Exploring Graphene Film: Properties, Applications, and Future
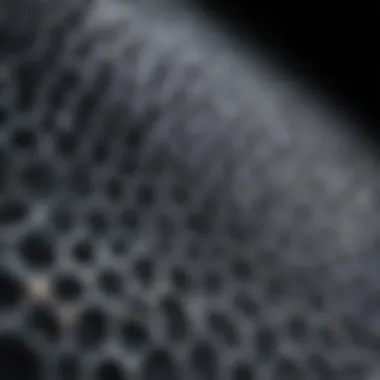
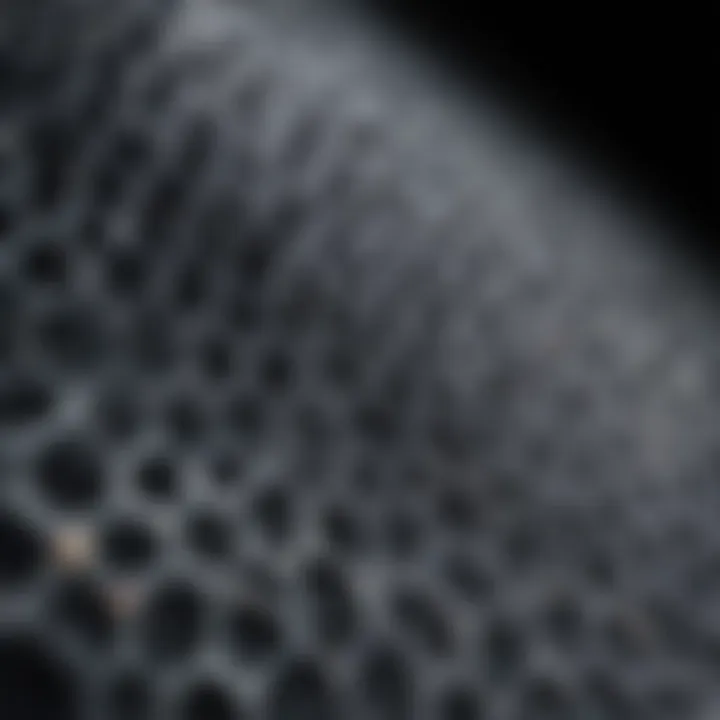
Intro
Graphene film has gained significant attention in recent years due to its unique properties and versatile applications. This single layer of carbon atoms arranged in a two-dimensional honeycomb lattice exhibits exceptional electrical, thermal, and mechanical characteristics. Researchers and industry professionals are increasingly exploring how graphene film can be integrated into various fields to advance technology and improve existing systems.
Understanding the production methods and potential applications of graphene film is crucial for fully appreciating its impact. From electronics to renewable energy and biomedicine, the implications of this material are vast, yet challenges remain in its effective implementation. This article aims to provide a comprehensive exploration of graphene film, focusing on current trends, research findings, and future prospects.
Research Overview
Key Findings
Recent studies have established several key findings regarding graphene film. These findings highlight its excellent conductivity, which can enhance the performance of electronic devices. Additionally, graphene films exhibit remarkable thermal conductivity, making them suitable for heat management applications in a variety of industries.
Moreover, the mechanical strength of graphene film surpasses that of traditional materials, positioning it as an ideal choice for lightweight and durable structures. The material also shows promise in flexible electronics, solar cells, and drug delivery systems, pointing to a diverse array of applications.
"Graphene film is becoming increasingly integral in technological applications due to its unique properties and potential to revolutionize several industries."
Study Methodology
The methodologies employed in the research on graphene film vary widely. Many studies utilize chemical vapor deposition (CVD) as a prominent method for producing high-quality graphene films. Other techniques include liquid-phase exfoliation and mechanical exfoliation. Each method has its own advantages and disadvantages in terms of scalability, quality, and cost-efficiency.
Research often involves a combination of experimental and computational approaches to investigate the properties of graphene film. Specific techniques like atomic force microscopy (AFM) and scanning electron microscopy (SEM) are employed to assess the film's structure and quality.
Background and Context
Historical Background
Graphene was first isolated in 2004, leading to a surge of interest across multiple disciplines. The Nobel Prize in Physics was awarded to Andre Geim and Konstantin Novoselov in 2010 for their groundbreaking work on graphene, cementing its significance in the scientific community. Since then, the refinement of production methods has accelerated research into its applications.
