancements in error correction techniques and the development of more robust platforms.
Understanding these various types of genome sequencing technologies equips researchers and industry professionals with the knowledge needed to choose the appropriate method for their specific applications. As these technologies continue to evolve, they promise to deepen our understanding of genetics and its applications across several domains.
The Working Mechanism of Genome Sequencers
Understanding the working mechanisms of genome sequencers is integral to grasping their overall functionality and significance in modern science. Genome sequencers do not merely run sequences; they embody complex methodologies that enable researchers to decode vast stretches of genetic information. This section will assess the key steps involved in the sequencing process, including sample preparation, the actual sequencing chemistry, and ultimately data analysis. Each component contributes to the accuracy and efficacy of genomic analysis, which is vital for various applications, from medical research to agricultural improvements.
Sample Preparation and DNA Extraction
Sample preparation is one of the first and most crucial steps in the genome sequencing process. This involves isolating the DNA from the biological samples which can come from numerous sources such as blood, saliva, or tissue. The quality and purity of the extracted DNA significantly influence the sequencing output.
Typically, a series of chemical processes are utilized for extraction. These processes include cell lysis to break down cell membranes, followed by removal of proteins and other contaminants, allowing only the genomic material to remain.
Moreover, qualitative checks are mandatory at this stage to ensure the DNA is suitable for subsequent procedures. Techniques like gel electrophoresis can visually confirm the presence and size of DNA fragments. A well-executed sample preparation enhances the reliability of the entire sequencing endeavor, ensuring fidelity of results.
Sequencing Chemistry and Processes
Once the samples are prepared, the next phase is actual sequencing. This can differ based on the technology being utilized, such as Sanger Sequencing or Next-Generation Sequencing (NGS).
In NGS, DNA fragments undergo a process called amplification, where they are copied to produce millions of identical pieces. This is typically achieved through polymerase chain reaction (PCR) or bridge amplification. During sequencing, the DNA is read as its nucleotides are incorporated.
The chemistry of this process often employs fluorescent tags or reversible terminator bases that allow for real-time analysis of incorporated bases, effectively translating genetic information into a readable format.
This step is decisive because precision during sequencing directly correlates to the accuracy of downstream applications such as variant calling and structural variant analysis.
Data Analysis and Interpretation
The final stage in the genome sequencing process is data analysis. Raw sequencing data must undergo extensive processing to derive meaningful insights. This includes quality control measures and filtering steps to eliminate errors and low-quality reads.
Once the data is cleaned, bioinformatics software comes into play. Algorithms align the sequences with a reference genome, allowing researchers to identify variants, mutations, and other significant phenomena.
Data analysis not only requires computational power but also a nuanced understanding of genetics to make appropriate interpretations. The final results can inform a range of applications, from personalized medicine strategies to evolutionary biology studies.
"The working mechanism behind genome sequencers is what empowers scientists to unlock the secrets of life encoded in DNA, paving the path for breakthroughs in research and medicine."
In summary, the working mechanisms of genome sequencers represent an intricate, multi-step process that starts from sample preparation, progresses through sequencing chemistry, and culminates in data analysis. Each phase holds its unique challenges and requirements, fostering advancements in the biotechnology field.
