Exploring the Diverse World of Permanent Magnets
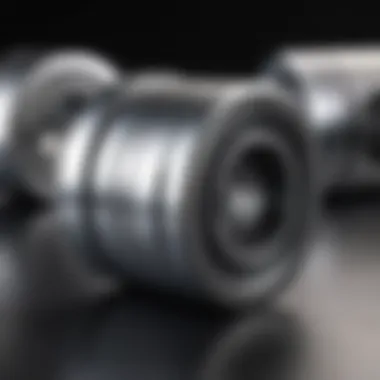
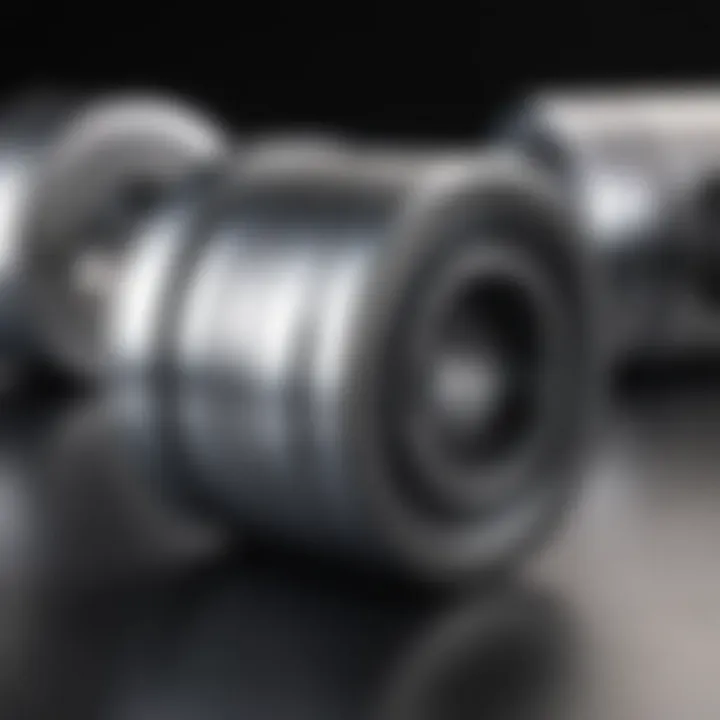
Intro
Understanding permanent magnets is essential in numerous fields. From electronics to industrial applications, their role cannot be overstated. Permanent magnets are materials that maintain a persistent magnetic field without the need for an external power source. This article delves into the various types of permanent magnets, investigating their properties, production methods, and real-world applications. By analyzing the latest advancements in magnet technology, we are better equipped to appreciate their significance in today's world.
Research Overview
This section outlines the key findings of ongoing research in permanent magnets and the methodologies used to gather this knowledge.
Key Findings
- Permanent magnets can be classified mainly into three categories: ferrite magnets, neodymium magnets, and samarium-cobalt magnets.
- Ferrite magnets are cost-effective and suitable for everyday applications such as loudspeakers and refrigerator magnets.
- Neodymium magnets offer superior strength and are widely used in advanced devices such as motors and sensors.
- Samarium-cobalt magnets are resistant to corrosion and are ideal for high-temperature environments, often found in aerospace applications.
Study Methodology
Research focuses on diverse methodologies including experimental setups, field studies, and manufacturer case studies. It combines laboratory tests that measure magnetic properties with market analysis to assess application trends.
Background and Context
This segment provides a historical perspective on permanent magnets and highlights current trends within the field.
Historical Background
The study of magnets dates back thousands of years, with the discovery of lodestones in ancient China. Over the centuries, improvements in magnet manufacturing emerged alongside technological advancements. In the 20th century, neodymium magnets were developed, revolutionizing the magnet industry due to their incredible strength.
Current Trends in the Field
Today, trends in permanent magnet technology emphasize sustainability and efficiency. Advances in materials science are leading to the development of ecologically friendly magnets, reducing reliance on rare earth materials. Researchers explore new alloys and composites, aiming to enhance magnetic performance while minimizing environmental impact.
"Permanent magnets are critical in modern technology. Their continued development shapes the future of numerous industries."
Prolusion to Permanent Magnets
Permanent magnets are materials that can maintain a consistent magnetic field without the need for an external power source. Their magnetic properties emerge from the alignment of their atomic structure, which allows them to retain magnetism after being magnetized.