Current Trends in the Field
Currently, graphene research is experiencing notable trends. The focus has shifted towards scaling up production methods to meet industry demands and exploring novel applications in emerging technologies. Research institutes and companies are striving to unlock the full potential of graphene in sectors like communications, smart materials, and renewable energy.
The evolving landscape of graphene research underscores its significance in driving technological innovation. As methodologies improve and new applications are discovered, the journey of understanding graphene film is far from complete.
Intro to Graphene Film
Graphene film is emerging as a transformative material with diverse applications across numerous fields. Its significance stems from its unique properties, which include exceptional electrical and thermal conductivity, remarkable mechanical strength, and various optical attributes. Such characteristics position graphene film as a vital component in electronics, renewable energy systems, and biomedicine.
Understanding graphene film not only highlights its potential benefits but also informs ongoing research and development efforts in technology. This section sets the foundation for exploring its definitions, historical context, and discovery.
Defining Graphene Film
Graphene film is essentially a single layer of carbon atoms arranged in a two-dimensional lattice. This structure gives graphene its extraordinary properties. The material can be produced in varying thicknesses, but the most remarkable characteristics are often observed in monolayer forms.
Graphene exhibits high electrical conductivity, making it ideal for use in electronic devices. Its thermal properties allow for efficient heat management in various applications. Furthermore, the strength-to-weight ratio of graphene is unparalleled, which opens up opportunities in fields such as aerospace and automotive.
Historical Context and Discovery
The discovery of graphene is a relatively recent scientific achievement, with significant milestones occurring in the early 21st century. In 2004, Andre Geim and Konstantin Novoselov successfully isolated graphene using a simple method called mechanical exfoliation. This breakthrough earned the duo the Nobel Prize in Physics in 2010.
Prior to this, the theoretical concept of graphene had existed since the 1940s, but practical applications were not realized until the successful extraction methods were developed. Since then, interest in graphene research has surged, leading to innovative applications and methods of synthesis. With ongoing studies, scientists are continually uncovering additional potential uses and enhancing the material’s properties.
"The versatile nature of graphene films opens up new avenues for technological innovations across various industries."
In summary, the importance of graphene film lies not only in its unique properties but also in its historical journey from theoretical concept to practical application. This understanding serves as a vital context as we delve deeper into the properties, synthesis methods, and broad applications of this remarkable material.
Properties of Graphene Film
Graphene film's properties are central to understanding its significance in various technological and scientific applications. The unique characteristics of graphene film make it a material of immense interest in fields like electronics, energy storage, and biomedicine. Examining its properties elucidates why this single layer of carbon atoms arranged in a hexagonal lattice structure is hailed as a potential game-changer. The properties include electrical conductivity, thermal conductivity, mechanical strength, and optical characteristics.
Electrical Conductivity
One of the most notable features of graphene film is its extraordinary electrical conductivity. Graphene can conduct electricity better than copper due to its structure, allowing for high mobility of electrons. This property makes graphene film appealing for use in transistors, sensors, and flexible electronics. Its high electron mobility leads to faster signal transmission, paving the way for faster computing and more efficient electronic devices.
Graphene's ability to maintain high conductivity while being thin (just one atom thick) means that it can be incorporated into devices without adding significant weight or bulk. This can be crucial for applications like wearable technology, which requires both lightweight materials and efficient performance.
Thermal Properties
Graphene film exhibits remarkable thermal conductivity, surpassing that of copper. This property is vital for various applications, particularly in managing heat in electronic components, where excessive heat generation can impact performance and reliability. As electronic devices become smaller and more powerful, effective thermal management becomes increasingly important.
Additionally, graphene's thermal properties make it suitable for use in heat sinks and thermal interface materials. This can enhance the durability and efficiency of devices from computers to smartphones.
