Protein Crosslinking: Mechanisms and Applications
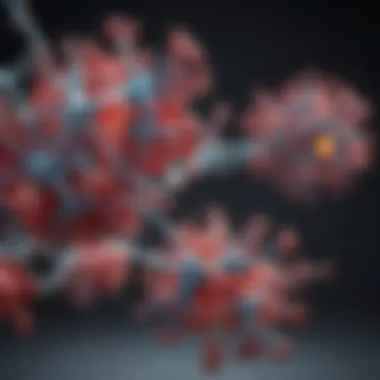
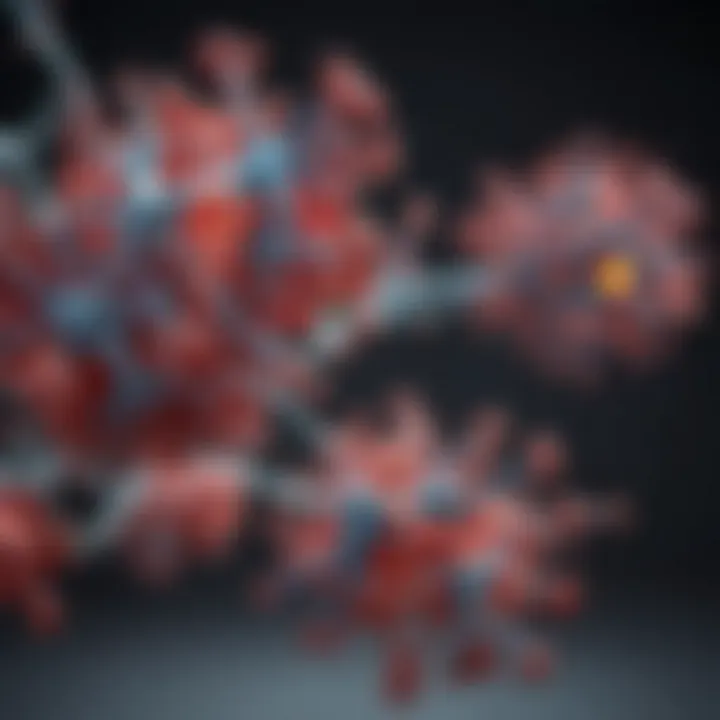
Intro
Protein crosslinking is a fascinating and multifaceted area of study that underpins many biological and industrial processes. In the simplest terms, it involves the creation of bonds between protein molecules, which can profoundly influence their structure, stability, and function. This topic becomes increasingly relevant in an age where biomolecular applications are at the forefront of scientific innovation. From enhancing the durability of materials to advancing therapeutic strategies in medicine, understanding the mechanisms and implications of protein crosslinking is essential for students, researchers, and professionals alike.
As we dive into this complex subject, readers will gain insight into the foundational mechanisms behind protein crosslinking, explore various techniques used to achieve it, and review its applications across multiple disciplines. The discussion further encompasses current trends and implications, highlighting how advancements in research methodologies are shaping our understanding of protein interactions and their significance in contemporary science.
Intro to Protein Crosslinking
Protein crosslinking is a fundamental biochemical process that is gaining immense attention in various scientific fields. The importance of protein crosslinking cannot be overstated, especially considering its vast implications in biology, medicine, and biotechnology. It involves the formation of stable linkages between protein molecules or within a single protein, which ultimately affects their structure and function. As such, an in-depth understanding of this phenomenon is crucial for students, researchers, educators, and professionals alike.
One pivotal benefit of protein crosslinking is its ability to enhance the stability of proteins. Stronger bonds between amino acids often lead to proteins that are more resilient, especially in harsh environments. This aspect is invaluable for developing industrial enzymes, where maintaining functionality under variable conditions is essential. Furthermore, crosslinking serves as a versatile tool in creating biomaterials. These materials find application in areas ranging from drug delivery systems to wound healing and tissue engineering, demonstrating the practical importance of mastering this process.
As we delve deeper into this article, we will also consider the various facets of protein crosslinking, such as its mechanisms, techniques, and applications. The urgency to comprehend these elements is heightened by the increasing complexity of biological systems and the demands of innovative medical solutions. By painting a comprehensive picture of protein crosslinking, this article aims to equip readers with the knowledge necessary to harness these mechanisms for future advancements.
"Understanding how proteins interlink not only gives us insight into fundamental biology but also paves the way for groundbreaking applications in medicine and industry."
Whether you are a seasoned researcher or a student exploring the intricacies of biochemistry, grasping the nuances of protein crosslinking offers numerous pathways to impact both scientific inquiry and practical application.
Fundamentals of Protein Structure
Understanding the fundamental aspects of protein structure is crucial when diving into protein crosslinking. This foundational knowledge provides insight into how proteins behave, how they can be manipulated, and why their interactions are significant in various applications.
