Understanding Gel Electrophoresis: Mechanisms and Applications
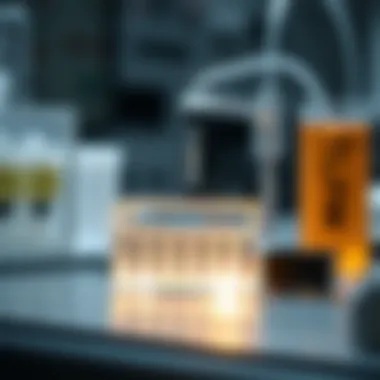
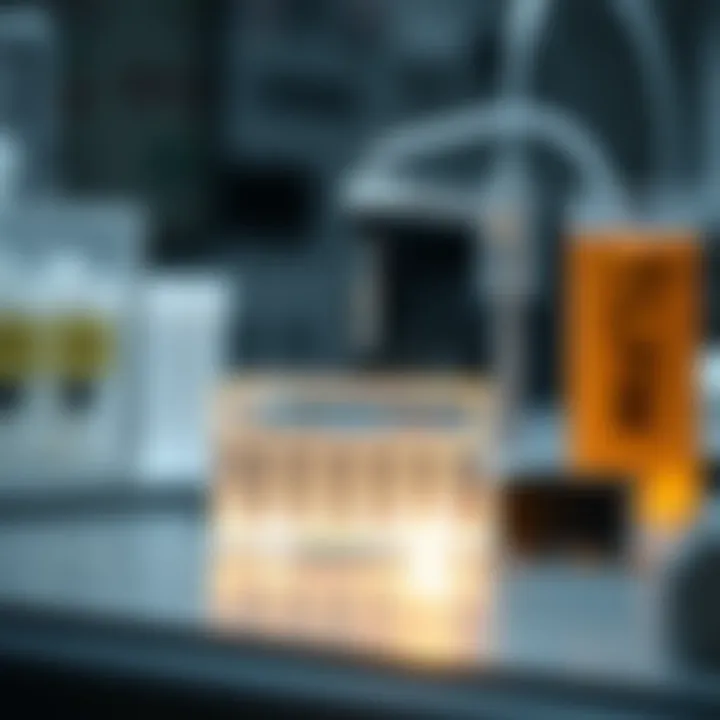
Intro
Gel electrophoresis stands as a cornerstone technique in molecular biology, enabling scientists to distinguish among various biomolecules with remarkable precision. This method not only facilitates the analysis of DNA, RNA, and proteins, but it also paves the way for groundbreaking innovations in genetic research, forensic science, and medical diagnostics. The ability to manipulate and visualize these biomolecules has reshaped our understanding and approaches to genetic analysis, offering both students and seasoned professionals an invaluable tool in their arsenal.
As we delve deeper into the mechanisms behind gel electrophoresis, it becomes essential to grasp not just the technical steps involved, but also the historical context and technological advancements that have swept through the field. By connecting these dots, we can appreciate how gel electrophoresis has evolved into a sophisticated method that continues to expand its applications, driven by the relentless pursuit of knowledge in biochemistry and molecular biology.
Intro to Gel Electrophoresis
The world of molecular biology and biochemistry is replete with techniques that allow scientists to analyze, separate, and manipulate biomolecules. Among these, gel electrophoresis stands as a cornerstone method that has revolutionized the way we understand and work with genetic material and proteins. Its significance transcends mere utility; it embodies a technological advancement that has paved the way for breakthroughs in genetic research, diagnostics, and forensic science.
Understanding gel electrophoresis entails more than just grasping how it works; it's about appreciating its relevance in both academic and applied disciplines. Gel electrophoresis enables researchers to visualize the unique fingerprints of DNA and the intricate profiles of proteins, thus opening the door to genetic mapping, disease diagnosis, and even evolutionary studies. The versatility of this technique is noteworthy, as it can be employed in various forms and settings—from academic labs to clinical environments.
With this article, we aim to delve deeper into the ninny aspects of gel electrophoresis, elucidating not just the 'how' but also the 'why' and 'where' it fits within the larger framework of molecular research. The benefits of mastering this technique are abundantly clear: knowledge of gel electrophoresis is a crucial part of the toolkit for students, researchers, educators, and professionals. Understanding the appropriate contexts for its application can significantly enhance experimental outcomes and research findings.