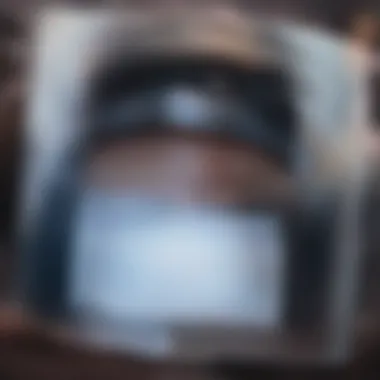
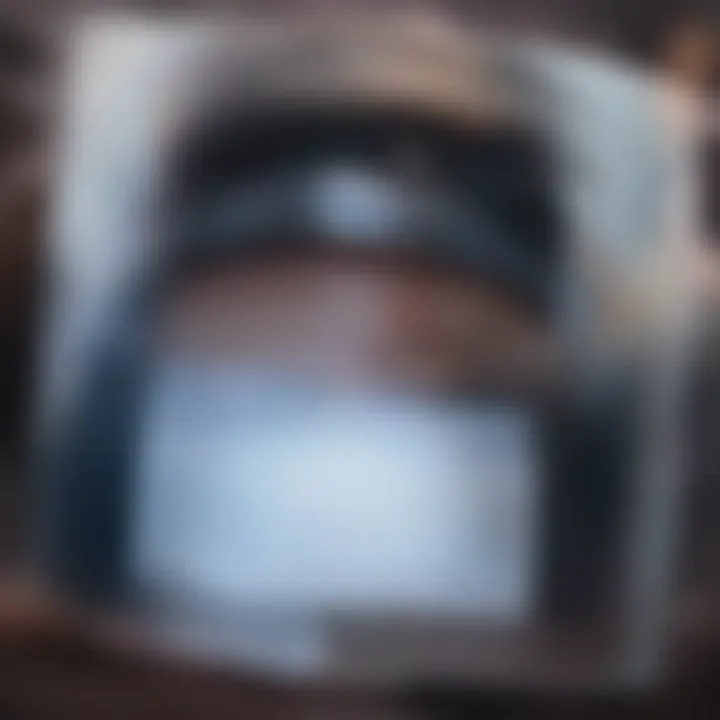
Mechanical Strength
Despite being incredibly thin, graphene film is remarkably strong. In fact, it is one of the strongest materials known, with tensile strength over 130 GigaPascals. This strength-to-weight ratio is unmatched, allowing graphene film to withstand significant stress without breaking.
The mechanical properties of graphene film can lead to innovations in the production of flexible electronics and durable composite materials. These materials can have applications spanning from construction to aerospace. For instance, integrating graphene into other materials can enhance their strength and resilience without adding bulk.
Optical Characteristics
Graphene film also possesses unique optical characteristics. It is nearly perfectly transparent, absorbing only about 2.3% of visible light. This quality makes it suitable for use in various photonic applications, including touchscreens and solar cells. Its transparency combined with its conductivity allows for the development of transparent conductive films, which are critical in modern displays and electronic devices.
Moreover, the interaction of graphene with light can lead to fascinating applications in photodetectors and other optical devices, enhancing their functionality while maintaining a slim profile. The behavior of electrons in graphene when exposed to electromagnetic waves can be exploited in advanced optical technologies.
In summary, the properties of graphene film contribute significantly to its versatility across various domains. The remarkable electrical conductivity, exceptional thermal properties, outstanding mechanical strength, and unique optical characteristics position graphene as a key player in future technological advancements. These properties not only promise improvements in existing technologies but also paint a picture of new possibilities that remain to be explored.
"Graphene’s potential is seemingly limitless in the realms of electronics, energy, and biomedical applications, reshaping the way we perceive material science."
Methods of Synthesis
The methods of synthesizing graphene film play a critical role in determining its quality, cost-effectiveness, and scalability. Each technique brings different qualities to the material, affecting its suitability for various applications. Understanding these synthesis methods is essential not just for researchers, but also for industries looking to utilize graphene film in their products. By deciding which synthesis method to use, one can influence the characteristics and performance of the resulting graphene film. Below, we discuss some of the primary methods employed today.
Chemical Vapor Deposition
Chemical Vapor Deposition (CVD) is one of the most widely used methods for producing high-quality graphene. This method involves depositing gaseous reactants on a substrate, where they react and form a solid material—in this case, graphene. CVD is particularly valued for its ability to produce large-area graphene films with superior electrical and thermal conductivity.
However, CVD has limitations. The process requires precise control of temperature and pressure, making it expensive and complex. The substrates used can also influence the properties of the graphene produced, leading researchers to explore various materials. Nonetheless, its capacity for high-quality production makes CVD a primary choice for commercial applications, particularly in electronics.
Liquid Exfoliation
Liquid exfoliation is another method for synthesizing graphene. This technique uses solvents to separate layers from bulk graphite. The process typically involves sonication, where high-energy sound waves agitate the graphite in the solvent. This method is generally more straightforward and cost-efficient than CVD, making it accessible for various small-scale applications.
Despite its advantages, liquid exfoliation has limitations in quality control. The resulting graphene layers may vary in thickness and quality, which can affect the material's properties. Researchers are continuously refining this method to enhance the uniformity and quality of the graphene sheets produced through this technique.