Understanding Protein Folding
Protein folding is a complex process where a polypeptide chain acquires its functional three-dimensional shape. This folding is not a random affair; rather it is driven by a series of interactions among the amino acids in the chain. The orderly arrangement of these interactions ensures that proteins are properly shaped to perform their functions. If proteins do not fold correctly, they can be rendered useless or even harmful, as seen in many diseases. The ability to alter or stabilize these folded structures through crosslinking techniques can enhance protein function while providing valuable insights into its behavior in different environments.
Types of Protein Structures
Protein structure can be categorized into four main tiers, each offering unique perspectives on how proteins function and interact. Let's dissect this classification:
Primary Structure
The primary structure refers to the linear sequence of amino acids in a protein. This sequence is crucial as it determines how the protein will fold into its complex three-dimensional form.
- Key Characteristic: The precise order of amino acids, dictated by the genetic code, gives rise to the protein's unique properties.
- Significance: It is a beneficial choice for this article because any variation can lead to significant functional differences in proteins.
- Unique Feature: The unique aspect of the primary structure is that it is unchangeable once synthesized, making it vital for studying mutations or modifications over time. However, its limitations lie in the fact that it doesn't provide any information about the protein's folded state or functionality.
Secondary Structure
The secondary structure involves localized folding patterns within a protein, typically into alpha helices and beta sheets.
- Key Characteristic: Hydrogen bonds stabilize these structures, making them integral to the protein's overall shape.
- Significance: This aspect of protein structure is popular for this article since these formations play a critical role in the protein's stability and functionality.
- Unique Feature: Secondary structures can easily be affected by environmental factors, like pH and temperature; this characteristic allows for exploring how crosslinking may reinforce or negate these changes.
Tertiary Structure
The tertiary structure refers to the overall three-dimensional shape of a single protein molecule, formed by further folding and interactions among the secondary structures.
- Key Characteristic: This structure is stabilized by various interactions such as hydrogen bonds, ionic bonds, and hydrophobic interactions.
- Significance: A deep understanding of tertiary structure is essential for this article because it directly relates to the protein's activity and its crosslinking potential.
- Unique Feature: Tertiary structures can be exceptionally intricate, and how a protein folds into this configuration can significantly affect disease processes. However, misfolding in this form can lead to dysfunctional proteins, marking a disadvantage in research.
Quaternary Structure
The quaternary structure involves the assembly of multiple polypeptide chains into a single protein complex. This form illustrates how proteins can come together and how their interactions function.
- Key Characteristic: It relies on the stabilization of interactions between separate polypeptide chains.
- Significance: It is essential in this article as it illustrates collaborative function in proteins that may be involved in crosslinked formations, which enhances functional diversity.
- Unique Feature: Quaternary structures can exhibit cooperative behavior; changes in one subunit can affect the others, which is a point of interest when considering crosslinking’s effects. Nevertheless, the complexity adds a layer of difficulty in studying these arrangements during protein analysis.
Understanding these structures is paramount. They are the groundwork on which protein interactions and modifications, such as crosslinking, are built. Recognizing these foundational elements enables more profound insights and applications across various fields, cementing the importance of protein structure in biological and industrial contexts.
Mechanisms of Protein Crosslinking
Understanding the mechanisms of protein crosslinking is a pivotal aspect of this study. It lays the groundwork for grasping how and why proteins can be modified to enhance their stability, functionality, and applicability across various domains. Protein crosslinking involves a variety of methods, both chemical and biological, each with its unique features and capabilities. By exploring these mechanisms in detail, we can uncover their roles in experimental setups, industry practices, and therapeutic developments.
Chemical Methods
Chemical methods are perhaps the most widely acknowledged strategies in protein crosslinking. They can be broken down primarily into covalent crosslinking and non-covalent interactions, both playing crucial roles in structural biology and biochemistry.
Covalent Crosslinking
Covalent crosslinking refers to the formation of stable bonds between proteins through covalent interactions. This technique has a reputation for strength and permanence, which makes it a popular choice in numerous applications. The hallmark characteristic of covalent crosslinking is its ability to create strong, irreversible connections that can significantly enhance the durability of protein structures.
One of the standout features of this approach is its versatility—different reagents can be employed to facilitate target-specific linking. For instance, agents like glutaraldehyde are often used due to their ability to form links with amino acid residues, stabilizing the protein structure and preventing denaturation.
However, there are disadvantages to consider. While covalent crosslinking is effective, it can also lead to unwanted changes in protein functionality if not executed carefully. Researchers must strike a balance, ensuring that the desired linkage does not interfere with the protein’s biological activity.
Non-covalent Interactions
In contrast to covalent techniques, non-covalent interactions involve weaker forces such as hydrogen bonds, ionic interactions, and hydrophobic effects. These interactions, while less permanent, have their own special features that make them invaluable in certain contexts. Non-covalent crosslinking is often beneficial when reversible binding is desired. This reversibility can be key in dynamic biological systems, allowing proteins to form and break connections in response to physiological changes.