Additionally, as one ventures through each section of this article, it will become apparent that this technique is continuously evolving. From basic interpretations to complex analytical applications, gel electrophoresis stands at the intersection of innovation and tradition in scientific exploration.
"Understanding electrophoresis is like grasping the pulse of molecular biology—anyone looking to contribute to this field must appreciate its dynamics and applications."
Next, we will navigate towards defining and providing a comprehensive overview of gel electrophoresis, laying the groundwork for a richer understanding of its mechanics and application in the research landscape.
Principles of Electrophoresis
The principles of electrophoresis form the bedrock of understanding how gel electrophoresis works, laying the groundwork for successful separations of biomolecules. A grasp of the fundamental concepts like charge, movement of molecules in an electric field, and the behavior of different gel matrices is crucial for researchers and students alike. These principles not only illuminate how molecules migrate but also assist in troubleshooting various issues during experimental procedures. Moreover, understanding the parameters that govern electrophoresis empowers users to optimize their methodologies for better results.
Basic Concepts of Charge and Movement
At the heart of electrophoresis lies the relationship between charge and movement. When an electric field is applied across a gel matrix, charged molecules experience forces pulling them towards the electrode of opposite charge. It's like a game of tug-of-war where the outcome depends upon the charge of the player. Positively charged molecules—known as cations— migrate towards the negatively charged electrode (cathode), while negatively charged molecules, or anions, move toward the positively charged electrode (anode). This basic, yet fundamental, concept dictates their movement speed, allowing for separation based on size and charge. For instance, smaller molecules often traverse the gel more quickly than larger ones, a key point to remember when planning experiments.
Gel Matrix Properties
The properties of the gel matrix significantly affect the outcome of electrophoresis. Choosing the right type of gel, and its concentration, can be pivotal in achieving accurate separations. Let’s delve deeper into these aspects.
Types of Gels
There are primarily two types of gels used in electrophoresis: agarose and polyacrylamide.
- Agarose Gel: This gel is derived from seaweed and is popular for separating larger DNA fragments. It’s easy to prepare and provides a favorable environment for biomolecules to migrate. One striking feature of agarose is its ability to form a porous matrix that allows for efficient separation of nucleic acids. However, agarose gels are not the best choice for resolving smaller proteins due to their larger pore size.
- Polyacrylamide Gel: In contrast, polyacrylamide offers a much tighter matrix, which is beneficial for separating smaller proteins. Its adjustable pore size and excellent resolution capabilities make it a favorite for many protein studies. However, preparing polyacrylamide gels can be more complex compared to agarose.
The gel type choice shapes the outcome of molecular separation, making it a crucial step in gel electrophoresis.
Selecting the Appropriate Gel Concentration
The concentration of the gel you choose has a direct effect on the resolution of your samples. Higher concentrations lead to smaller pores, making them suitable for smaller molecules. Conversely, lower concentrations are better for larger biomolecules.
- Key Characteristic: The unique feature of gel concentration is that it can be tailored to suit specific experimental needs. For example, using a 1% agarose gel might suffice for larger DNA fragments, whereas a 10% polyacrylamide gel may be required for separating small proteins effectively.
- Advantages and Disadvantages: Selecting the right concentration is not just about resolution; it's also about optimizing the resolution for the specific molecule's size. If the concentration is too high, it could impede the mobility of larger molecules. On the flip side, if it’s too low, smaller molecules might pass through too easily, resulting in poor separation.
Understanding these components deepens comprehension of gel electrophoresis and empowers practitioners to make informed decisions, ultimately driving better experimental results.
Components of Gel Electrophoresis Systems
Understanding the components that comprise gel electrophoresis systems is crucial for anyone looking to delve into this method. The efficiency and reliability of this technique heavily depend on the apparatus and power supply. Failure in either of these can lead to unreliable results or even damage to samples. Let's break it down into these two major categories.
Gel Apparatus
The gel apparatus is at the heart of gel electrophoresis. This is the setup where the gel is cast and samples loaded for separation. Typically, a gel box consists of several components: the tray to hold the gel, the comb to create wells for sample loading, and the electrodes responsible for applying the electric field.