Thermal Reduction of Graphene Oxide
Thermal reduction of graphene oxide involves converting graphene oxide into graphene by subjecting it to high temperatures in an inert atmosphere. This process not only reduces the oxygen content but also restores the electrical conductivity of the material. The technique is relatively straightforward and can yield good-quality graphene if conditions are controlled well.
Challenges exist with this method too. The thermal reduction may not completely remove all oxygen-containing groups, which can affect the material's characteristics. Optimizing parameters like temperature and time is essential to achieve the best results. Researchers are actively studying this method for potential advancements in efficiency and quality.
Mechanical Exfoliation
Mechanical exfoliation, often referred to as the "Scotch tape" method, involves physically peeling graphene layers from graphite. This technique is simpler and cost-effective, making it useful for laboratory-scale applications. It usually provides high-quality single-layer graphene, suitable for various experimental purposes.
Yet, the limitations of mechanical exfoliation are significant. The process is not scalable for industrial production, as it can only produce small quantities of graphene. This restricts its use in commercial applications but continues to be favored in research environments for studies requiring high purity.
In summary, each synthesis method has its own set of advantages and drawbacks. The choice of method ultimately depends on the intended application of the graphene film. A thorough understanding of these synthesis techniques is fundamental to harnessing the true potential of graphene in diverse fields.
Applications in Electronics
Graphene film holds immense potential in the realm of electronics. Its unique properties, such as high electrical conductivity and mechanical flexibility, position it as a material that can revolutionize how electronic components are designed and integrated. With its ability to conduct electricity more efficiently than traditional materials, graphene allows for the development of smaller, faster, and more energy-efficient devices. This section explores specific applications within electronics, focusing on transistors and sensors, flexible electronics, and energy storage devices.
Transistors and Sensors
Transistors are fundamental to modern electronic devices. They act as switches or amplifiers for electronic signals. Graphene film can significantly improve transistor performance due to its high carrier mobility and low noise characteristics. This results in faster switching times and reduced power consumption.
Sensors also benefit from graphene's properties. The material's large surface area enhances its sensitivity, making it ideal for applications in biosensing. In particular, graphene-based sensors can detect biomolecules at low concentrations, which is critical in medical diagnostics.
Flexible Electronics
The shift towards flexible electronics is gaining momentum, and graphene film plays a crucial role. Traditional rigid components limit design possibilities and user experience. Graphene's thin, lightweight characteristics enable a new class of devices that can bend and fold. This flexibility opens up opportunities in applications like wearable devices and flexible displays.
In wearable technology, graphene film can be incorporated in smart textiles. These materials can monitor health metrics without sacrificing comfort. The integration of graphene provides both durability and functionality, offering better solutions for users.
Energy Storage Devices
Graphene film's advantages extend into energy storage applications. Supercapacitors and batteries stand to benefit from graphene's properties. It enhances charge storage capacity and longevity. Supercapacitors made with graphene can charge and discharge rapidly, making them suitable for applications requiring high power density.
While still in development, graphene-based batteries show promise in improving recharge times and energy density compared to traditional lithium-ion batteries. As research progresses, the adoption of graphene in energy storage could markedly enhance battery efficiency and performance.
"Graphene's role in electronics could lead to a new era in device efficiency and performance."
In summary, graphene film is an important material for advancing electronic applications. It has the potential to transform devices by enhancing performance, enabling flexibility, and improving energy storage solutions. The ongoing research and development will surely expand its implications in the field of electronics.