Applications of Genome Sequencing Machines
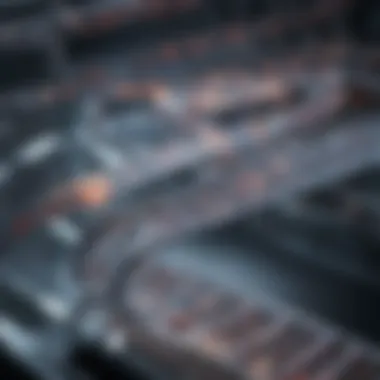
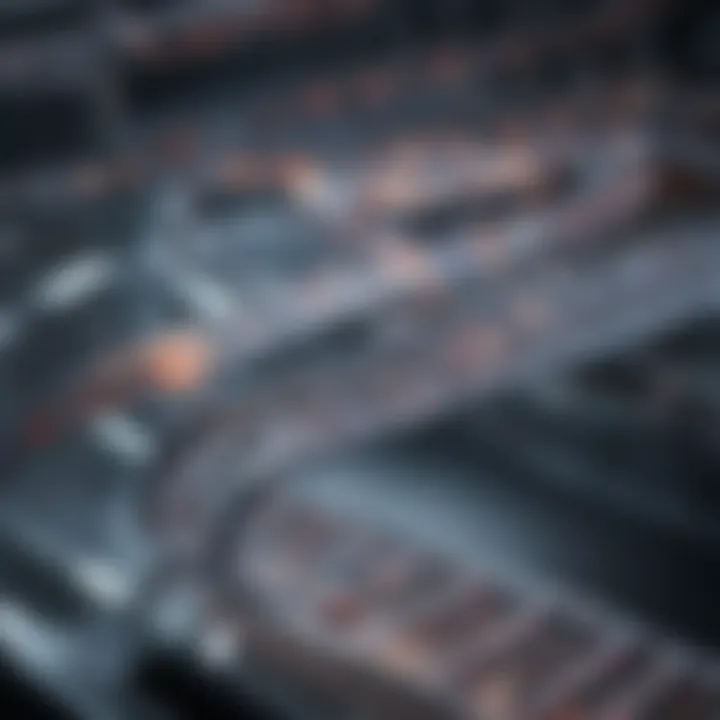
The applications of genome sequencing machines reflect their profound impact across multiple domains, particularly in the realms of healthcare, agriculture, and scientific discovery. These applications are not mere technological advancements; they represent a paradigm shift towards a future where genomic data drives decision-making in medicine, farming, and beyond. Understanding these applications is critical for recognizing the multifaceted benefits they bring to society and research.
Biomedical Research
In biomedical research, genome sequencing machines serve as essential tools for unlocking the complexities of human genetics. By sequencing genomes, researchers can identify genetic mutations linked to various diseases. This capability has fueled advancements in understanding hereditary conditions, cancers, and other complex disorders.
Genome sequencing has enabled significant breakthroughs in identifying disease-associated genes. For instance, the study of the BRCA1 and BRCA2 genes illustrates how sequencing can reveal susceptibility to breast and ovarian cancers, guiding preventative measures and treatments. Furthermore, researchers utilize this technology to explore population genomics, understanding how genetic diversity influences health outcomes across different demographics.
Quote: "The ability to sequence a genome within hours, rather than years, opens new possibilities for real-time genetic analysis in research and clinical settings."
Moreover, genome sequencing machines facilitate the study of rare diseases. By sequencing the genomes of affected individuals, researchers can discover novel genetic variants, paving the way for targeted therapies. This application not only enhances our understanding of diseases but also accelerates the development of precision medicine.
Personalized Medicine
The concept of personalized medicine, often referred to as precision medicine, relies heavily on genome sequencing machines. This approach tailors medical treatment to the unique genetic composition of an individual. By analyzing a patient’s genome, healthcare providers can design customized treatment plans that are more effective and have fewer side effects.
For example, in oncology, genome sequencing helps to identify specific mutations within a tumor's DNA. This information enables oncologists to select therapies that specifically target those mutations. As a result, patients receive treatment that is more likely to be effective compared to traditional one-size-fits-all approaches.
Additionally, pharmacogenomics, a branch of personalized medicine, investigates how genes affect a person’s response to drugs. This knowledge allows for the optimization of drug choices and dosages for individual patients, minimizing adverse reactions and enhancing efficacy.
The economic implications of personalized medicine are also noteworthy. Reducing trial-and-error in treatments can lead to cost savings for both patients and healthcare systems. This application shows that genome sequencing is not only a scientific tool but also an important economic driver in healthcare.
Agricultural Biotechnology
In agricultural biotechnology, genome sequencing machines play a vital role in enhancing crop yields and resilience. These machines allow scientists to analyze the genomes of plants, identifying traits related to growth, disease resistance, and environmental adaptation. This knowledge is critical as global populations rise and climate change poses new challenges to food security.
By sequencing the genomes of staple crops like rice, maize, and wheat, researchers can develop genetically modified organisms (GMOs) that are more productive and durable against pests and diseases. Genomics also facilitates marker-assisted breeding, where specific genetic markers linked to desirable traits are used to improve plant breeding programs.