Such methods are less likely to alter a protein's inherent activity since they do not entail permanent modifications. This gentler approach, however, can also be a double-edged sword, as the weaker interaction might lead to the dissociation of proteins under unfavorable conditions.
Biological Processes
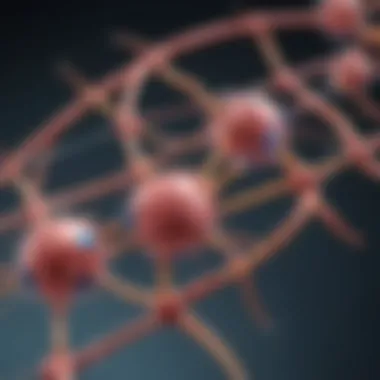
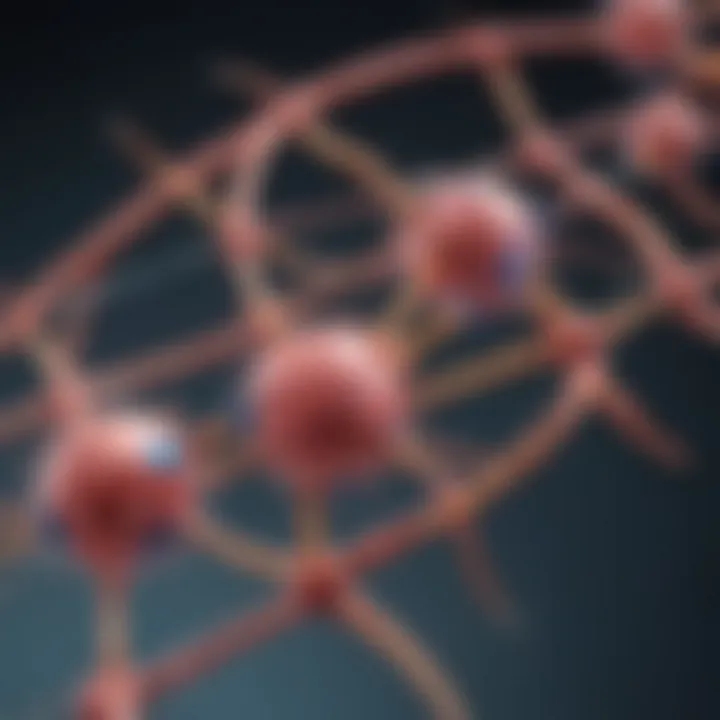
Biological processes associated with protein crosslinking highlight the natural mechanisms utilized by organisms. Here, we can delineate between enzymatic and non-enzymatic forms of crosslinking, each serving significant roles in biological function.
Enzymatic Crosslinking
Enzymatic crosslinking employs specific enzymes to mediate the formation of crosslinks between proteins, typically creating stronger and more specific associations. A defining attribute of this method is the efficiency and selectivity that enzymes provide. Since these biological catalysts work under mild conditions, they often do not disrupt the structural integrity of proteins.
An example can be found in transglutaminases. These enzymes promote the formation of covalent bonds between glutamine and lysine residues, which can enhance the mechanical properties of proteins, especially in tissues. While enzymatic crosslinking is generally more nuanced, its complexity may result in longer reaction times and the necessity for specific conditions to achieve desired outcomes.
Non-enzymatic Crosslinking
Non-enzymatic crosslinking occurs spontaneously in biological contexts, driven by various chemical reactions. For example, oxidative reactions can lead to crosslinking between protein side chains; this method is significant in the aging of tissues and conditions such as fibrosis. One notable characteristic of this process is its ability to occur without the requirement for catalytic agents, thus gaining an edge in simplicity.
However, non-enzymatic crosslinking often lacks the precision of enzymatic methods. The random nature of these links can lead to unpredictability in the final protein structure, potentially compromising functionality. Consequently, while straightforward, non-enzymatic methods must be approached with caution to avoid undesired outcomes in protein behavior.
"Understanding the mechanisms behind protein crosslinking is essential for enhancing protein properties, which is fundamentally significant in applications from drug delivery to biomaterials."
This overview of mechanisms sheds light on the complex interplay between different methods, highlighting their strengths and pitfalls as we navigate the intricate landscape of protein crosslinking. The careful selection of these techniques based on desired outcomes will ultimately drive effective applications in research and industry.
Techniques for Protein Crosslinking
The realm of protein crosslinking encompasses a variety of techniques, each serving a distinct purpose and contributing to the overall understanding and application of protein behavior. Various methods utilized in protein crosslinking enable researchers to manipulate protein structures, and to gain insight into their functionality. As we dig deeper, the significance of these techniques becomes evident, impacting fields ranging from biotechnology to medicine.