Understanding permanent magnets is crucial for those in technology, engineering, and materials science. The significance of this subject lies in its application across various industries, from consumer electronics to healthcare. They play a vital role in the operation of devices such as motors, generators, and electronic sensors.
The exploration of permanent magnets also encompasses their classification. Different types of magnets exhibit unique characteristics which determine their suitability for specific applications. This guide will delve into those classifications and highlight not just the types, but also the interactions of these magnets with their environments.
Defining Permanent Magnets
Permanent magnets are defined by their ability to retain magnetism over time. Unlike temporary magnets that lose their magnetism when removed from a magnetic field, permanent magnets require no maintenance or power usage to sustain their performance once they are manufactured. Their magnetic field is caused by the alignment of magnetic domains within the material. The choice of material greatly influences the strength and durability of the magnet. Significant examples include ferrites, neodymium, and samarium cobalt.
Importance of Permanent Magnets in Technology
The role of permanent magnets in technology cannot be overstated. They are essential components in many devices used in everyday life. In consumer electronics, for instance, they are found in speakers, microphones, and hard disk drives. Their ability to produce strong magnetic fields enables these devices to function efficiently.
Moreover, in industrial applications, permanent magnets are utilized in motors, conveyor systems, and separation processes. Magnetic resonance imaging (MRI) machines in medical settings rely heavily on powerful permanent magnets for diagnostic imaging.
"Permanent magnets are indispensable in technology, serving both functional and transformative roles in various applications."
The impact of permanent magnets extends beyond conventional uses. Their importance is growing as researchers seek to enhance their efficiency and reduce material costs. Understanding the fundamental principles and classifications of these magnets will provide insights into their capabilities and inspire innovations in their use.
Classification of Permanent Magnets
Understanding the classification of permanent magnets is crucial for various technological applications and research. By categorizing these magnets, we can identify their distinct properties and functionalities. Each type of magnet serves specific purposes based on its physical characteristics and material composition. Recognizing these differences allows professionals and researchers to select the appropriate magnet for their needs, promoting efficient design in fields ranging from electronics to healthcare.
Overview of Magnet Types
Permanent magnets can be broadly classified into three main categories: ferromagnetic magnets, ferrimagnetic magnets, and rare earth magnets. Each category exhibits unique properties that affect their performance in practical applications.
- Ferromagnetic Magnets: These magnets are made from materials like iron, cobalt, and nickel. They exhibit strong magnetic properties, which is why they are commonly used in motors, generators, and transformers.
- Ferrimagnetic Magnets: Composed mainly of oxides like magnetite and various metal ions, these materials have less magnetic strength compared to ferromagnetic types. Ferrite magnets are often found in applications requiring non-corrosive materials, such as in electronics and refrigerator magnets.
- Rare Earth Magnets: This category includes neodymium and samarium-cobalt magnets, which are noted for their exceptional strength. Despite their small size, these magnets produce high magnetic fields and find use in various high-tech applications such as mobile phones, speakers, and wind turbines.
The distinction in types is not just academic; it leads directly to practical choices in design and application.
Physical Properties of Permanent Magnets
The physical properties of permanent magnets are critical in determining their performance. Several key characteristics define how these magnets function and their suitability for specific uses.
- Coercivity: This property indicates the ability of a magnet to retain its magnetization after an external magnetic field is removed. High coercivity materials resist demagnetization, making them ideal for applications where stability is essential.
- Remanence: This refers to the magnetization remaining in a magnet after an external magnetic field is applied and then removed. This property is crucial when the magnet needs to retain its magnetic strength over time, like in various sensors.
- Magnetic Energy Product: Measured in Mega-Gauss-Oersteds (MGOe), it signifies the efficiency of a magnet. A higher energy product indicates a stronger magnet, essential for compact and powerful designs.
- Temperature Stability: Some permanent magnets can lose their magnetization when exposed to high temperatures. Understanding this ensures that the selected magnet performs well in varying environmental conditions.