Furthermore, livestock breeding benefits similarly. Genome sequencing aids in selecting animals with traits for improved health, growth rates, and productivity. The integration of genetic data into breeding programs accelerates genetic gains, ensuring sustainable agricultural practices.
Limitations and Challenges
The landscape of genome sequencing technology, while groundbreaking, is not without its obstacles. Understanding the limitations and challenges of genome sequencing machines is crucial for appreciating their current capabilities and future potential. This section aims to illuminate the key aspects that constrain the technology while also highlighting the ethical considerations and data privacy issues that arise as a result of advancements in this field. The implications of these limitations affect not only researchers and medical professionals but also the patients and communities involved in genomic studies.
Technical Limitations
Genome sequencing technology has seen rapid developments, yet there are inherent technical limitations that affect the quality and accuracy of sequencing results. One primary concern is accuracy. Even advanced methods such as Next-Generation Sequencing (NGS) can produce errors in base calling, leading to incorrect interpretations. This can affect subsequent analyses and conclusions drawn from the data.
Another issue is the coverage and depth of sequencing. Insufficient coverage can result in missed variants, particularly in regions of the genome that are difficult to sequence, such as repetitive sequences. Variations may also go unnoticed in lower coverage samples, compromising the reliability of findings.
Additionally, the cost of sequencing machines and reagents remains significant. While the cost per base has decreased dramatically over the years, initial investment in these technologies can be prohibitive, especially for smaller laboratories or institutions. The complexity of handling and maintaining sophisticated sequencing technologies presents another barrier, requiring specialized training and expertise.
Ethical Considerations
The rise of genome sequencing brings with it a host of ethical questions that cannot be overlooked. The ability to generate detailed genomic information raises concerns about consent and the understanding of risks involved in participating in genomic studies. Participants may not fully grasp how their genetic data will be used, shared, or stored.
Moreover, there is an ongoing debate regarding the consequences of genetic information, particularly in terms of discrimination in healthcare, employment, or insurance. The potential for misuse of genetic information looms large; thus, robust frameworks for ethical governance are essential to protect individuals' rights.
Furthermore, the quest for genomic data can unintentionally lead to disparities, where specific populations may be over-represented in research, skewing results and creating inequities. Researchers must remain vigilant and conscientious in considering these ethical implications as they advance the field.
Data Privacy Issues
As genome sequencing technology progresses, data privacy emerges as a significant challenge. The genetic information obtained is incredibly sensitive and personal. There is a heightened risk of this data being exposed or misused if not properly protected.
Researchers and institutions must navigate complicated regulations concerning data sharing and storage. Current frameworks may not be adequate to address the complexities of genetic data, leading to potential breaches of privacy. For instance, the sharing of genomic databases can inadvertently disclose individual identities if not anonymized properly, especially when combined with other datasets.
Furthermore, public trust is essential for successful genomic research. If individuals perceive that their genetic data is not protected adequately, they may withdraw from participation, hampering scientific advancement. It is imperative for institutions to adopt transparent practices around data collection, storage, and sharing to foster confidence and safeguard privacy.
"In tackling the challenges posed by genome sequencing, it is essential to balance innovation with ethical practices and robust data protection measures to ensure responsible advancement in this field."