Crosslinking Agents
Glutaraldehyde
Glutaraldehyde is recognized as a robust agent widely used for protein crosslinking in laboratories. Its main feature lies in its ability to react with amino groups on proteins, forming stable covalent bonds. It is particularly favored for its efficiency and relatively low cost, making it a go-to choice in research. It’s like having a universal tool—versatile and applicable in various scenarios including tissue fixation and enzyme immobilization.
However, the use of Glutaraldehyde does come with a catch. The reactivity that makes it effective can also lead to unwanted side reactions. Thus, while it significantly contributes to crosslinking and stability of proteins in different materials, careful handling and optimization are crucial to avoid these side effects.
Dithio(2-nitrobenzoic acid)
Dithio(2-nitrobenzoic acid) stands tall in the landscape of crosslinking agents, mainly due to its specificity in targeting disulfide bonds within proteins. This specificity allows for selective crosslinking that can help elucidate protein interactions and conformations in a more refined manner. It’s almost like a sniper's precision, enabling researchers to delve deeper into the structural dynamics of proteins.
One of its notable advantages is that it is less reactive with other functional groups, minimizing non-specific binding. However, its controlled nature can mean that reactions take longer to complete. In the grand scheme of crosslinking, this trade-off is often justified by the quality and relevance of data obtained.
Multi-functional Reagents
Multi-functional reagents boast a broad reactivity profile, allowing them to form diverse linkages with multiple proteins simultaneously. This unique characteristic makes them incredibly useful for assembling complex protein assemblies. With the ability to facilitate intricate networks, they effectively push the boundaries of what can be achieved in protein engineering.
As an added bonus, these reagents can often be modified to tailor their specificity and reactivity, which gives researchers a toolbox of options when designing experiments. However, this flexibility can also pose challenges; achieving the desired balance between specificity and reactivity often requires extensive optimization and a good grasp of the underlying chemistry at play.
Analytical Techniques
Mass Spectrometry
Mass spectrometry (MS) has established itself as an indispensable analytical technique in the realm of protein research. This method excels in identifying and quantifying proteins and their modifications, making it an ideal companion for studying crosslinked complexes. The key feature of MS is its ability to provide high-resolution results that can reveal minute details about molecular weights and structures.
Its benefit lies not just in its sensitivity, but in its versatility across various types of samples. The ability to analyze a vast range of proteins adds to its allure, making mass spectrometry a popular choice in the research community. Yet, one must keep in mind the inherent complexity of interpreting the results, which can require significant expertise to navigate.
NMR Spectroscopy
Nuclear magnetic resonance (NMR) spectroscopy provides a window into the dynamic world of protein interactions. Its core advantage is being able to observe proteins in their native environments, which is crucial for understanding biophysical properties. With the capability to explore tertiary and quaternary structures, NMR facilitates an intimate look at how crosslinking influences protein conformations over time.
The downside, however, is that this technique can require large quantities of highly pure protein samples, which can be a costly hurdle in some experiments. However, when the stars align, the insights gleaned from NMR can greatly enhance our understanding of complex biological functions.
Fluorescent Probes
Finally, fluorescent probes usher in a new era of real-time monitoring of protein interactions and crosslinking events. These probes are adept at tagging specific sites on proteins, allowing researchers to visualize dynamic processes with an impressive degree of clarity. The key characteristic of fluorescent probes is their capability to provide immediate feedback on crosslinked proteins in living systems.
Their unique prowess lies in functionalizing specific areas of proteins, making them an attractive choice for studying interactions in complex cellular environments. Despite their advantages, researchers must manage the challenges associated with potential background noise and signal overlap, which can complicate data interpretation.
The techniques used for protein crosslinking not only enable analysis but also foster innovation in solving biological puzzles.
Applications of Protein Crosslinking
In recent years, the significance of protein crosslinking has shot through the roof, branching out into multiple fields like biotechnology, medicine, and food science. This versatility is one of the major reasons it’s being researched more than ever; it holds the potential to improve processes and products across various industries. The focus here revolves around how protein crosslinking not only enhances functionality but also plays a role in addressing critical challenges in these domains.
Biotechnology
Enzyme Immobilization
When it comes to enzyme immobilization, protein crosslinking can be a game changer. Enzyme immobilization involves attaching enzymes to a solid support, allowing for their reuse and stability in multiple cycles of a reaction. This technology is pivotal for continuous processes, such as in industrial biocatalysis, where efficiency is essential. A key characteristic that stands out is its ability to significantly enhance the durability of enzymes under varying environmental conditions, prolonging their active lifespan.
Utilizing crosslinking agents like glutaraldehyde enables firm binding of enzymes to supports which indeed keeps them from leaching into the reaction medium. Yet, on the other hand, certain methods might lead to loss of enzyme activity, hence precision in selecting the right parameters is key for maximizing the benefits.
