Exploring Non-Spherical Particulate Systems
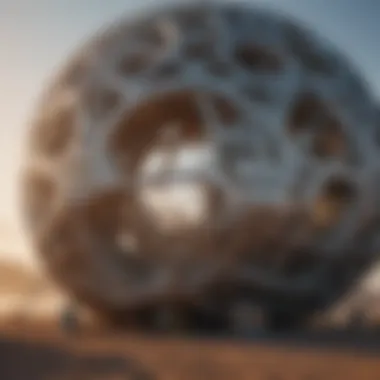
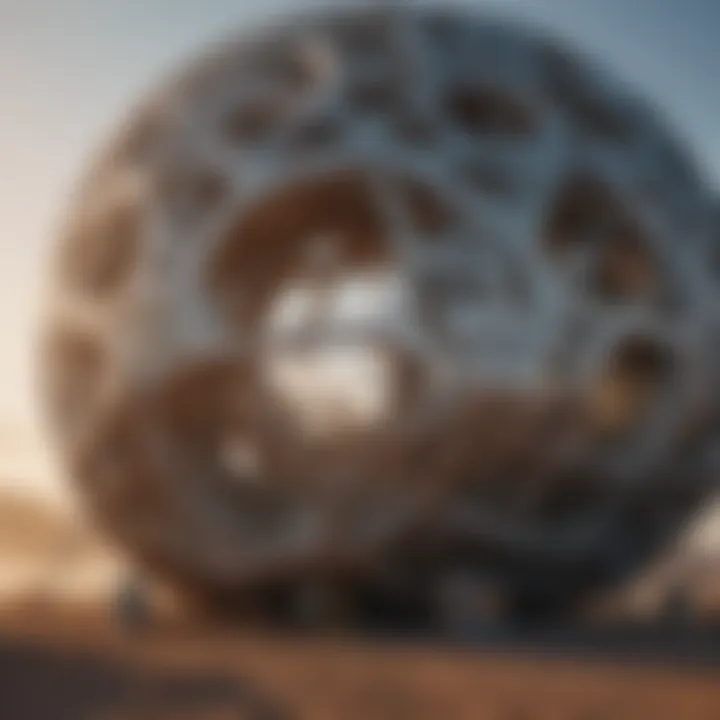
Intro
Non-spherical particulate systems (NSPCs) have gained a lot of attention in various scientific fields. These systems include particles that deviate from the common spherical shape. Understanding NSPCs is vital for researchers and professionals because their unique properties can significantly impact applications in industries ranging from materials science to biology. This article sets to provide a comprehensive overview of NSPCs, highlighting their importance, methodologies for their analysis, and the latest advancements in the field.
Research Overview
In this section, we will review key findings and the methodologies used in recent studies on NSPCs.
Key Findings
Recent research reveals that NSPCs exhibit distinct behaviors that influence their interaction with biological environments, fluid dynamics, and material properties. Some significant findings include:
- NSPCs can enhance drug delivery efficiency in biomedical applications.
- Their characteristic shapes provide advantages in catalysis and filtration processes.
- The mechanical properties of NSPCs can be tuned through design, impacting the strength and durability of materials.
Understanding these nuanced properties is essential as industries pursue innovations that leverage the unique attributes of NSPCs.
Study Methodology
Researchers employ a variety of approaches to characterize NSPCs. Common methods include:
- Electron Microscopy: Provides high-resolution images of particle morphology.
- Dynamic Light Scattering: Helps in determining size distributions of non-spherical particles in solution.
- Computational Modeling: Utilizes simulations to predict the behavior of NSPCs in various environments.
Each of these methodologies contributes to a better understanding of how non-spherical shapes affect performance in practical applications.
Background and Context
A comprehensive examination of NSPCs requires a look at their historical background and current trends within the scientific community.
Historical Background
The study of particles has been of interest for many decades. Initially, most focus was on spherical particles due to their simplicity in representation and analysis. However, researchers soon recognized that non-spherical shapes could potentially offer greater functionality in various applications. The shift to study NSPCs commenced in various sectors, such as medicine and materials science, as the implications of shape became clearer.
Current Trends in the Field
Today, advancements in technology have led to innovative methods for creating and analyzing NSPCs. Notable trends include:
- Increasing integration of nanotechnology with NSPC design.
- Development of software tools for simulation and modeling.