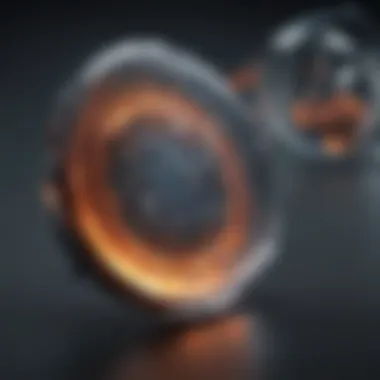
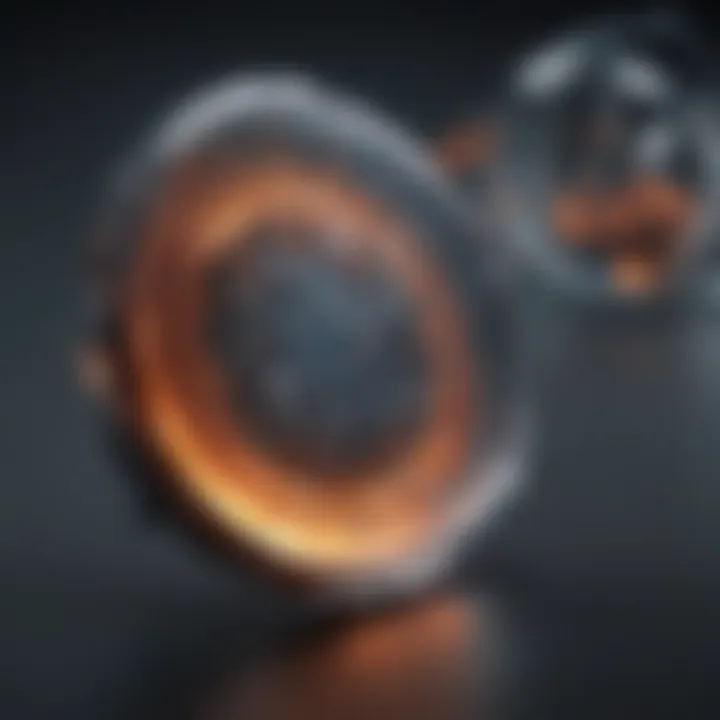
Impact on Renewable Energy
Graphene film has gained attention for its potential to significantly impact renewable energy sectors. This material's superior properties offer solutions to some of the most pressing challenges in energy efficiency and storage. As we transition to more sustainable energy sources, the role of graphene film could be vital for enhancing performance and reducing costs in renewable technologies.
One primary area of interest is its application in solar energy. Graphene film can greatly enhance the efficiency of solar cells. Thanks to its excellent electrical conductivity and transparency, it can serve as a conductive layer in photovoltaic cells. By providing better electron transport and reducing energy losses, graphene-based solar cells can achieve higher conversion efficiencies compared to traditional silicon-based cells. Furthermore, these cells can be made lighter and more flexible, broadening their installation possibilities and operational efficiencies.
Another area where graphene film shows promise is in energy storage devices. As the demand for efficient energy storage increases, especially with the growth of intermittent energy sources like solar and wind, supercapacitors made with graphene materials are emerging as a solution. They offer rapid charging and discharging capabilities, increased energy density, and a longer lifecycle than conventional batteries. These characteristics can make energy storage more accessible, promote the use of renewable sources, and aid in grid stability.
The incorporation of graphene film into renewable energy applications presents both opportunities and challenges. On one hand, it can lead to innovations and improved efficiency. On the other hand, issues related to the scalability of graphene production and its integration into existing manufacturing processes need to be resolved. Despite these challenges, the potential benefits of graphene film in renewable energy applications make it a topic worth further exploration and investment.
"Graphene's extraordinary properties could redefine the standards for energy efficiency and effectiveness in renewable technologies."
Solar Cells
Solar cells are one of the most promising applications of graphene film in renewable energy. The integration of graphene into photovoltaic systems has the potential to revolutionize solar technology. Currently, traditional solar cells, primarily made from silicon, face limitations such as weight, cost, and inefficiency in energy conversion.
Graphene film shows potential to overcome these challenges. For instance, its excellent electrical conductivity means that it can enhance the flow of electrons generated by sunlight, leading to higher energy conversion rates. Additionally, since graphene is both transparent and strong, it can be used as a protective and conductive layer over solar panels. This not only improves durability but also reduces the weight compared to traditional materials.
The ability to manufacture flexible solar panels is another significant advantage. Unlike standard rigid panels, graphene-based cells can be applied to various surfaces, enabling applications on vehicles, buildings, and even clothing.
Supercapacitors
In the realm of energy storage, supercapacitors represent a critical technology for managing the electricity supply from renewable sources. Graphene film enhances the performance of supercapacitors by offering higher energy and power density. It can store and release energy quickly, which is crucial for applications requiring short bursts of power.
The high conductivity of graphene not only supports rapid charging but also contributes to a longer lifecycle compared to traditional battery technologies. This durability makes graphene-enhanced supercapacitors suitable for diverse applications ranging from consumer electronics to electric vehicles and grid stabilization.
Furthermore, with the rise of electric vehicles, the demand for efficient supercapacitors is essential. Graphene can reduce the weight, volume, and cost of energy storage systems, making electric transportation more efficient and accessible.