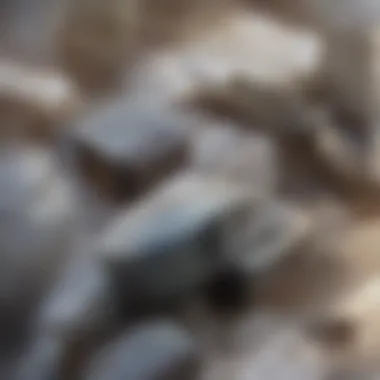
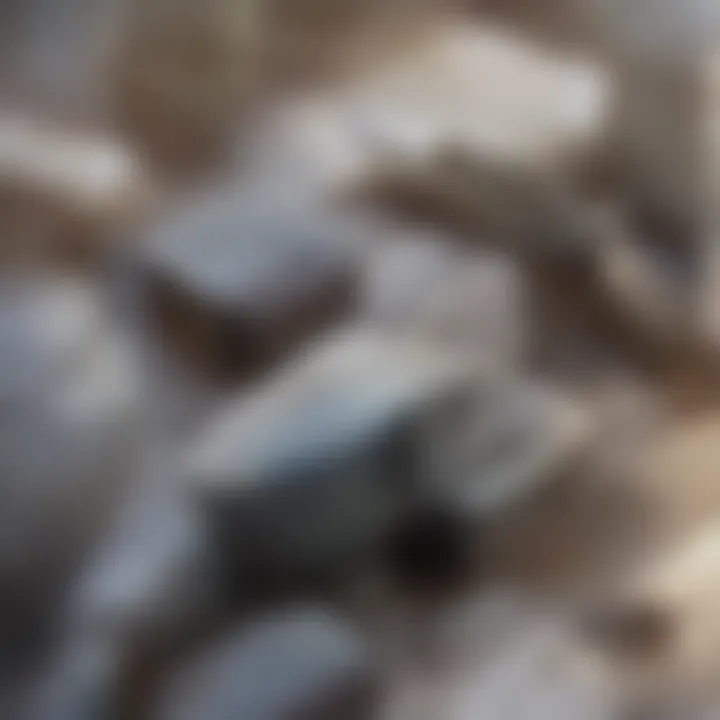
"The classification of permanent magnets is fundamental in ensuring the right choices are made in design and application across industries."
By distinguishing between the types and knowing their physical properties, engineers and designers can optimize performance in applications ranging from consumer products to industrial machinery. Understanding these classifications lays the groundwork for future innovations in magnet technology.
Ferromagnetic Magnets
Ferromagnetic magnets represent a crucial segment of the permanent magnet category. Their unique properties stem from the alignment of internal magnetic domains. This intrinsic ordering is what amplifies their ability to interact with magnetic fields. Understanding these magnets, and their characteristics can shed light on their significance in various applications and technologies.
Characteristics of Ferromagnetic Materials
Ferromagnetic materials exhibit several distinctive characteristics that set them apart from other magnets. These include:
- High Magnetic Permeability: Ferromagnetic materials can easily become magnetized when exposed to an external magnetic field. This is due to their high permeability which allows them to absorb magnetic lines of force.
- Retentivity: Once they have been magnetized, these materials can retain significant amounts of magnetization even after the external magnetic field is removed. This property is critical for applications where consistent magnetic strength is needed.
- Curie Temperature: Each ferromagnetic material possesses a specific temperature called the Curie temperature. Beyond this temperature, the material loses its ferromagnetic properties. Understanding this aspect is important for applications that experience thermal fluctuations.
- Anisotropy: Many ferromagnetic materials show directional dependence in their magnetic properties. This means that their magnetic behavior may vary depending on the direction of the applied magnetic field.
Common Applications of Ferromagnetic Magnets
The applications of ferromagnetic magnets span multiple industries, showcasing their versatility. Some of the key applications include:
- Electric Motors and Generators: Ferromagnetic magnets play a vital role in converting electrical energy into mechanical energy and vice versa. These magnets help create the magnetic fields necessary for motor operation.
- Magnetic Sensors: Devices like reed switches and Hall effect sensors rely on ferromagnetic materials to detect magnetic fields, making them indispensable in various electronic circuits.
- Transformers: The core of transformers is often made from ferromagnetic materials to enhance the efficiency of electrical energy transfer.
- Data Storage Devices: In hard drives, ferromagnetic magnets are used to store data in a magnetic medium.
Ferromagnetic materials are foundational in modern technology, driving the operation of essential devices and systems.
In summary, ferromagnetic magnets are integral to the functionality of numerous applications in both consumer electronics and industrial processes. Their unique properties allow them to be used effectively across a wide range of technologies.