- Advantages of Enzyme Immobilization:
- Disadvantages of Enzyme Immobilization:
- Enhanced stability
- Reusable enzymes
- Easier product separation
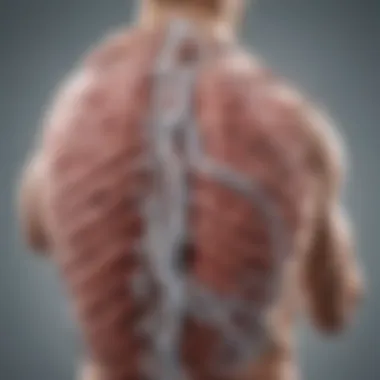
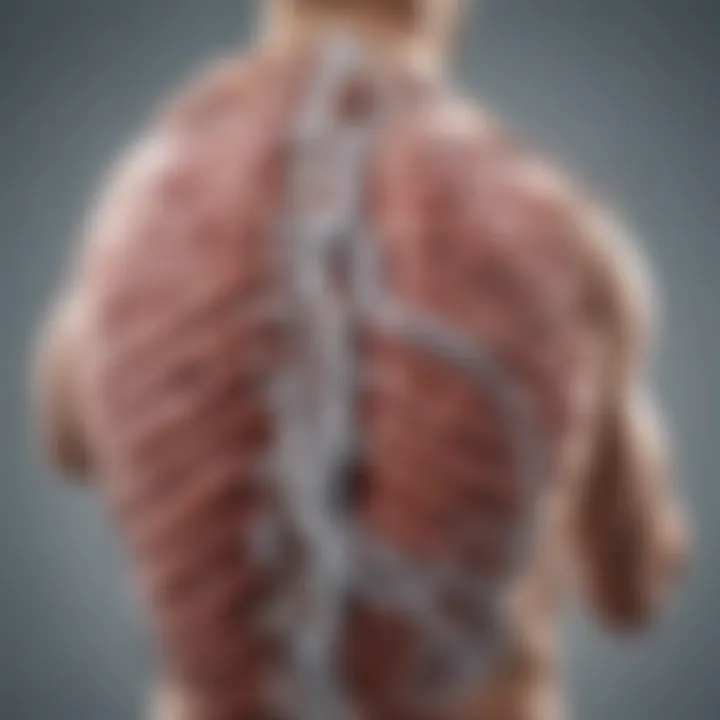
- Potential loss of activity
- Costly initial setup
Biomaterials Development
Turning to biomaterials development, crosslinking plays a crucial role in tailoring the mechanical properties of synthetic and natural polymers. The versatility of crosslinked proteins leads to the creation of materials that can mimic the properties of human tissues. This characteristic makes crosslinked biomaterials particularly enticing for researchers looking to create scaffolds for tissue engineering or regenerative medicine.
A unique feature is the ability to control degradation rates and mechanical strength by adjusting the crosslinking density, applying varying methods of crosslinking, and using different agents. This tunability can make these biomaterials exceptional candidates for specific applications, whether aiming for fast-degrading gelatinous support for soft tissue repair or durable scaffolds for bone regeneration.
- Advantages of Biomaterials Development:
- Disadvantages of Biomaterials Development:
- Customizable properties
- Biocompatible options
- Sustainable materials
- Complexity in synthesis
- Regulatory hurdles
Medical Applications
Drug Delivery Systems
Moving into the medical applications realm, the development of drug delivery systems leverages crosslinking methods to ensure targeted therapy. By encapsulating drugs within crosslinked carriers, it becomes possible to achieve controlled release, tailored to specific biological conditions. This is especially valuable in cancer therapy where timing and dosage precision can enhance treatment efficacy while minimizing side effects.
A key feature here is how crosslinking can create hydrogels that respond to stimuli like pH or temperature, adjusting release rates of medical compounds accordingly. This adaptability turns out to be a significant benefit in customizing treatments, though potential challenges remain concerning stability and reproducibility of such systems.
- Advantages of Drug Delivery Systems:
- Disadvantages of Drug Delivery Systems:
- Improved bioavailability
- Targeted drug action
- Reduction of side effects
- Complexity in design
- Manufacturing cost
Tissue Engineering
Similarly, tissue engineering heavily relies on protein crosslinking for developing scaffolds that support cell adhesion and growth. The physical architecture of these scaffolds is crucial as it influences cellular behavior, ultimately affecting tissue regeneration processes. Protein crosslinking allows for the creation of three-dimensional structures that facilitate nutrient flow and cell migration, essential factors for effective tissue integration.
Crosslinked matrices can closely mimic the extracellular matrix found naturally within human tissues, appealing for applications in organ transplantation. However, it’s important to consider challenges that might arise, such as immune reactivity and the longevity of the scaffolds once implanted.