In summary, both solar cells and supercapacitors highlight the vast potential of graphene film in renewable energy applications. Continued research and investment in these areas could lead to breakthroughs that promote sustainability and create a more efficient energy future.
Applications in Biomedicine
The exploration of graphene film in biomedicine presents a compelling domain, integrating innovative material science with health and medicine. With its unique properties, graphene film opens up pathways for enhanced diagnostic and therapeutic techniques that hold significant promise. As such, this section will discuss specifically the applications of graphene in drug delivery systems and biosensors, highlighting the benefits, considerations, and potential advancements in medical technology.
Drug Delivery Systems
Graphene film plays a crucial role in the evolution of drug delivery systems. The material's high surface area and biocompatibility make it an ideal candidate for drug transport applications. One notable benefit is the ability to create targeted drug delivery mechanisms. This specificity can potentially minimize side effects by concentrating therapeutic agents directly at the disease site.
In addition, graphene's excellent conductivity can facilitate the release of drugs through external stimuli such as electric fields or ultrasound. This controllable release mechanism not only enhances effectiveness but also allows for scheduled administration, improving patient compliance.
However, challenges remain in biocompatibility and the potential for toxicity in certain forms of graphene. Researchers are actively investigating modifications to the surface of graphene to improve these properties while still leveraging its advantages. Rigorous testing is essential to ensure safety before practical applications can be widely adopted.
"The integration of graphene film in drug delivery systems signifies a leap forward in personalized medicine, allowing for treatments that are not only more effective but also safer for patients."
Biosensors
The potential of graphene film extends into the realm of biosensors. Graphene's properties enable the creation of highly sensitive biosensors that can detect low concentrations of biological markers. These markers are crucial for early diagnosis of diseases such as cancer and diabetes.
Biosensors made with graphene film offer several key advantages. Their high sensitivity allows for rapid and accurate detection, which is essential in clinical settings. Furthermore, these sensors can be designed to be portable and easy to use, facilitating point-of-care testing outside traditional lab environments.
Moreover, the versatility of graphene allows for integration with different types of detection mechanisms, such as electrochemical and optical sensors. This flexibility ensures that biosensors can be tailored for various applications, leading to broader adoption across medical fields.
Nonetheless, the development of graphene-based biosensors is not without hurdles. The stability of the sensors in biological environments and their long-term performance are areas that require thorough investigation. Addressing these concerns will be pivotal to the successful implementation of graphene biosensors in everyday medical practice.
Environmental Applications
The use of graphene film extends into several environmental applications, offering unique solutions to pressing ecological issues. These applications demonstrate both the functional advantages of graphene film and its potential to contribute to sustainability. As the world grapples with challenges like water scarcity and air pollution, innovations derived from graphene could lead to significant improvements in these areas.
Water Filtration
Water contamination poses a dire threat to health and ecosystems. Traditional filtering methods struggle against tiny impurities and chemical contaminants. Graphene film, with its remarkable properties, provides a feasible alternative. The film's porous structure allows for efficient filtration. By utilizing graphene's high surface area, researchers can enhance water treatment processes.
Recent studies highlight the capacity of graphene oxide membranes. These membranes can selectively filter ions and molecules at molecular levels. Their application can reduce hazardous materials in drinking water, offering cleaner solutions.
Some potential benefits of using graphene film in water filtration include:
- High efficiency: Capable of filtering nanometer-sized particles.
- Cost-effectiveness: Potential to reduce costs compared to conventional filtering systems.
- Durability: Long-lasting materials which need less frequent replacement.
While promising, there are considerations to address. Environmental impact during production and the adherence to regulatory standards are critical. Researchers must ensure that the synthesis of graphene film does not introduce new pollutants into the environment.