Ferrimagnetic Magnets
Ferrimagnetic magnets play a significant role in the broader category of permanent magnets, offering unique properties valuable across various applications. This type of magnet operates based on the principle of opposing magnetic moments within its structure, leading to distinctive behaviors. Understanding the characteristics and importance of ferrimagnetic magnets contributes to a comprehensive grasp of magnetic materials. These magnets are essential in multiple sectors such as electronics, telecommunications, and industrial applications.
Defining Ferrimagnetism
Ferrimagnetism is a specific type of magnetic ordering found in certain materials. In ferrimagnetic materials, magnetic moments of unequal magnitudes align in opposite directions. This results in a net magnetization due to the imbalance between the opposing moments. The most commonly referenced ferrimagnetic materials are ferrites, which are composed of iron oxide combined with other metal oxides. This configuration allows them to maintain magnetization even in the absence of an external magnetic field. The unique magnetic interactions in these materials make them distinct from both ferromagnetic and paramagnetic materials.
Many ferrimagnetic materials, such as magnetite, are notable for their resistive properties and are utilized in high-frequency applications due to their low eddy current loss. As a result, ferrites are commonly found in transformers, inductors, and magnetic cores. This character gives ferrimagnetic materials an edge in applications requiring stability and efficiency, especially at higher frequencies.
Uses of Ferrimagnetic Magnets in Industry
The industrial use of ferrimagnetic magnets cannot be overstated. Their unique characteristics make them suitable for a variety of applications. Here are some notable uses:
- Electronics: Ferrimagnetic materials are often employed in transformers and inductors where efficiency is crucial. The low losses incurred in their operation contribute significantly to the performance of electronic devices.
- Telecommunications: Ferrites are widely used in antennas and high-frequency devices. They help improve signal quality by reducing interference.
- Magnetic Storage Devices: Ferrimagnetic materials play a role in data storage solutions through their ability to retain magnetic information reliably.
- Sensors: These magnets are also integrated in various sensors, ensuring accurate measurements in industrial processes.
"Ferrimagnetic materials are key components in modern technology, enhancing efficiency and performance across diverse applications."
Rare Earth Magnets
Rare earth magnets are a crucial subject in the study of permanent magnets. Their unique properties stem from the materials they are composed of, which include neodymium, samarium, and cobalt. These magnets stand out for their remarkable strength and stability, exceeding the performance of traditional magnets. In this section, we will discuss two major types of rare earth magnets—neodymium magnets and samarium cobalt magnets. We will also examine their distinct characteristics and the pros and cons of using them in various applications.
Types of Rare Earth Magnets
Neodymium Magnets
Neodymium magnets are perhaps the most well-known type of rare earth magnet. They are notable for their high magnetic strength. This characteristic is due to the specific alignment of neodymium, iron, and boron in their structure, leading to exceptionally strong magnets. Their strength makes them a popular choice in various applications, from consumer electronics to industrial machinery.
A unique feature of neodymium magnets is their ability to maintain a strong magnetic field. This makes them effective for applications where space is a constraint, thus allowing for smaller and lighter devices without compromising performance.
However, neodymium magnets come with some disadvantages. They have a tendency to corrode if not properly coated, typically with a layer of nickel or epoxy. This vulnerability necessitates care in environments with moisture or high humidity.
Samarium Cobalt Magnets
Samarium cobalt magnets offer an alternative to neodymium resistors. Their key characteristic is temperature stability. They maintain their magnetic performance even in high-temperature settings, which can be a major advantage in specific applications like aerospace and military technologies.
These magnets have a unique feature of being more resistant to demagnetization compared to neodymium magnets. This allows them to be used in more demanding environments without losing their magnetism.
On the downside, samarium cobalt magnets can be more expensive to produce than neodymium magnets, which can limit their usage in cost-sensitive applications. Additionally, they are typically somewhat less powerful than neodymium magnets, which can be a drawback for some uses.
Advantages and Disadvantages of Rare Earth Magnets
Examining the advantages and disadvantages of rare earth magnets is essential for understanding their place in current technology.
Advantages:
- High Magnetic Strength: Both neodymium and samarium cobalt magnets provide immense holding power.
- Compact Size: Their magnetic strength allows for smaller designs in products, making them ideal for consumer electronics and other applications that require efficiency.
- Diversity of Applications: From medical devices to motors in electric cars, rare earth magnets are versatile in application.