- Greater collaboration among disciplines to address complex challenges involving NSPCs.
The field is rapidly evolving and expanding, leading to novel applications and insights into the role of particle shape in scientific processes.
Preface to Non-Spherical Particulate Systems
The study of non-spherical particulate systems (NSPCs) presents a unique perspective in various scientific fields. Understanding these systems is critical for multiple reasons. Non-spherical particles can influence physical, chemical, and biological processes in distinctive ways compared to their spherical counterparts. Their irregular shapes lead to complex interactions that are fundamental in areas such as materials science, biomedical applications, and environmental science.
Through this article, we will dissect the complexities involved in NSPC research. Each section will encompass definitions, theoretical foundations, characterization, applications, challenges, and future trends. This roadmap aims to aid students, researchers, educators, and professionals in navigating the intricate landscape of NSPCs. Understanding NSPCs not only advances scientific knowledge but also enhances technological applications, leading to breakthroughs in various sectors.
Definition of Non-Spherical Particulate Systems
Non-spherical particulate systems are defined as collections of particles that do not conform to a standard spherical shape. These particles can exhibit a range of geometries, including but not limited to ellipsoids, rods, discs, and irregular shapes. This variance in morphology significantly influences the behavior of the particles in different environments.
The specificity of the shape leads to distinctive attributes, such as altered flow properties, enhanced surface area, and varying chemical reactivity. Such behaviors are essential when considering applications in fields such as catalysis, nanotechnology, and drug delivery.
Importance in Scientific Research
The significance of NSPCs in scientific research cannot be overstated. Their unique properties and behaviors make them vital subjects for exploration in numerous disciplines.
- In biological systems, non-spherical particles can mimic natural cells or cellular components, enhancing drug delivery systems or biosensor function.
- Within the domain of industry, their application can lead to the development of more efficient catalysts and improved material properties in nanocomposites.
- Environmental scientists study NSPCs for their role in pollution measurement and remediation strategies, leading to more effective solutions for environmental challenges.
"Understanding non-spherical particles goes beyond traditional spherical models; it opens avenues for innovative discoveries across multiple scientific domains."
The complexity introduced by non-spherical shapes demands advanced analytical techniques and theoretical models, which can drive innovation and foster interdisciplinary collaborations. As NSPCs continue to gain prominence, a deeper understanding will promote scientific advancement and practical applications.
Theoretical Foundations
The theoretical foundations of non-spherical particulate systems (NSPCs) are essential for grasping their complex behaviors and interactions. By understanding these foundations, researchers can better characterize the properties and applications of non-spherical particles. It is clear that the geometry and dynamics of these particles play a vital role in determining their functionalities in various disciplines, such as biology and materials science. Equipping oneself with theoretical knowledge aids in troubleshooting experimental challenges and enhances the predictive power of computational models. Thus, these foundations establish a robust framework from which further empirical and applied research can proceed.
Geometric Representations
Geometric representations refer to the mathematical models used to describe the shapes and forms of particles. The study of NSPCs requires going beyond traditional spherical models as real-world particles often display complex geometries. Each shape, from ellipsoids to rods, influences physical behavior such as flow characteristics and interaction with surrounding media.

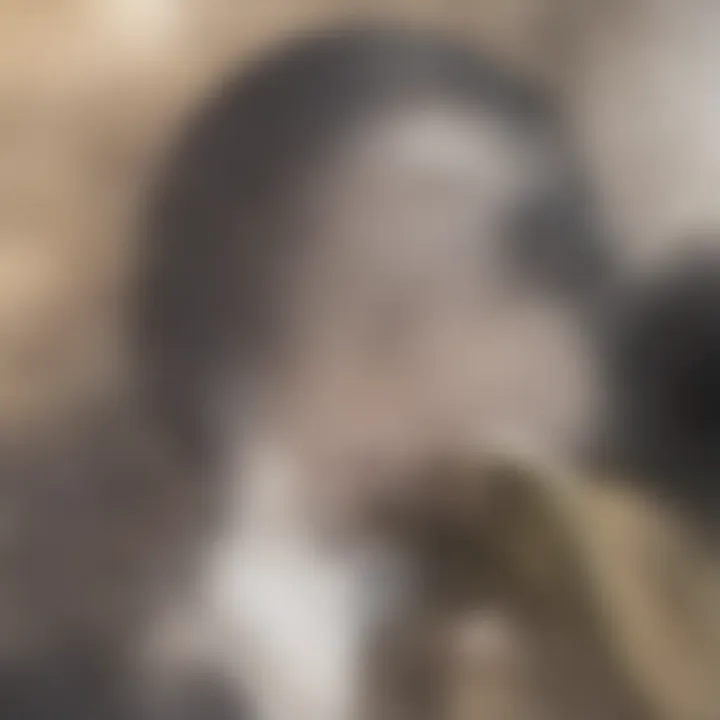
A crucial aspect of geometric representation is its ability to inform properties like surface area, volume, and mass distribution. This information is vital for predicting reactivity and behavior in various environments. Techniques such as image analysis or computational geometry can be employed to quantify these shapes effectively.