Air Purification
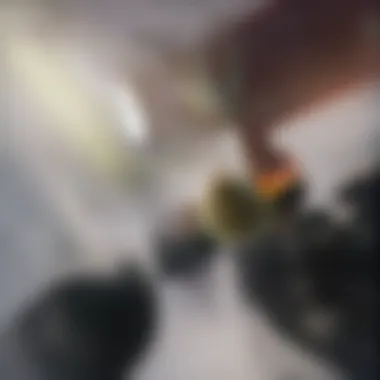
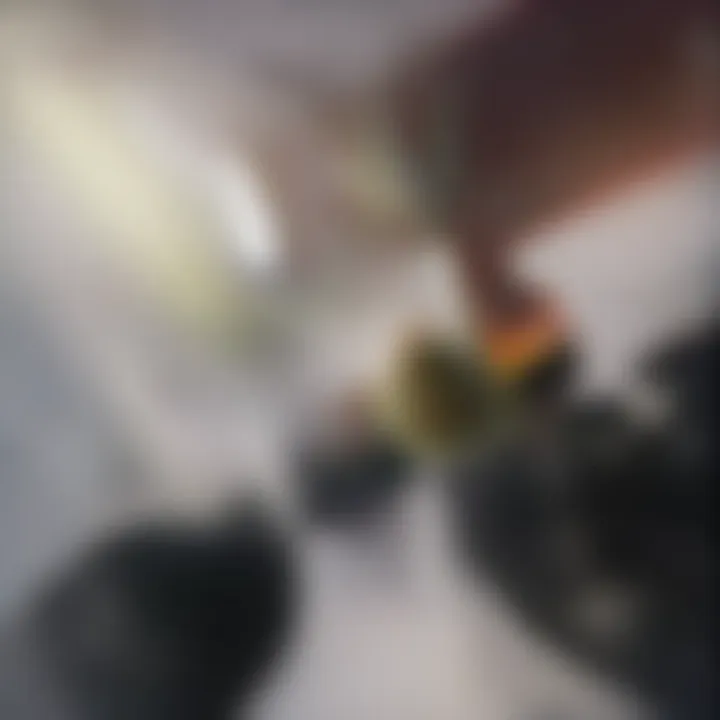
Air quality significantly impacts human health and the environment. Graphene film applications can be employed in air purification technologies. These films can assist in filtering airborne pollutants, such as volatile organic compounds and particulate matter.
Graphene's unique properties, including high conductivity and surface reactivity, make it suitable for use in devices that detect and remove harmful substances. Studies indicate the ability of graphene-based materials to adsorb toxic gases, improving air quality effectively.
Some benefits of using graphene in air purification include:
- Enhanced adsorption: Increased surface area leads to better pollution absorption.
- Integration into existing systems: Graphene films can be added to current air purification technologies.
- Fast-paced response: Greater efficiency in detecting and removing pollutants due to rapid adsorption qualities.
However, researchers must address challenges related to scalability. The production methods for graphene films need optimization for mass utilization. Furthermore, performance must be validated in different environments before widespread implementation.
Conclusion: The potential of graphene film for environmental applications represents a significant advancement. Addressing both water filtration and air purification bolsters the promise of graphene in solving crucial environmental issues. As research progresses, the hope is that graphene may play an even larger role in sustainability efforts.
Challenges in Graphene Film Research
Graphene film is a material with exciting potential, but its widespread application faces several challenges. These hurdles are crucial for understanding the current obstacles that the graphene industry must address before it can fully realize its promise in various sectors. Exploring the challenges in research not only shines a light on the limitations but also emphasizes the opportunities for innovation and improvement. As researchers work tirelessly to overcome these difficulties, the path to integrating graphene into commercial applications becomes clearer.
Scalability of Production Techniques
One of the primary challenges is the scalability of production techniques. While lab-scale synthesis of graphene film has been achieved successfully, transitioning to large-scale production involves complex considerations. Methods like Chemical Vapor Deposition and Liquid Exfoliation have been utilized, yet they often result in limited yield and high costs. The need for a cost-effective and efficient process is vital for the commercialization of graphene products.
Challenges related to scalability also include:
- Quality Consistency: Maintaining uniform quality across large batches is difficult, as small variations can impact the film's performance.
- Environmental Concerns: Many production methods may produce toxic byproducts, which complicates large-scale implementation.
- Resource Availability: Ensuring a steady supply of raw materials for production can pose risks, especially as demand increases.
Integration with Existing Technologies
Another significant hurdle is the integration of graphene film with existing technologies. While graphene offers enhanced performance capabilities, adapting current systems to utilize this novel material can be complex. This integration faces various challenges such as:
- Compatibility Issues: Graphene's properties may not align well with traditional materials used in electronics and other applications, necessitating redesigns.
- Standardization: The lack of standardized processes for incorporating graphene into products hampers development and widespread adoption.
- Industry Resistance: Industries may be hesitant to adopt new materials due to the cost and risk associated with changing established practices.
"The successful integration of graphene into existing systems is imperative to unlock its true potential and drive innovation across multiple fields."
Addressing these challenges is essential for unlocking the full range of applications and benefits of graphene film. A focused approach looking at scalability and integration with existing technologies can pave the way for future advancements.