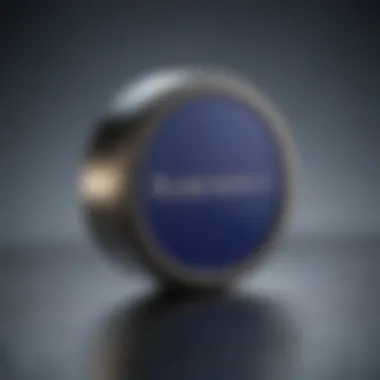
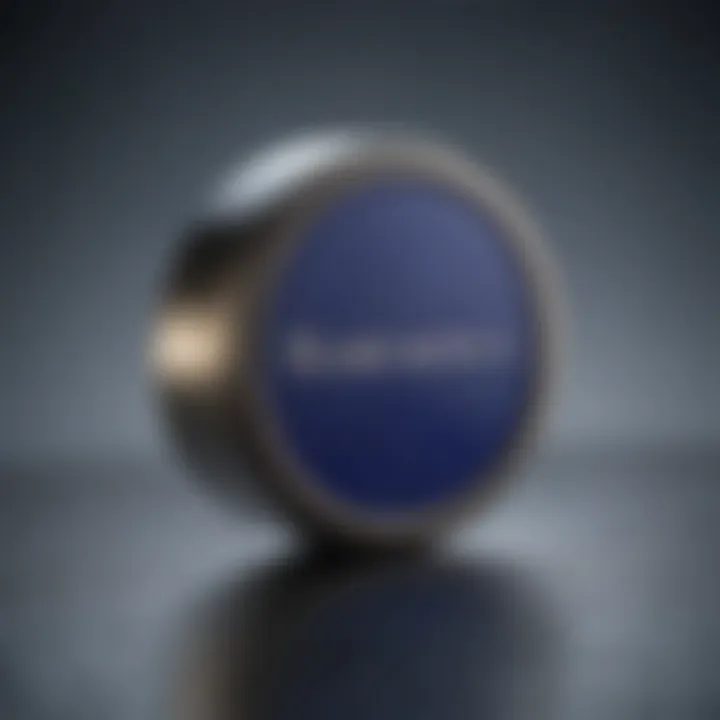
Disadvantages:
- Cost: The production cost of rare earth magnets can be high, which can impact pricing in consumer markets.
- Corrosion: Neodymium magnets can corrode without protective coatings, demanding extra care in design and application.
- Limited Availability: Rare earth elements may become scarce, affecting production.
"Rare earth magnets are a cornerstone of progress in technology, enabling advancements that were not possible before."
Understanding these attributes assists researchers and professionals in making informed decisions in their applications.
Manufacturing Processes for Permanent Magnets
Manufacturing processes for permanent magnets play a crucial role in defining their performance and applications. Understanding these techniques is essential not only for the production of high-quality magnets but also for optimizing costs and enhancing the effectiveness of the final products. Manufacturers must consider various factors such as material characteristics, desired magnetic properties, production efficiency, and environmental considerations. This section explores two significant methods: sintering techniques and bonded magnets production.
Sintering Techniques
Sintering is a versatile process extensively used in the production of permanent magnets, particularly for those made from powders like ferrites and rare earth elements. The process involves shaping magnet powders into a desired form and then heating them in a controlled atmosphere without melting them. This heating allows particles to fuse together, resulting in a strong and dense magnetic material.
Key Points of Sintering Techniques:
- Material Selection: The choice of powder material is critical. Common materials include ferrites and neodymium-iron-boron particles.
- Temperature Control: The temperature must be carefully monitored to avoid excessive grain growth, which can affect magnetic properties.
- Equipment: Specialized furnaces are employed to facilitate the sintering process, often using inert gases to prevent oxidation.
- Post-Sintering Treatment: After sintering, magnets may undergo additional processes, like machining or coating, to enhance their performance and durability.
This method typically results in magnets with high coercivity and remanence, which are essential for various applications from motors to hard disk drives. However, defects such as cracks or porosity can occur if the process is not conducted properly.
Bonded Magnets Production
Bonded magnets are another significant class of permanent magnets, often used in applications requiring complex shapes or low weight. The production of bonded magnets combines magnetic powders with a polymer or resin binder, resulting in a flexible and versatile product.
Key Aspects of Bonded Magnets Production:
- Mixing of Components: Magnet powders are mixed with binding agents. This mixture can be done in different ratios to achieve desired magnetic properties.
- Molding Methods: The combination is then molded under heat and pressure to form the desired shapes, using techniques like compression or injection molding.