Dimensional Analysis and Scaling Laws
Dimensional analysis is a systematic approach to drawing relationships between different physical quantities. It simplifies problems involving NSPCs by reducing complex variables into more manageable forms. Scaling laws, on the other hand, help in understanding how changes in size or shape affect properties at larger or smaller scales.
In the context of NSPCs, dimensional analysis can lead to insights into the flow behavior in suspensions, sedimentation rates, and diffusion patterns. Scaling laws allow researchers to extrapolate findings from microscopic studies to macroscopic applications. For instance, understanding how particle shape influences the effective viscosity of a suspension can guide formulations in fields like pharmaceuticals and food technology.
Statistical Mechanics Approaches
Statistical mechanics provides a framework for relating microscopic particle behavior to macroscopic properties. This approach is key when studying NSPCs because it allows for the connection between the collective behavior of a large number of particles and their individual characteristics.
By employing statistical mechanics, researchers can investigate phenomena such as phase transitions and thermodynamic properties of NSPCs. These insights are essential for application areas, including material development and environmental science. For example, understanding the distribution and arrangement of non-spherical particles can contribute to designing better catalysts or sensors.
The convergence of geometric representation, dimensional analysis, and statistical mechanics creates a comprehensive understanding of NSPCs that is invaluable in both fundamental and applied research.
Characterization Techniques
The characterization of non-spherical particulate systems (NSPCs) is essential for understanding their unique properties and behaviors. This process involves using various techniques that allow researchers to observe, analyze, and measure the physical characteristics of these particles. Each method provides distinct insights, which can enhance the comprehension of how NSPCs function in different settings. Proper characterization aids in optimizing applications across scientific fields, from biology to material science.
Microscopy Methods
Microscopy is a pivotal technique in the characterization of NSPCs. It enables visualization at a microscopic level, allowing detailed analysis of particle shape, size, and distribution. There are two primary forms of microscopy used in this context.
Transmission Electron Microscopy
Transmission Electron Microscopy (TEM) stands out for its ability to provide high-resolution images of particles at the nanoscale. Utilizing a beam of electrons, TEM passes through a specimen, revealing intricate details of non-spherical shapes. The key characteristic of TEM is its exceptional resolution, which can reach up to 0.1 nanometers.
This method is a popular choice due to its unparalleled detail. The unique feature of TEM lies in its capability to visualize internal structures, offering insights not just into the surface, but also the interior of particles. However, the downsides include sample preparation challenges and the requirement for a vacuum environment, which may not always be feasible.
Scanning Electron Microscopy
In contrast, Scanning Electron Microscopy (SEM) provides three-dimensional imaging by scanning the surface of the sample with a focused beam of electrons. One of the key strengths of SEM is its versatility in analyzing larger areas and a wide range of materials.
This technique is beneficial due to its ability to produce high-resolution images with depth of field, making it easier to analyze and interpret non-spherical structures. The unique feature of SEM is that it usually does not require complex sample preparation compared to TEM. Nevertheless, it has some limitations, such as lower resolution compared to TEM and the inability to image bulk materials without specific preparations.
Spectroscopic Techniques
Spectroscopic techniques complement microscopy by providing information about the composition and properties of NSPCs. Two notable methods are Dynamic Light Scattering and X-ray Diffraction.
Dynamic Light Scattering
Dynamic Light Scattering (DLS) is crucial for determining the size distribution of small particles in suspension. It measures the fluctuations in intensity of light scattered by a sample. The key characteristic of DLS is its ability to analyze samples quickly and in real time.