When considering the gel apparatus, a few key features matter:
- Stability: It must be sturdy enough to prevent any movement during the run, as even slight shifts can cause irregularities in bands.
- Compatibility: Ensure that the apparatus is compatible with the type of gel being used, whether it be agarose or polyacrylamide. Different gels may have different requirements concerning the dimensions and construction of the apparatus.
- Ease of Use: Designs that facilitate easy loading and visualization can save precious time and reduce user errors.
When setting up, proper assembly is vital. Inadequate sealing can lead to plans gone awry, with buffer leakage resulting in band distortion. An ill-prepared apparatus is like a leaky boat; it will sink the project if not addressed before setting sail.
Power Supply
A reliable power supply is the lifeline of any electrophoresis run. It provides the electric current necessary to drive the movement of charged molecules through the gel matrix. Without it, gel electrophoresis would just be an exercise in futility.
Here are important considerations when choosing a power supply:
- Voltage Control: Some methods require precise voltage settings. A power supply that allows for easy adjustments will help optimize the conditions for the samples being analyzed.
- Current Limiting: This feature helps prevent overheating and ensures the gel can withstand longer runs without degradation.
- Voltage and Time Monitoring: Understanding how to monitor these parameters helps prevent run conditions from veering into dangerous territory.
It's imperative that researchers do not overlook their power supply. A malfunction can lead to uneven bands, smearing results or in the worst-case scenario, loss of precious samples. Just as you wouldn't drive a car with a faulty engine, running a gel with a dubious power source is a gamble that isn't worth taking.
"The quality of the equipment directly affects the quality of the results; investing in good equipment is paramount for reproducible outcomes," says Dr. Emily Chen, a leading researcher in molecular biology.
In sum, the components of gel electrophoresis systems serve as the backbone of the process. A sturdy and well-designed gel apparatus combined with a reliable power supply can make or break experiments in biomolecule separation. As you embark on this scientific journey, remember: each component plays a vital role in shaping the outcomes of your experiments.
Sample Preparation Techniques
Sample preparation techniques stand as the backbone of gel electrophoresis, akin to a solid foundation for a house. Without proper preparation, the reliability and accuracy of experimental results can suffer significantly. It's critical to handle samples with care and precision, ensuring that the identity and concentration of biomolecules are well preserved for optimal separation during the electrophoresis process.
Executing appropriate sample preparation can make a world of difference. It helps in removing contaminants that may interfere with the electrophoretic run, allowing for cleaner and clearer results. Additionally, well-prepared samples can lead to sharper bands, better resolution, and improved reproducibility of experiments.
Key considerations in sample preparation include maintaining consistency and avoiding common pitfalls, like degradation or contamination. Both DNA and protein samples demand specific techniques to optimize their integrity and concentration before analysis.
Preparing DNA Samples
When it comes to preparing DNA samples, the process is straightforward yet pivotal. First off, one needs to ensure that the DNA is extracted using reliable and clean protocols to avoid carryover of contaminants from cellular debris or other components. Often, researchers use methods such as phenol-chloroform extraction or silica-based capture techniques.