- Cooling and Finishing: After molding, the magnets are cooled and may undergo finishing processes like surface treatment for improved adhesive properties.
Bonded magnets are advantageous because they are easier to produce into customized shapes. They tend to be less brittle than sintered magnets, making them suitable for demanding applications, such as automotive and consumer electronics.
"The choice of production technique directly influences the performance characteristics of the permanent magnets, ultimately affecting their efficiency in practical applications."
In summary, understanding the manufacturing processes for permanent magnets enables the production of components that meet specific performance criteria necessary for various applications. Each method, whether sintering or bonding, has its distinct advantages and limitations, which manufacturers must navigate to yield high-quality magnetic materials.
Magnet Performance Metrics
Magnet performance metrics are critical for understanding the effectiveness and functionality of different types of permanent magnets. These metrics determine not only how strong a magnet is, but also how well it performs under various conditions. Knowing these values helps engineers and designers select the right magnets for specific applications, which is essential in sectors ranging from consumer electronics to industrial machinery. Understanding these metrics can also lead to advancements in magnet design and overall technology.
Magnetic Strength Measurements
Magnetic strength measurements refer to the quantification of a magnet's ability to attract or repel magnetic materials. The most common metric for measuring magnetic strength is the magnetic flux density, commonly expressed in teslas (T). One fundamental way to gauge a magnet's strength is by its maximum energy product, measured in megagauss-oersteds (MGOe), which indicates how much energy a magnet can deliver per volume.
Another important metric is remanence, which represents the residual magnetic field a magnet retains after being magnetized. The higher the remanence, the stronger the magnet will be after exposure to an external magnetic field. Also, coercivity describes how resistant a magnet is to becoming demagnetized. These parameters are essential for applications where robustness of magnetic performance is crucial.
"The choice of magnet must be driven by its performance metrics to ensure optimal usability in intended applications."
Temperature Stability of Magnets
Temperature stability is a vital aspect of magnet performance. Permanent magnets can lose their magnetism when exposed to high temperatures. This phenomenon happens because heat increases the vibration of atoms, which in turn affects the alignment of magnetic domains within the material. Each type of permanent magnet has a specified maximum operating temperature.
For instance, neodymium magnets have a lower temperature threshold than samarium-cobalt magnets. Understanding these thermal limits is crucial when selecting magnets for applications exposed to varying temperature conditions, such as electric motors, hard drives, and other critical machinery.
In addition to maximum operating temperatures, it's essential to consider the thermal coefficient of magnetization, which indicates how much a magnet's strength changes with temperature. A low thermal coefficient suggests better stability across temperature variations, making the magnet more reliable in demanding environments.
Proper awareness about magnetic strength and temperature stability is vital in research and application. These insights not only enhance the performance of permanent magnets but also inform future innovations in magnet technology.
Emerging Trends in Permanent Magnet Technology
The landscape of permanent magnets is constantly evolving with newer materials and technologies emerging. These trends are crucial for multiple reasons: they enhance efficiency, reduce cost, and consider environmental sustainability. As industries grow more dependent on advanced magnet technology, the relevance of these trends becomes more pronounced. In this section, we will explore key advancements in material science and sustainability considerations that are shaping the future of permanent magnets.
Advancements in Material Science
Innovations in material science play a pivotal role in the development of permanent magnets. New magnetic materials are being studied and created to improve magnetic performance. For instance, researchers are focusing on new composite materials that blend rare earth magnets with other elements to optimize their properties. This can lead to stronger and lighter magnets that are easier to integrate into various applications.
Adjacent to that, the exploration of nanostructured magnets yields exciting possibilities. These magnets can exhibit vastly enhanced magnetic properties compared to their traditional counterparts. The small scale allows manipulation of material properties at an atomic level, which could revolutionize the efficiency of permanent magnets across industries.
Another focus area is on the discovery of less expensive and more abundant alternatives to rare earth materials. This could alleviate some of the supply challenges currently faced by industries relying on Neodymium and Samarium. By leveraging innovative materials, producers can create a more stable market and reduce costs while providing effective alternatives.