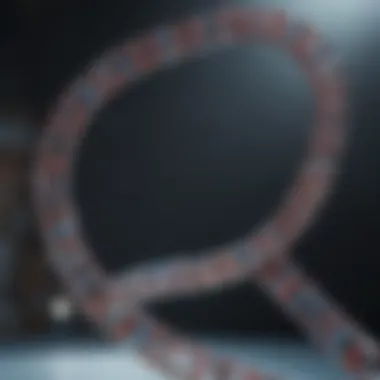
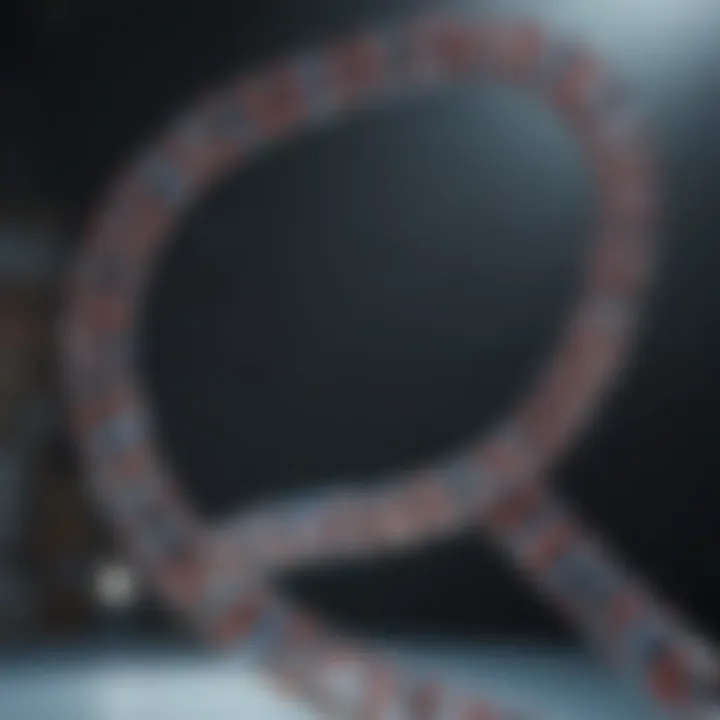
Overall, recognizing the limitations and challenges of genome sequencing machines is vital for developing responsible strategies that advance research while protecting individuals and communities.
Future Directions in Genome Sequencing Technology
The field of genome sequencing technology is rapidly evolving. New advancements promise to enhance both the speed and accuracy of sequencing processes. Understanding these developments is crucial for researchers and professionals in genetics and bioinformatics. Future directions also reflect the growing demand for personalized medicine and its applications in other domains like agriculture and public health.
Advancements in Speed and Accuracy
Recent improvements in genome sequencing machines focus significantly on speed and accuracy. Traditional sequencing methods often took weeks or months to produce results. However, the advent of next-generation sequencing (NGS) has reduced this time to mere hours or days. As technology progresses, researchers are seeking ways to further decrease the turnaround time without sacrificing accuracy.
For example, innovations such as adaptive sequencing allow machines to adjust their analysis techniques on-the-fly based on preliminary data. This agility enhances overall throughput and provides quicker insights into complex genetic sequences while maintaining reliable results. Moreover, accuracy is essential for clinical applications. Advances in error-correction algorithms and high-fidelity sequencing reagents are paramount. The integration of these advancements minimizes sequence discrepancies, which is vital when making diagnoses or crafting tailored treatment plans for patients.
Integration with Artificial Intelligence
Artificial Intelligence (AI) is positioned as a game-changer in the realm of genome sequencing, impacting data analysis and interpretation substantially. AI algorithms can process vast amounts of data generated by sequencing machines more efficiently than traditional methods. By employing machine learning, these systems learn from vast datasets and improve their predictions over time.
This capability proves particularly beneficial in genomic research, where interpreting mutations and their potential impacts can be daunting. Advanced analytical frameworks powered by AI enable researchers to identify patterns or anomalies more effectively. Consequently, AI can help accelerate discoveries in genetic disorders and potentially guide personalized treatment options. Its application can also support real-time decision-making in clinical settings, making genomic information more accessible and actionable.
The Role of Cloud Computing
Cloud computing facilitates the storage and processing of extensive genomic data, which is especially critical due to the massive output generated by modern sequencing technologies. As researchers generate terabytes of genomic data, traditional storage methods become inadequate. Cloud solutions offer scalable, secure, and cost-effective storage options.
Additionally, cloud platforms allow for collaborative research across institutions. Shared databases enable researchers to access and analyze a wealth of genetic information without geographical barriers. This collaborative environment enhances innovation and accelerates research outcomes.
Despite its advantages, data privacy issues remain a concern. Ensuring the security of sensitive genomic data is paramount. Researchers and institutions must implement robust security measures to comply with regulations while harnessing the power of cloud computing.