Future Prospects of Graphene Film
The ongoing exploration of graphene film presents significant opportunities for advancement in numerous sectors. Understanding future prospects is crucial. Innovations can change how we approach technology and material science. New research is constantly revealing further potential for graphene film, opening the door to previously unexplored applications.
Emerging Trends in Research
Research trends currently focus on enhancing the synthesis methods of graphene film. Efforts aim to optimize production, making it more cost-effective. For instance, developments in Chemical Vapor Deposition can lead to better quality films at lower costs.
Additionally, integrating graphene film with existing materials can create hybrid systems. These can have superior properties, enhancing performance in various applications. Researchers are also exploring ways to functionalize graphene with other compounds. This could lead to tailored properties suitable for specific uses.
Moreover, studies into the environmental impact of graphene reflect a vital trend. Understanding how to recycle and dispose of this material responsibly is imperative. As graphene's popularity grows, so does the responsibility surrounding its lifecycle.
Innovations in electronic applications are another key trend. Research is focused on developing graphene-based devices, such as flexible electronics and next-generation transistors. These would not only be more efficient but also enable new forms of technology that are lighter and more adaptable.
"Graphene's unique properties open new avenues for technological advancements that we have only begun to explore."
Potential New Applications
The potential for new applications of graphene film is vast. In the medical field, its use in biosensors and drug delivery systems is expanding. Novel approaches could make treatments more effective and targeted. The ability of graphene to interact with biological systems makes it suitable for these applications.
In the energy sector, graphene films are looking promising in solar energy. Developing high-efficiency solar cells using graphene could reduce costs and improve energy capture levels. Similarly, advancements in supercapacitors could be revolutionized by graphene-based technologies, providing fast charging capacities.
Graphene is also gaining attention for its environmental applications. For water filtration systems, incorporating graphene can enhance filtration efficiency significantly. This can have major implications for addressing global water scarcity. In industrial settings, graphene could provide advanced filtering for toxic substances in air quality systems.
In summary, the future of graphene film is not just about its properties but also about how it can transform various industries. Continued research will likely unveil many more promising applications. As the field grows, the implications for technology and everyday life are expected to be profound.
End
The conclusion of this article serves as a pivotal moment to reflect on the significance of graphene film. It is essential to understand that graphene film stands as a remarkable material with varied properties, birthed from unique production techniques. The potential it holds influences multiple fields, ranging from electronics to renewable energy and biomedicine. This section will synthesize the key insights presented earlier while stressing the continuous innovation surrounding graphene film.
Summary of Key Points
In summary, several key points emerge from the exploration of graphene film:
- Defining Characteristics: Graphene film boasts remarkable electrical, thermal, and mechanical properties that set it apart from traditional materials.
- Synthesis Techniques: Various methods of production, such as chemical vapor deposition and liquid exfoliation, play a crucial role in determining the quality and application of graphene.
- Electronics Applications: The use of graphene in transistors, sensors, and flexible electronics highlights its transformative potential in the tech industry.
- Renewable Energy: Graphene's role in solar cells and supercapacitors presents significant opportunities in the quest for sustainable energy solutions.
- Biomedical Uses: Innovations in drug delivery systems and biosensors introduce new possibilities in healthcare and personalized medicine.
- Environmental Impact: Its application in water filtration and air purification showcases graphene's potential for addressing pressing environmental challenges.
- Current Challenges: Understanding issues like scalability and technology integration is crucial for future advancements in graphene film research.
- Future Prospects: Ongoing research suggests emerging trends and potential applications that could revolutionize various industries.
Final Thoughts on the Importance of Graphene Film
Graphene film holds a unique position in modern material science. Its implications stretch across diverse domains, fostering innovation and facilitating technological advancements. As research progresses, the focus on overcoming existing challenges will become even more critical. Stakeholders in technology, energy, and medicine need to pay attention to developments in graphene film, as it could serve as a cornerstone for future solutions.
Additionally, education and collaborative efforts among researchers and industry professionals are vital. They will ensure that the potential of graphene is harnessed effectively and ethically. The journey of understanding and applying graphene film is only beginning. Therefore, appreciating its capabilities and facing the challenges ahead can lead to significant achievements that benefit society as a whole.
"Graphene film is not just a material; it represents the forefront of innovation and possibility in scientific inquiry."
Overall, as we reflect on the comprehensive exploration of graphene film, its significance cannot be overstated. It stands as a testament to the power of research and the need for continual exploration in unlocking the secrets of remarkable materials.