- Advantages of Tissue Engineering:
- Disadvantages of Tissue Engineering:
- Scaffold biocompatibility
- Mimics natural tissue properties
- Promotes cell proliferation
- Immunogenic responses
- Difficulties in scaling up production
Food Science
Food Preservation
In the sphere of food science, crosslinking techniques contribute significantly to food preservation. By stabilizing food proteins, crosslinked polymers can enhance the nutritional shelf life and safety of products. A main characteristic is the ability to create hydrocolloids that prevent spoilage and microbial growth, which helps in maintaining the quality and flavor of food products. Such characteristics make it a beneficial choice in an industry focused on reducing waste and extending product availability.
Unique features like increased viscosity and improved texture can positively impact the consumer experience in addition to food safety. However, it’s crucial to be cautious about the right balances as excessive crosslinking might change the sensory attributes of food.
- Advantages of Food Preservation:
- Disadvantages of Food Preservation:
- Prolonged shelf life
- Maintains nutritional value
- Acts against spoilage
- Potential alteration of flavor
- Possible changes in texture
Texture Modification
Finally, turning to texture modification, protein crosslinking allows for the manipulation of texture in food products, impacting overall consumer satisfaction. Alterations to protein textures can lead to products that are more appealing, whether making them more tender or providing a firmer bite. In this light, it’s a popular choice among food formulators looking to differentiate their offerings in a competitive market.
For instance, crosslinking can create a gel-like consistency ideal for yogurts or desserts, enhancing mouthfeel and stability. However, the challenge remains to ensure that any modifications do not adversely affect the original nutritional profile or taste of the product.
- Advantages of Texture Modification:
- Disadvantages of Texture Modification:
- Enhanced mouthfeel
- Customizable product characteristics
- Creative culinary applications
- Potential nutritional compromise
- Risk of consumer rejection due to unfamiliarity
As the applications of protein crosslinking expand, understanding these various contexts illuminates the potential it holds across diverse fields, showcasing its promise and paving the way for innovative solutions to complex challenges.
Implications of Protein Crosslinking
The implications of protein crosslinking reach deep into both biological and industrial realms, shaping the very foundations of how proteins function and interact. Understanding these implications not only highlights the relevance of protein crosslinking in contemporary science but also illuminates its far-reaching effects on health, material stability, and innovation.
Impact on Protein Functionality
When proteins undergo crosslinking, their functionality can be significantly altered. Crosslinking often enhances the stability of proteins, making them more resilient to environmental fluctuations. For instance, proteins that are crosslinked may resist denaturation due to heat or pH changes, allowing them to maintain their functional integrity over a longer period. This enhancement in stability is particularly beneficial in biotechnology applications where enzymes are often immobilized for industrial processes. Optimized functionality due to crosslinking can lead to increased efficiency in assays and reactions, fostering advancements in the field.
However, not all crosslinking is beneficial. Depending on the conditions under which it occurs, crosslinking can inadvertently impede protein functionality. Structural changes might block active sites, potentially leading to a loss of biological activity. This duality emphasizes the importance of context in assessing the implications of protein crosslinking.
Role in Disease Mechanisms
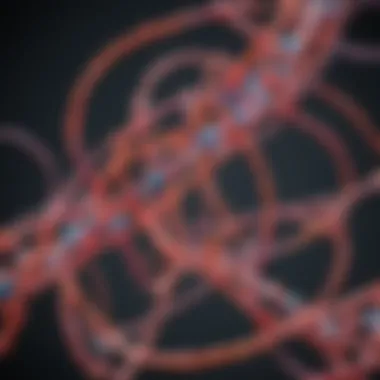
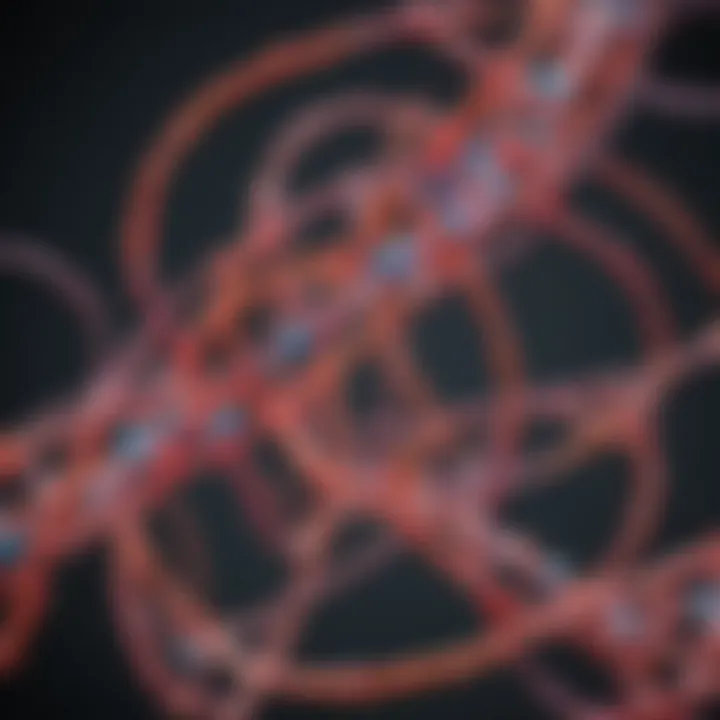
Understanding the role of protein crosslinking in disease mechanisms offers insights into pathologies that arise from malfunctioning or misfolded proteins. Such knowledge can direct research towards innovative therapies and interventions.