Sustainability Considerations in Magnet Production
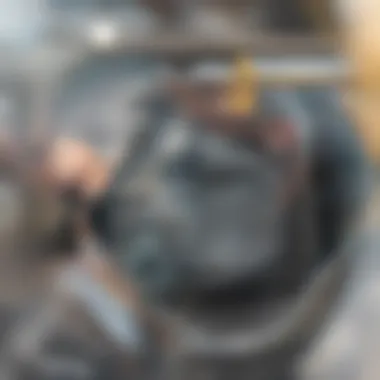
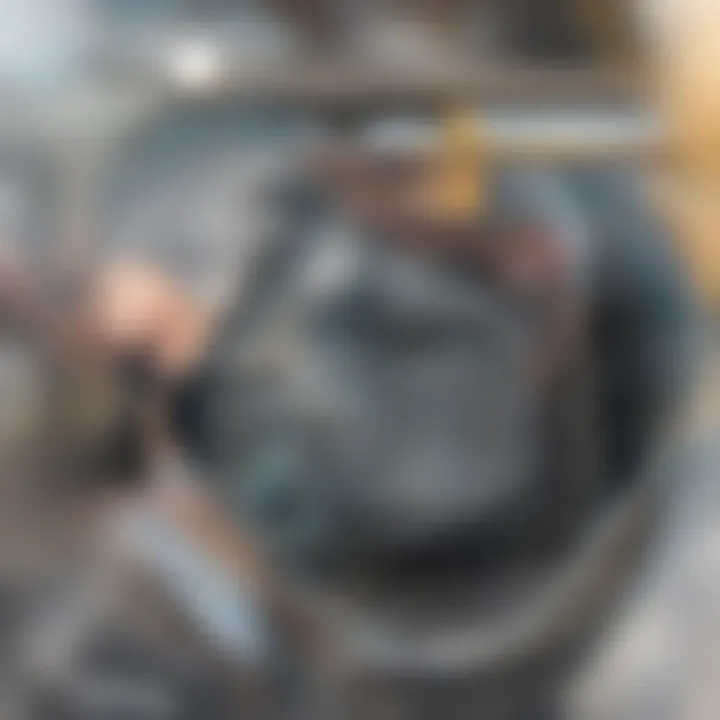
As environmental awareness grows, sustainability in magnet production becomes increasingly vital. The extraction process of rare earth elements raises concerns about ecological impact. Mining these precious materials often leads to habitat destruction and excessive waste. Companies are seeking solutions to minimize this impact.
Adoption of recycling methods for permanent magnets is one promising approach. By reclaiming materials from old electronics, manufacturers can mitigate the need for new mining operations. This not only decreases environmental strain but also recycles valuable resources.
Furthermore, the development of biodegradable magnets is being researched. Although still in early stages, these innovations could drastically change how magnet materials are manufactured, reducing pollution and waste in production.
"Emerging trends in materials science and sustainability in production practices are crucial in making permanent magnets not just more efficient but also environmentally friendly, ensuring their place in the future of technology."
The commitment to sustainable practices and advancement in material science highlights the forward-thinking nature of the industry. This dual focus signifies that the future of permanent magnets can be both effective and responsible, providing benefits that reach beyond mere performance.
Applications of Permanent Magnets
Permanent magnets have significant roles across various industries, enhancing functionality, efficiency, and reliability. Understanding their applications offers insights into technology's dependency on magnetic materials. This article section assesses the practical uses of permanent magnets, facilitating an understanding of their long-term impact on devices and systems. Their ability to maintain a stable magnetic field without external power makes them essential in consumer electronics, manufacturing, and healthcare.
Permanent Magnets in Consumer Electronics
Consumer electronics leverage permanent magnets in numerous ways. From smartphones to loudspeakers, these magnets enhance performance and user experience. For example, in audio devices, permanent magnets are vital components in speakers, where they interact with coils to produce sound. Without them, the sound quality would significantly deteriorate.
Besides that, motors found in devices like electric toothbrushes and gaming controllers benefit from permanent magnets. These motors enable efficient energy conversion, enhancing device longevity and reliability.
Moreover, permanent magnets are crucial in the hard disk drives of computers. They store vast amounts of data and contribute to the overall speed of data retrieval. As technology evolves, the demand for more compact and efficient permanent magnets in consumer devices continues to rise.
Industrial Applications: An Overview
In industrial settings, the versatility of permanent magnets makes them indispensable. They are utilized in various applications, from assembly lines to material handling. For instance, magnetic separators help remove metal contaminants from products during manufacturing, ensuring quality control and safety.
Permanent magnets also play a role in heavy machinery. Hoisting systems often rely on magnetic pulleys for lifting materials safely. They contribute to the automation of assembly lines, improving the efficiency of operations while reducing human error.