Once isolated, the purity and concentration of the DNA need to be quantified, commonly using spectrophotometry. A260/A280 ratio analysis provides insight into the DNA’s purity; a ratio of around 1.8 is considered pure for DNA. The samples might then be diluted to an optimal concentration for loading onto the gel, ensuring that each well receives an equal and appropriate volume
Protein Sample Preparation
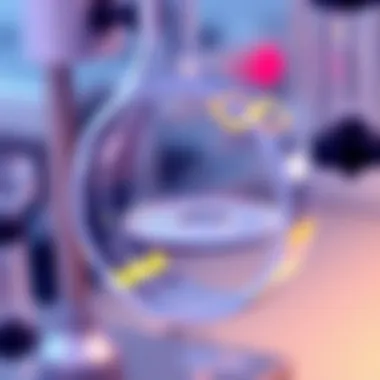
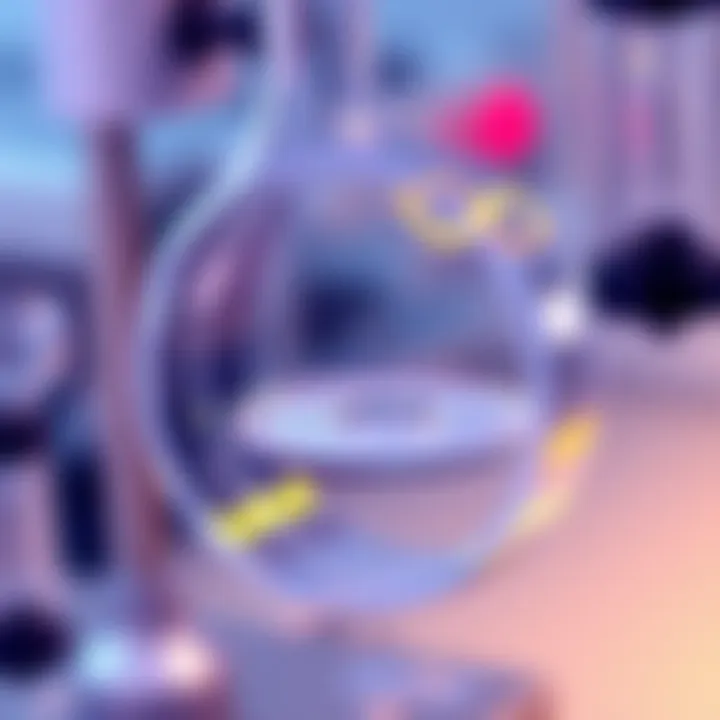
Dilution Techniques
Dilution techniques are crucial when it comes to protein sample preparation. Protein samples often need to be diluted to avoid overloading the gel, which can lead to smeared or poorly resolved bands. That's why scientists often perform serial dilutions to reach ideal concentrations.
One key characteristic of dilution techniques is their simplicity. By preparing a standard curve of known concentrations, researchers can extrapolate concentrations in their unknown samples with accuracy. It’s a beneficial choice because it lays down a straightforward approach for a variety of protein samples, from pure extracts to more complex mixtures.
The main advantage of proper dilution is that it helps achieve greater precision in results and reproducibility across experiments. However, it’s important to remember that excessive dilution can lead to loss of valuable protein, shutting down the ability to analyze low-abundance proteins in a sample.
Denaturation Steps
Denaturation steps are important in protein sample preparation. This process involves unfolding the protein structure, exposing the polypeptide chains. A common practice includes the use of heat or chemical agents, such as sodium dodecyl sulfate (SDS). This step ensures all proteins are separated properly during electrophoresis, as forced into linearized forms.
One key aspect of denaturation is the increased visibility of protein bands, which provides more accurate interpretations. It’s a popular method because it streamlines the step before electrophoresis, allowing for a more facilitated analysis.
However, denaturation comes with its own set of challenges. Overdenaturating the samples can lead to protein aggregation or degradation. Finding the right balance is essential to prevent any loss of information about the protein's properties.
"Proper sample preparation is like seasoning in cooking; a little goes a long way, but too much can ruin the dish."
Through a meticulous approach in preparing both DNA and protein samples, researchers can push the boundaries of gel electrophoresis applications, unveiling a world where biomolecule separation is not only possible but optimized.
Running Gel Electrophoresis
Running gel electrophoresis is a pivotal step in the larger process of molecular analysis. Without it, all the preparatory work done prior would be for naught. In this stage, the samples are driven through a gel matrix under the influence of an electric field, allowing for the separation of biomolecules such as DNA, RNA, or proteins based on their size and charge. This process is critical not only for resolving complex mixtures but also for obtaining insight into the composition and structure of the biomolecular entities in question. Here, we will delve into the specifics of setting up the gel and managing the operational conditions to maximize the effectiveness of the electrophoresis process.
Setting Up the Gel
The setup of the gel is where the magic truly begins. This involves preparing the gel matrix, typically made from agarose or polyacrylamide, depending on the type of molecules you are analyzing. The right concentration of the gel is crucial; too low, and larger molecules may traverse too quickly, while too high can hinder even smaller ones from migrating appropriately. Thus, achieving the right balance can be a bit of a Goldilocks situation - not too thick, not too thin, just right.
- Gel Preparation: Start by dissolving the agarose powder in a buffer solution, then gently heat it until fully melted. After cooling slightly, pour it into a casting tray with a comb positioned to create wells. Be mindful not to let it cool too much before pouring, as this can lead to premature solidification.