"The combination of speed, AI, and cloud computing presents opportunities for unprecedented innovations in genome sequencing and personalized medicine".
Future directions in genome sequencing technologies are driven by a continuous desire to enhance efficiency, both in terms of speed and accuracy. With the integration of AI and the scalability offered by cloud computing, landscape of genome sequencing machines is poised for transformative changes. Researchers and healthcare professionals must stay informed about these developments to leverage new advancements effectively.
Concluding Remarks
In concluding the discourse on genome sequencing machines, it is imperative to reflect on their transformative role in modern science and technology. These machines are not just sophisticated devices; they represent pivotal advancements in our ability to decode genetic information. Their ability to sequence genomes quickly and with remarkable accuracy has reshaped numerous fields, particularly in biomedical research and personalized medicine.
The Transformative Impact of Genome Sequencing
Genome sequencing has ushered in a new era of understanding genetic predispositions and disease mechanisms. The ability to read and interpret genetic codes allows researchers and clinicians to unveil hidden correlations between genetics and various health conditions.
- Precision Medicine: One of the most significant impacts is the development of precision medicine. By utilizing genetic information, healthcare providers can tailor treatments specifically to an individual’s genetic makeup. This enhances the effectiveness of therapies and minimizes adverse effects.
- Research Advancements: Genome sequencing has empowered researchers to investigate complex diseases with greater depth. It aids in identifying genetic markers crucial for early diagnosis and treatment of diseases such as cancer, diabetes, and hereditary disorders.
"The integration of genome sequencing in research allows for a much deeper understanding of the biological complexities underpinning health and disease."
- Agricultural Development: Beyond medicine, genome sequencing influences agricultural biotechnology. It enables the development of crops that are more resilient to pests, diseases, and environmental stresses. This leads to improvements in food security worldwide.
Call for Continued Research and Innovation
Regardless of these advances, challenges remain. Continuous research and innovation in genome sequencing technology are essential. Key areas to focus on include:
- Enhancing Accuracy: While current technologies are impressive, ongoing improvement in sequencing accuracy and efficiency is needed to ensure that genomic data is reliable.
- Ethical Standards: As genome sequencing becomes more integrated into healthcare, there is a growing need for ethical guidelines to govern its use. Patients' genetic data must be handled with care to avoid privacy breaches.
- Cost Reduction: Lowering the costs associated with sequencing remains a critical goal. Accessible genome sequencing could democratize healthcare, making personalized treatments available to a larger population.
In summary, as we stand at the forefront of genome sequencing technology, it is essential to foster a culture of continued research. Collaboration among scientists, healthcare professionals, and policymakers will drive future innovations and ensure that the benefits of genome sequencing can be harnessed safely and effectively.
Importance of References
- Validation of Information
The information presented in the article is underpinned by reputable sources, guaranteeing its accuracy. References help establish the authenticity of claims regarding the mechanisms, applications, and limitations of genome sequencing. This is essential for a field that impacts biomedical research, personalized medicine, and agricultural biotechnology. - Facilitating Further Research
By including a diverse range of citations, the article encourages readers to explore related works and expand their understanding. Individuals, whether students or professionals, will find an avenue to deepen their knowledge by following the references provided. The complexity of the subject matter makes this aspect vital since it allows for broader exploration of the nuances in sequencing technologies. - Contextualizing Advances and Discoveries
References offer historical insights and frame modern advancements within the context of previous work. Understanding how current genome sequencing machines evolved from earlier technologies is critical for appreciating their significance today. The citations can guide readers through seminal works, such as the Human Genome Project, laying the groundwork for recent innovations in sequencing technology.
Key Considerations
- Quality of Sources
It is important to rely on authoritative sources, such as peer-reviewed journals and established institutions. This assures readers that the information derived from references is based on rigorous scientific standards. - Diversity of Perspectives
Utilizing various sources, including articles from multiple disciplines, enriches the article and adds depth. The interconnected nature of genome sequencing implies that insights from genetics, bioinformatics, and ethical discussions should be included for a well-rounded examination.
The End
"The only way to learn is to create a space to ask questions and seek answers based on sound information."
This understanding will pave the path for continued research and innovation in the realm of genome sequencing.