Other industrial uses include magnetic clamping systems, which are essential in machining processes. These clamps align and hold metal workpieces securely during production, thus enhancing precision.
Medical Uses for Permanent Magnets
Medical technology increasingly integrates permanent magnets, showcasing their adaptability. In MRI machines, permanent magnets create a strong magnetic field necessary for imaging. Their reliability significantly influences the accuracy of medical diagnostics by providing high-resolution imaging of internal body structures.
Moreover, magnets are used in devices that operate on principles of magnetotherapy. These devices are believed to aid in pain relief and recovery, though more research is needed to validate their efficacy.
Permanent magnets also find use in implantable medical devices. Their compact size and durability are essential for components like pacemakers, which need a reliable energy source to function effectively over time.
"Permanent magnets are at the frontier of various technological advancements, demonstrating their essential role in modern applications."
Future Prospects for Permanent Magnet Research
The field of permanent magnet research has numerous implications for future technologies and applications. As industries strive for more efficient and sustainable solutions, innovations in magnet technology are at the forefront of this quest. Researchers are focusing on enhancing the performance, reducing costs, and addressing environmental concerns associated with the production of permanent magnets. This is crucial, especially as global demand for powerful and compact magnets increases. The significance of this topic lies in its potential to drive advancements across various sectors, including renewable energy, electric vehicles, and consumer electronics.
Innovations on the Horizon
Several exciting innovations are emerging in the field of permanent magnet research. One notable development is the exploration of new materials to create magnets that are not only high-performing but also more sustainable. Researchers are experimenting with alternatives to rare earth materials. For instance, advancements in metallic alloys have shown promise, offering similar benefits without relying heavily on scarce resources.
Further, techniques like 3D printing are beginning to reshape how magnets can be produced. This manufacturing approach can reduce waste and allow for more complex magnet geometries, potentially enhancing their performance. Using design optimization algorithms, researchers can create custom magnets tailored for specific applications, improving efficiency.
Additionally, increasing focus on recycling methods for rare earth elements may significantly impact future developments. By establishing methods to recover and reuse these materials, researchers might mitigate supply chain issues and environmental impact, making the production of permanent magnets more eco-friendly.
Potential Challenges in Magnet Research
While the future of permanent magnet research looks promising, various challenges persist. One major concern is the dependency on limited earth resources, particularly rare earth metals like neodymium and dysprosium. Fluctuations in their availability can lead to price volatility, which affects the entire supply chain. Finding viable substitutes or improving the recycling processes becomes essential to address these concerns.
Another challenge involves the balance between performance and sustainability. Innovations that aim to reduce environmental impact should not compromise the magnetic performance required in various applications. This balance will require careful consideration and further research.
Lastly, there is ongoing competition within the industry to develop next-generation magnets that can outperform existing models. The race for innovation can lead to increased research and development costs. Therefore, collaboration among stakeholders—researchers, manufacturers, and policymakers—will be vital in overcoming these challenges while pushing the boundaries of permanent magnet technology forward.
"The future of permanent magnet technology depends not only on innovation but also on sustainable practices and collaborative efforts across industries."
Finale
The conclusion serves a vital role in summarizing the various elements discussed throughout this article regarding permanent magnets. It encapsulates the significance of understanding the diverse types of permanent magnets and their implications in various fields. By closing the loop on the core topics covered, the conclusion helps readers to integrate the knowledge they have gained into a coherent overall understanding.
Recap of Key Points
In this article, we have explored a range of permanent magnets, each with unique properties and applications.
The key points include:
- Types of Permanent Magnets: We discussed ferromagnetic, ferrimagnetic, and rare earth magnets.
- Material Properties: Characteristics such as magnetic strength, temperature stability, and manufacturing processes were highlighted.
- Applications: The usage of permanent magnets in consumer electronics, industrial sectors, and healthcare was extensively examined.
- Future Trends: Advancements in material science and sustainability ongoing challenges in research were outlined.
These points illustrate the complexity and importance of magnets in modern technology and science.
Final Thoughts on Permanent Magnets
Permanent magnets are not only fundamental in a myriad of applications but also pivotal in driving technological innovations. Their diverse applications, ranging from everyday items like speakers and motors to specialized uses in medical machinery, underline their relevance across numerous sectors. Recognizing the potential of permanent magnets can lead to new applications and improved efficiencies in existing technologies.