This method is beneficial because it offers insights into the average particle size, which is essential for many applications including drug delivery. The unique feature of DLS is its suitability for colloidal systems. However, it can produce inaccurate results if the particles are not adequately dispersed or if they aggregate.
X-ray Diffraction
X-ray Diffraction (XRD) plays an essential role in determining the crystalline structure of non-spherical particles. By observing the angles and intensities of X-rays scattered off a sample, researchers can infer various structural properties. A significant advantage of XRD is its capability to provide information about phase and crystallinity.
This method is popular due to its reliability and relatively straightforward sample preparation. The unique feature of XRD is that it can analyze materials in bulk form. However, it is often limited to crystalline materials, which might exclude some colloidal systems from analysis.
Computational Modeling
Computational modeling is increasingly relevant for characterizing NSPCs, offering a powerful avenue for simulation and prediction. Two noteworthy techniques within this category are Particle Dynamics Simulation and Finite Element Analysis.
Particle Dynamics Simulation
Particle Dynamics Simulation (PDS) allows researchers to virtually observe and predict the behavior of non-spherical particles under various conditions. The key characteristic of PDS is its ability to handle a vast number of particles, giving insights into collective behavior and interactions.
PDS is beneficial because it can model complex systems which may be impractical to study experimentally. The unique feature of PDS involves its adaptability to different physical forces. However, it requires significant computational resources and may involve approximations that can affect accuracy.
Finite Element Analysis
Finite Element Analysis (FEA) is another prominent technique for modeling the mechanical response of non-spherical particles. This approach divides the particle into smaller elements for analysis, allowing for detailed stress, strain, and displacement calculations. A key strength of FEA lies in its ability to analyze complex geometries.
This method is beneficial for studying how non-spherical particles respond to external forces, making it crucial in fields like materials science and engineering. The unique feature of FEA is its effectiveness in predicting material behavior under various conditions. However, it may be less effective for particles with extreme shapes or in cases with highly irregular features.
Applications of NSPCs
Non-spherical particulate systems (NSPCs) have crucial roles across various fields of research and industry. Their unique shapes and properties lead to distinctive behaviors and functionalities. Understanding these applications provides insights into the benefits these systems offer. For example, NSPCs can enhance drug delivery efficiency in medical settings, while also finding utility in environmental monitoring, aiding in pollution measurement and remediation strategies. Its this versatility that makes studying NSPCs vital.
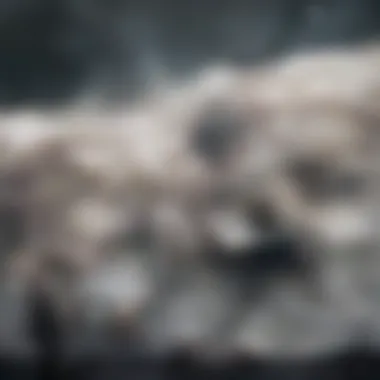
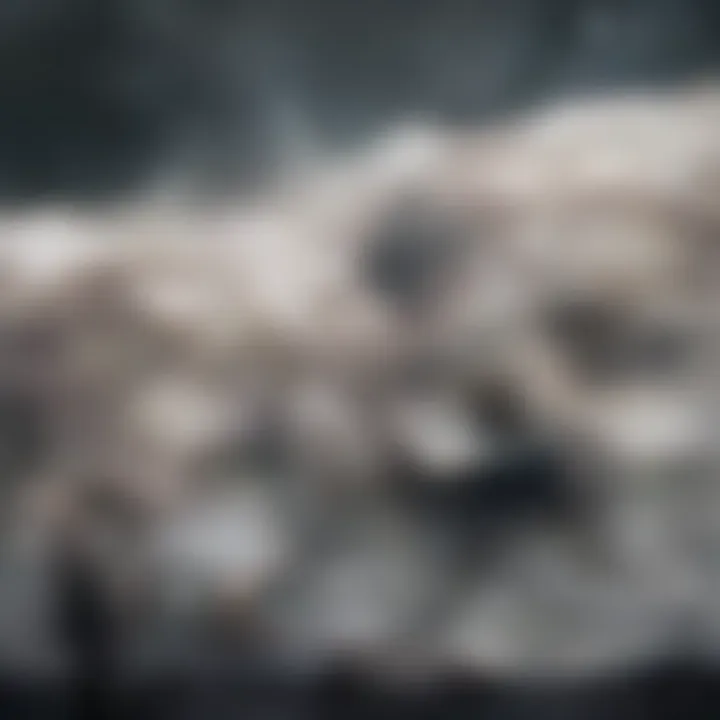
A few specific areas where NSPCs are utilized include:
- Biological Systems
- Industrial Applications
- Environmental Implications
Each of these areas reveals the growing influence of NSPCs on technology and its evolution.