- Well Formation: Make sure the comb is positioned correctly to yield wells of suitable size for your samples. This is where the sample loading will begin, playing a significant role in ensuring accurate positioning during the run.
- Buffer Addition: Once the gel has set, fill the electrophoresis tank with the same buffer to help conduct electricity and provide the necessary ionic environment for the movement of the charged molecules.
Operating Conditions
Once everything is set up, the focus shifts to operating conditions, which significantly impact how well gel electrophoresis will perform.
Voltage Regulation
Voltage regulation is a key characteristic of running gel electrophoresis. The applied voltage determines the rate of migration of the molecules through the gel. Too high, and you risk overheating the gel and creating distortions in the separation. Conversely, too low can make processes take far longer than necessary, which isn’t practical in either research or clinical settings.
- Optimal Settings: Usually, a voltage of around 5 to 10 volts per centimeter of gel length is recommended, depending on the type of gel and the size of the nucleic acids or proteins being analyzed.
- Cooling Mechanisms: Employing coolers or fans can help maintain the gel’s integrity by preventing excessive heat generation.
The ability to finely tune voltage settings can also assist in the resolution of closely migrating bands, enhancing the clarity and quality of results.
Time Management
Time management during the running phase is equally critical. The duration of electrophoresis can affect the separation's quality and resolution.
- Migration Time: Monitoring how long the gel runs is essential. Typically, one should stop the run when the dye front reaches a specific distance, usually about two-thirds down the gel. This timing ensures you do not lose your samples out of the bottom of the gel.
- Run a Pilot Experiment: It’s often wise to perform a trial run under similar conditions to understand how long to run your experiment without losing sight of crucial data points.
Keeping an eye on the clock can be challenging in busy labs, yet it's imperative for achieving reproducible and accurate results that can be analyzed later.
"Attention to detail during the running phase of gel electrophoresis can mean the difference between a clear, interpretable result and a frustratingly ambiguous one."
Effectively managing both the voltage and time ensures a streamlined running process, allowing for higher-quality data accumulation designed to support subsequent analysis and interpretations. Understanding these nuances serves to refine methods and ultimately drive more significant scientific discoveries.
Visualization Techniques
Visualization techniques are pivotal in gel electrophoresis, allowing researchers to observe, interpret, and analyze the separation of biomolecules post-electrophoresis. Presently, there are several methods used to stain and visualize DNA and proteins in gels, with each technique having its unique attributes that cater to different experimental requirements. Choosing the right visualization method can significantly impact the quality and clarity of results.
Staining Methods
Ethidium Bromide
Ethidium bromide, or EtBr, has been a staple in molecular biology labs for decades, primarily known for its effectiveness in visualizing DNA. This intercalating agent binds to the DNA double helix, fluorescing under ultraviolet light. What sets EtBr apart is its simplicity and reliability. It's especially beneficial when analyzing nucleic acid bands, as the fluorescent intensity correlates with the amount of nucleic acid present, enabling quantification and assessment of DNA integrity.
However, there are some considerations regarding safety and toxicity. Ethidium bromide is a known mutagen, meaning there’s a risk of genetic damage, which elevates handling precautions during experiments. Although ultraviolet light allows direct visualization, it can also lead to DNA degradation if prolonged exposure occurs. In summary, EtBr is a popular choice for its sensitivity and cost-effectiveness, yet users must weight the safety risks it entails.
Silver Staining
Silver staining is another technique widely regarded for its high sensitivity in protein visualization. This method can detect proteins in low concentrations, often as minute as 0.1 ng. What makes silver staining distinctive is its ability to produce sharp, well-defined bands, which are invaluable during protein analysis. Unlike ethidium bromide, silver staining does not rely on fluorescence and provides a robust contrast against gel backgrounds, making it easier to differentiate bands.
However, preparing silver-stained gels can require a bit more time and resources due to the necessary steps like fixation and developing processes. Moreover, it is generally less straightforward than EtBr and might result in some background noise if conditions are not optimized. Silver staining might also not be ideal for all electrophoretic settings, particularly those used for nucleic acids. Overall, the advantages of silver staining include its heightened sensitivity and clear band presentations, making it a top choice for specific protein studies.