Connective Tissue Disorders
Connective tissue disorders often stem from issues in collagen and elastin, primarily proteins that form the structural framework of various tissues. Crosslinking in these proteins is a double-edged sword. On one hand, the crosslinking can provide necessary structural support. On the other hand, excessive or abnormal crosslinking can contribute to stiffness and loss of elasticity, characteristics seen in disorders such as Marfan syndrome.
Key to connective tissue disorders is the impact of enzyme-mediated crosslinking. Enzymes like lysyl oxidase play critical roles in facilitating the formation of covalent bonds between collagen fibers. The unique feature of improper crosslinking in connective tissues is that it results in weakened function of these structural components. As a result, this can lead to debilitating conditions that affect quality of life, emphasizing the necessity to understand these processes in the article.
Neurodegenerative Diseases
Neurodegenerative diseases, particularly Alzheimer's and Parkinson's, also highlight the implications of protein crosslinking. In these conditions, protein misfolding and subsequent crosslinking can lead to the formation of amyloid plaques and neurofibrillary tangles, hallmark features of these disorders.
The key characteristic of neurodegenerative diseases is the accumulation of these aggregates, which disrupt communication between neurons and contribute to cognitive decline. This aspect of disease pathophysiology makes neurodegenerative diseases a focal point for understanding how crosslinked proteins can drive disease progression.
In discussions on these diseases, it's clear that crosslinking poses both challenges and opportunities. While dysfunctional crosslinking can exacerbate conditions, an in-depth understanding of these processes can open doors for potential therapeutic strategies aimed at reducing amyloid formation or targeting crosslinked aggregates for elimination. This demonstrates the value of integrating knowledge of crosslinking dynamics in addressing complex health issues.
Advances in understanding the implications of protein crosslinking can guide future research toward breakthroughs in treatment strategies for various disorders, fostering hope in realms that currently appear challenging.
Recent Advances in Research
Research in protein crosslinking has made remarkable strides in recent years, largely due to technological advancements and an evolving understanding of protein interactions. This growth is crucial for several reasons: it improves the methods used in biological studies, enhances industrial applications, and paves the way for innovative medical treatments. As this field progresses, it sheds light on crosslinking's role in protein functionality, stability, and interactions with other biomolecules.
Innovative Crosslinking Techniques
Click Chemistry
Click chemistry represents a significant turning point in the world of protein crosslinking. This method focuses on swift, reliable, and selective reactions between molecules. A standout attribute of click chemistry is its highly efficient nature, allowing researchers to achieve desired crosslinking with minimal byproducts. In the context of this article, it contributes notably to creating peptide and protein conjugates without cumbersome purification steps. Its straightforward procedure and reproducibility make it a popular choice among scientists who are developing new bioconjugates or probing cellular mechanisms.
Moreover, the unique feature of click chemistry lies in its versatility. Researchers can tailor it to fit a wide array of biochemical environments, making it adaptable for various applications. However, the two-step approach sometimes requires careful optimization, with the potential downside being increased complexity in certain experimental setups. Nonetheless, the benefits it offers in terms of speed and specificity often outweigh these challenges.
Photo-crosslinking
Similarly, photo-crosslinking has emerged as a valuable technique in recent research, distinguished by its ability to use light to induce crosslinking reactions. This method involves the incorporation of photoreactive groups into proteins, which can be triggered to form links upon exposure to specific wavelengths of light. Its key characteristic is the precise spatial and temporal control it offers researchers during experiments. In an elaborate protein study, this specificity can be vital, allowing scientists to observe interactome mapping or investigate biological processes under various conditions without disturbing cellular environments.
Unique to photo-crosslinking is its potential in live-cell imaging and functional studies. The technique permits the investigation of dynamic processes within cells, thus providing insights often left uncovered by traditional methods. However, the necessity for light activation might limit its application in sensitive systems or contexts where prolonged light exposure could lead to cellular damage.
Emerging Applications
As these innovative techniques flourish, new applications continue to emerge, expanding the realms of protein crosslinking. One notable area includes targeted drug delivery systems, where crosslinked proteins can form stable complexes to protect therapeutic agents during circulation in the body. Another application lies in the development of advanced biomaterials, enhancing the characteristics of engineered tissues or scaffolds used in regenerative medicine. The intricate relationship between structural integrity and functional performance makes crosslinking invaluable in these burgeoning fields.