Biological Systems
Drug Delivery Systems
Drug delivery systems rely on NSPCs to enhance the bioavailability of therapeutic agents. The shape and surface characteristics of these particles improve their interaction with biological membranes. Their unique feature allows for targeted delivery, which minimizes side effects and enhances therapeutic efficacy. This specificity is a major reason why NSPCs are a popular choice for research in drug delivery.
Some advantages of drug delivery systems include:
- Improved pharmacokinetics
- Enhanced stability of drugs
- Targeted release mechanisms
However, challenges remain. For instance, the manufacturing process of NSPCs can be intricate. Ensuring consistency and reproducibility is essential to avoid patient-specific variations.
Biosensors
Biosensors utilizing NSPCs are a critical aspect of health diagnostics. They can detect biological signals with high sensitivity and specificity. The shape and composition of these sensors allow for better binding and interaction with analytes. This leads to rapid and accurate measurement of biological molecules.
Key characteristics include:
- High surface area-to-volume ratio
- Real-time signal transduction
Yet, these biosensors may face limitations in terms of stability under varying environmental conditions. This may affect their long-term applicability in clinical settings.
Industrial Applications
Catalysts
In industrial chemistry, NSPCs serve as highly efficient catalysts. Their unique geometry increases the active surface area, enhancing reaction rates. This characteristic makes NSPCs a favored choice. They can facilitate a variety of chemical reactions, leading to improved process efficiency.
Advantages of using NSPC catalysts involve:
- Increased reaction rates
- Lower energy requirements
On the downside, catalyst deactivation can occur due to fouling. This can complicate their performance and longevity in industrial processes.
Nanocomposites
Nanocomposites comprising NSPCs are well-known for their enhanced mechanical and thermal properties. The incorporation of these unique particles into a matrix material can significantly improve its performance. This makes nanocomposites appealing for a variety of applications, including construction and electronics.
Key features of nanocomposites are:
- Improved strength-to-weight ratio
- Enhanced thermal stability
Nonetheless, challenges like dispersion of NSPCs in the matrix and the potential increase in production costs must be evaluated.
Environmental Implications
Pollution Measurement
In environmental science, NSPCs are applied in pollution measurement techniques. Their distinct properties make them suitable for detecting specific pollutants at very low concentrations. This capability is invaluable for environmental monitoring and assessment.
They enable:
- Sensitive detection of contaminants
- Faster response times in analysis
Challenges may include the need for specific conditions to maintain performance, which could limit their application in varied environments.
Remediation Strategies
NSPCs are also at the forefront of environmental remediation strategies. They can adsorb harmful substances from contaminated water and soil due to their increased surface area. This adsorption capacity can lead to more effective cleanup processes.
Advantages of using NSPCs in remediation strategies include:
- Efficient removal of contaminants
- Reduced overall environmental impact
However, factors such as cost and scalability of using NSPCs in large remediation projects may pose challenges.