Image Analysis
The process of interpreting results doesn’t stop at visualizing bands; it extends further to image analysis. Software tools, designed specifically for analyzing gel images, come into play by enabling quantification of band intensity and size. Impartial analysis is crucial, as it can provide insights into concentration levels, molecular weight estimations, and potential abnormalities in samples. Having accurate data can make a significant difference, particularly in diagnostic applications or experimental validations. Ultimately, a thorough understanding of image analysis augments the utility of gel electrophoresis techniques.
Interpretation of Results
The interpretation of results in gel electrophoresis holds significant importance as it resonates through the various stages of analysis, allowing researchers to draw meaningful conclusions from their experiments. This process goes beyond mere observation; it combines analysis with an understanding of the underlying principles of molecular biology. Proper interpretation empowers scientists to assess the quality of their samples, validate their hypotheses, and expand their knowledge in fields ranging from genetics to biochemical research.
Essentially, interpreting results involves reading the gel patterns generated during electrophoresis. Scientists utilize these patterns to gain insights about the size, charge, and quantity of the separated biomolecules. The finely-tuned methodology employed in this technique hinges on the precision of these interpretations. A misinterpretation could lead to erroneous conclusions and ultimately affect the reliability of the research.
As researchers dive into analyzing gel results, there are specific elements to keep in mind:
- Clarity of Bands: Well-defined and distinct bands indicate successful separation of biomolecules. If bands appear smeared or diffuse, it might suggest issues during the running process or sample preparation.
- Migration Distance: The distance traveled by the bands can provide critical information about the size of the biomolecules. Smaller molecules will migrate farther compared to their larger counterparts, which will have a limited migration range.
- Lane Consistency: Comparing bands across different lanes can help assess sample integrity and concentration. Consistent patterns lend credibility to the experimental process.
In the following sections, we will explore how to read gel patterns and estimate molecular weights. Understanding these concepts not only serves to enhance results interpretation but also contributes to better study design and execution overall.
Reading Gel Patterns
Reading gel patterns is akin to deciphering a coded message. Each band represents a distinct biomolecule, and the arrangement as a whole tells a story about the samples at hand. A typical gel may exhibit multiple lanes, each designated for a distinct sample. Here’s how to effectively analyze these patterns:
- Identify Band Characteristics: Note the number of bands, their sizes, and intensities. Each of these factors can tell you whether the sample is pure, contains degradation products, or represents multiple species.
- Compare with Standards: Utilizing a molecular weight marker is a widely accepted method to gauge the sizes of your unknown samples. These benchmarks assist researchers in determining via comparison whether they are observing expected or unexpected molecules.
- Evaluate Background Signals: Look out for any background staining. An excessively dark background may obscure band visibility and complicate interpretation. The goal is clear, manageable results that speak loudly about molecular presence.
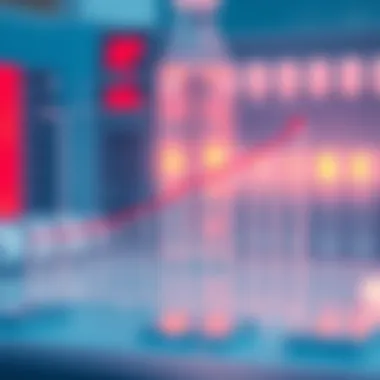
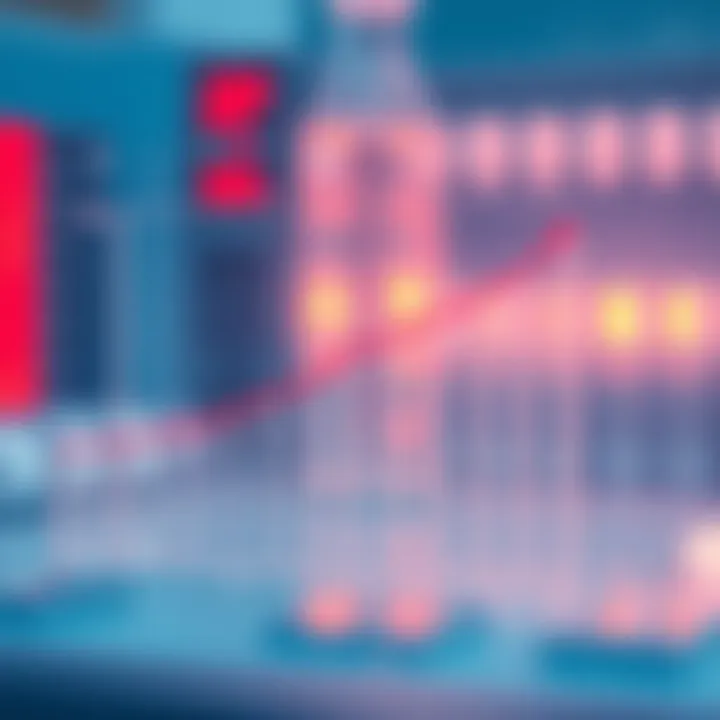
"Reading gel patterns correctly not only affirms the validity of your experimental results but also shapes the direction of future inquiries."