Future Directions in Protein Crosslinking Research
The focus on protein crosslinking is not just about understanding current practices or limitations; it entails envisioning the trajectory of this field in the coming years. As we explore Future Directions in Protein Crosslinking Research, it becomes clear that the fusion of diverse scientific disciplines, alongside a careful examination of ethical implications, is essential for the advancement of knowledge and applications related to protein crosslinking.
Potential for Interdisciplinary Research
Interdisciplinary research is becoming a cornerstone in driving innovation within protein crosslinking. By merging insights from biology, chemistry, materials science, and even computational modeling, researchers can create a more unified understanding of protein behavior. This collaboration can leverage cutting-edge technologies and methods, pushing the envelope not just in academia but in industrial applications as well.
For instance:
- Biochemical insights can enhance the design of crosslinking agents, optimizing their effectiveness and specificity.
- Materials science can contribute to the development of novel biomaterials that incorporate crosslinked proteins, enabling applications from drug delivery to regenerative medicine.
- Computational biology can facilitate predictive modeling for protein interactions, allowing for rational design of crosslinking strategies.
Imagine a scenario where a team of biochemists, engineers, and data scientists collaborate to tackle a disease. They combine their knowledge to create new protein formulations that bind with cellular receptors, enhancing therapeutic efficacy. This kind of synergy embodies the future of research in the protein crosslinking landscape, where boundaries blur and collective expertise propels breakthroughs that would be elusive in isolation.
Ethical Considerations
As the horizons of protein crosslinking expand, so too must our attention to the ethical implications that accompany these advancements. The potential for manipulation of biological systems raises critical concerns. For all the promise that comes with innovative techniques and applications, there are considerations about safety, consent, and the broader impact on life.
- Safety: Ensuring the safety of new crosslinking agents and their uses is paramount. Toxicity profiles must be thoroughly assessed, particularly for biomedical applications.
- Consent and Transparency: In various settings, especially in medicine, obtaining informed consent becomes vital, particularly if genetic alterations are involved in crosslinking methodologies.
- Ecosystem Impact: The ecological ramifications of employing synthetic crosslinked proteins in food science and environmental management need a clear framework to avoid unintended consequences.
It is imperative that researchers engage with ethicists, regulatory bodies, and community stakeholders to navigate these complex issues. The drive for innovation should never outpace the safeguards that protect individuals and our environment. In promoting an open dialogue about these ethical considerations, the field of protein crosslinking can cultivate a responsible research culture that prioritizes human and environmental welfare.
"As the science of protein crosslinking evolves, we must remember that innovation without ethics is a ship without a compass."
Epilogue
The conclusion section wraps up the intricate discussions presented throughout this article, emphasizing the multifaceted nature of protein crosslinking. It's not merely a scientific curiosity; it's a crucial element bridging various disciplines and bringing significant implications within biotechnology, medicine, and materials science. Understanding protein crosslinking serves as a gateway to enhancing the functionality and stability of proteins, which is paramount in both research and practical applications.
Summary of Key Insights
Throughout this article, we have uncovered various components surrounding protein crosslinking:
- Definition and Mechanisms: This includes both chemical and biological methods, illustrating how unique reactions facilitate crosslinking in different environments.
- Diverse Techniques: We discussed specific reagents like glutaraldehyde and innovative approaches such as click chemistry, which have revolutionized the field.
- Applications Across Fields: The implications of crosslinking span many areas—from enzyme immobilization in biotechnology to tissue engineering in medicine and even food science with preservation techniques.
- Significance of Research: Recent advancements underscore the ongoing evolution within this domain and shine a light on unexplored territories.
Notably, it's essential to comprehend how these insights intertwine with larger scientific paradigms and drive forward research. The interconnectedness of protein crosslinking with diseases, particularly in areas such as neurodegenerative disorders, mirrors the broader implications this technology holds in human health.
Implications for Future Research
As we look ahead, several future research pathways emerge with promising potential:
- Interdisciplinary Collaboration: The integration of different scientific domains can unearth novel applications and innovative methodologies, potentially enhancing the effectiveness and scope of protein crosslinking.
- Ethical Considerations: With advances in biotechnology, there's a pressing need to address ethical concerns surrounding genetic manipulation and its broader impacts on society. Striking a balance between innovation and ethical responsibility is crucial.
- New Techniques and Agents: Developing more sophisticated crosslinking agents and refining existing techniques could elevate the efficiency and specificity of protein interactions.
Future research could also explore the kinetic and thermodynamic perspectives of crosslinking, leading to a better understanding of these reactions' underlying principles. In summary, the landscape of protein crosslinking remains ripe for exploration, with its implications touching on both the fundamental science and its practical applications.
As researchers and practitioners continue to push the boundaries, the insights gleaned from these investigations will contribute significantly to the broader scientific narrative, driving the engines of innovation and application forward.