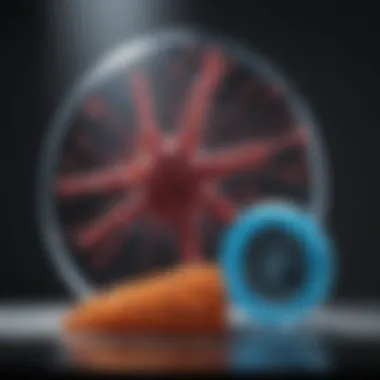
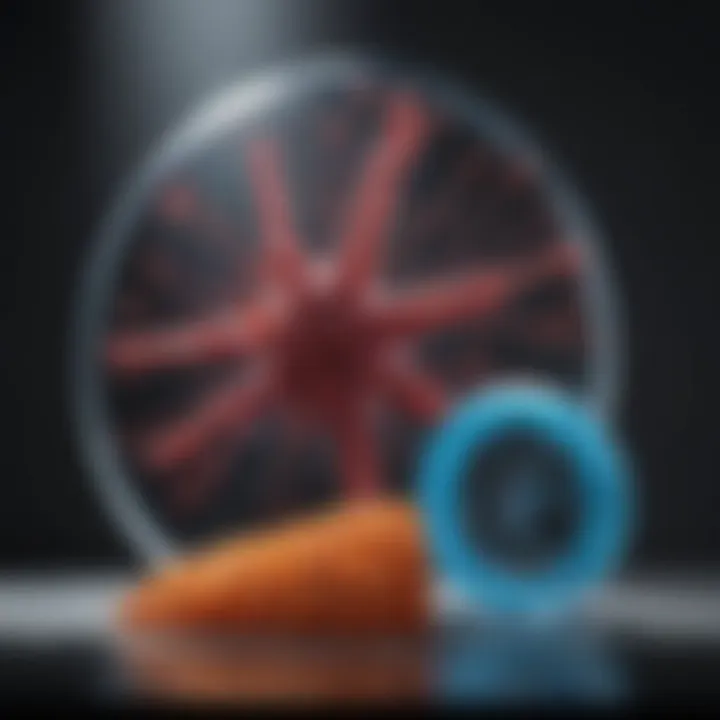
Challenges in NSPC Research
The exploration of non-spherical particulate systems (NSPCs) unveils a range of complex challenges that researchers must navigate. These obstacles impact the understanding and application of NSPCs across various scientific disciplines. In this section, we will examine three primary challenges: characterization limitations, standardization issues, and data interpretation difficulties. Each of these elements plays a critical role in how effectively researchers can work with NSPCs, and addressing these challenges may significantly enhance the research outcome.
Characterization Limitations
Characterizing non-spherical particles is inherently complex due to their diverse shapes and sizes. Traditional techniques often fall short when assessing the multifaceted nature of these systems. For example, methods like dynamic light scattering are effective for spherical particles but struggle with irregular geometries. This reliance on inadequate characterization methods can lead to a poor understanding of the physical and chemical properties of NSPCs.
Characterization of NSPCs is crucial for their applications across fields such as materials science and biology.
Considerations such as particle interactivity and morphology must be accounted for to ensure accurate results. Advanced techniques, such as atomic force microscopy and scanning electron microscopy, show promise in addressing some limitations, yet they require specialized knowledge and instrumentation, which may not be readily available to all research facilities. This disparity can hinder collaborative research efforts and slow down scientific advancement in the field.
Standardization Issues
In the realm of NSPC research, standardization is a recurring theme. The lack of standardized methods for the synthesis and characterization of non-spherical particles introduces significant variability in research findings. Without a common framework, results from one study may not be directly comparable to another, complicating the accumulation of knowledge.
Standard operating procedures need to be established to guide researchers in creating and analyzing NSPCs. Standardization would enhance reproducibility and ensure that findings are reliable and interpretable. This process entails collaboration across institutions and fields to develop protocols that can be universally adopted. By addressing standardization issues, the research community can work towards enhancing the collective understanding of non-spherical particulates.
Data Interpretation Challenges
Interpreting the data derived from NSPC studies raises its own set of challenges. The intricate nature of non-spherical particles often means that simple statistical models fail to capture the reality of their behavior. This reality can result in misleading conclusions that may hinder further exploration or application.
Researchers must grapple with how to accurately model the interactions of non-spherical particles within their environments. This complexity poses significant obstacles to deriving predictive models useful in practical applications. For example, in drug delivery systems, the behavior of non-spherical particles in biological systems is often uncertain. This uncertainty raises questions about their efficacy and safety.
Future Directions
The exploration of non-spherical particulate systems (NSPCs) continues to evolve, creating essential pathways for advancements across various scientific fields. As researchers deepen their understanding of NSPCs, emerging insights suggest transformative applications and novel potential. This section focuses on the evolving landscape of research, the innovative applications being developed, and the significance of interdisciplinary collaborations.
Emerging Research Trends
Recent years have shown increased interest in identifying and pursuing emerging research trends in NSPCs. Several trends are evident:
- Nanomaterials: Research is increasingly shifting toward the manipulation of non-spherical nanoparticles. This can enhance properties such as catalytic activity and drug delivery efficacy.
- Dynamic Systems: There is growing attention on the behavior of NSPCs in dynamic environments. Understanding flow, stability, and distribution patterns of these particles can lead to advancements in various applications.
- Biomimicry in Design: Increasingly, scientists are looking at nature as a model for designing NSPCs. This biomimetic approach can offer novel solutions in product development, particularly in the biomedical field.
These trends indicate a promising future for NSPCs, where knowledge is applied creatively to solve complex scientific problems.
Innovative Applications
The application of NSPCs is expanding into diverse fields, revealing unique capabilities that were previously underexplored. Some notable innovative applications include:
- Smart Drug Delivery Systems: NSPCs facilitate targeted drug delivery, improving effectiveness while reducing side effects. Techniques like functionalization allow drugs to attach specifically to these particles and release them at precise locations.
- Advanced Sensors: Innovative sensors using NSPCs can detect minute chemical changes, enhancing early diagnosis in medical conditions. Their unique shapes provide increased surface area for better interactions with target molecules.
- Energy Storage: NSPCs used in battery technology can enhance energy density and performance. Understanding particle morphology contributes to better charge-discharge cycles and overall efficiency.
These applications underline the multi-faceted roles NSPCs can play in enhancing both technology and health.
Interdisciplinary Collaborations
Future directions in NSPC research heavily depend on a collaborative approach. Engaging experts from intersecting disciplines brings various dimensions to the study of NSPCs. For example:
- Material Science: Collaborations can lead to the design of new materials by understanding how particle shapes affect their properties.
- Biology: Working with biologists can yield breakthroughs in understanding how NSPCs interact with biological systems, facilitating innovations in drug design.
- Computational Science: Working with computational scientists helps in modeling interactions of NSPCs, leading to predictions that can guide experimental efforts.
This strong emphasis on interdisciplinary collaboration is key for maximizing the potential of NSPCs.
In summary, the study of NSPCs holds immense potential. Addressing emerging trends, innovative applications, and fostering interdisciplinary collaborations can ultimately lead to ground-breaking advancements, impacting multiple domains of science and technology.
The future of NSPC research appears bright. Its implications stretch broadly, influencing areas from healthcare to environmental science.
End
The conclusion serves as a pivotal reflection of the entire discourse on non-spherical particulate systems (NSPCs). This section aggregates insights and lessons learned throughout the article, emphasizing the critical elements that underpin our understanding of NSPCs. It does not merely summarize but also highlights the intricate web of interactions, characteristics, and applications that make NSPCs a focal point of contemporary scientific inquiry.
Summary of Key Insights
Throughout the article, several key insights emerge, crucial for grasping the complexity of NSPCs:
- Diversity of Forms: NSPCs exhibit varied shapes and sizes, which profoundly influences their behavior in different environments. This diversity can lead to unique physical and chemical properties that can be exploited across various applications.
- Characterization Techniques: Effective characterization is essential for understanding non-spherical particles. Techniques such as transmission electron microscopy and dynamic light scattering are integral in revealing the structural and dynamic aspects.
- Interdisciplinary Applications: The implications of NSPCs span multiple fields including biology, material science, and environmental studies. Their utilization in drug delivery systems and pollution remediation showcases their versatility.
"Understanding the multifaceted nature of NSPCs enables researchers to innovate beyond traditional limits and apply these particles benefits in novel ways."
Integrating these insights is paramount for advancing research and applications involving NSPCs.
Implications for Future Research
As we look towards the future, none of the findings can be disregarded. There exist numerous implications for future research stemming from our analysis of NSPCs:
- Innovative Techniques: Continued development of advanced characterization methods will likely yield deeper insights. Enhanced imaging and measurement techniques could allow for real-time observation of NSPCs in complex media.
- Theoretical Models: Developing more sophisticated theoretical models can help predict behavior of NSPCs in varied conditions. This prediction capability will be crucial for optimizing their applications.
- Sustainability Considerations: Future studies should emphasize the environmental impact of NSPCs. Research into biodegradable options or environmentally friendly fabrication techniques represents a significant opportunity to contribute sustainability in material science.
In summary, as NSPCs continue to garner attention in scientific and engineering circles, the potential for groundbreaking discoveries remains substantial. Ongoing investigation into their properties, interactions, and potential applications will further illuminate their role within various disciplines, fostering innovation and technological advancements suitable for tomorrow's challenges.