Molecular Weight Estimation
Molecular weight estimation is a critical step following the reading of gel patterns. It translates the visual data into quantitative information essential for understanding the biomolecules being studied. Accurate estimates can assist in the following ways:
- Characterization of Molecules: Knowing the molecular weight aids in classification—determining if a protein or DNA fragment behaves in a manner consistent with its known properties.
- Verification of Purity: If the estimated molecular weight matches the expected value for your target molecule, it's a strong indicator of sample purity.
- Facilitating Comparisons: When assessing samples across different experiments or conditions, having molecular weight estimates allows researchers to compare and contrast forces at play.
To estimate molecular weight from gel results, researchers typically rely on:
- Standard Curves: Constructed using known sizes of standard proteins or DNA fragments to help extrapolate unknowns based on migration distances.
- Mathematical Calculations: Utilizing Simple formulas to relate distance traveled by unknown bands with their respective weights. This step is crucial, although retention of a degree of caution is required—molecular weight is not always linear with migration distance.
Applications of Gel Electrophoresis
Gel electrophoresis has found its niche in various fields of molecular biology and biochemistry. The ability to separate and analyze DNA, RNA, and proteins offers significant insights for both research and clinical applications. Through this powerful technique, scientists can decipher the complexities of genetic and proteomic data, which is crucial for advancing our understanding of biology at a molecular level. Let's dive into several prominent applications of gel electrophoresis, highlighting their equal importance.
DNA Analysis and Fragmentation
The application of gel electrophoresis in DNA analysis cannot be overstated. It serves as a fundamental tool for researchers aiming to navigate the intricacies of genetic material. By using this technique, scientists can separate DNA fragments based on length and size—which is instrumental in various genetic studies.
For instance, agarose gel electrophoresis is commonly employed when examining PCR products to confirm amplification or to analyze restriction fragment length polymorphisms (RFLPs). The visualization of these fragments, often stained with ethidium bromide, enables scientists to determine the success of their experiments quickly. Moreover, this method is pivotal for applications such as cloning, sequencing, and even genetic mapping.
Amplifying the understanding of genetic material through gel electrophoresis allows researchers to establish connections between DNA sequences and their corresponding phenotypes, driving forward the field of genomics.
Protein Characterization
Just as DNA analysis is a hallmark of genetic research, gel electrophoresis plays a significant role in protein characterization. It allows for the separation of protein mixtures, which is essential for identifying, quantifying, and analyzing proteins. Techniques such as SDS-PAGE utilize a polyacrylamide gel matrix to denature proteins and provide a clear separation based on molecular weight.
The insights gained from protein electrophoresis are critical for numerous applications, including:
- Identifying protein isoforms and post-translational modifications. Electrophoresis helps researchers reveal different forms of proteins that may be crucial for understanding diseases.
- Analyzing enzyme activity and interaction. Gel electrophoresis can be used to study how proteins interact with substrates or other molecules, which is critical in drug discovery and development.
- Characterizing complexes. When proteins form complexes, gel electrophoresis can help unravel their constitution and properties, assisting in elucidating biological functions.
Genetic Fingerprinting
Genetic fingerprinting, or DNA profiling, is a powerful application of gel electrophoresis. This technique enables the identification of individuals based on unique patterns in their DNA, making it invaluable in forensic science, paternity testing, and biodiversity studies.
Through the utilization of microsatellite markers or short tandem repeats (STRs), researchers can differentiate between individuals by observing the variation in DNA sequences. The gel provides a visual format where the amplified STR fragments can be compared across different samples, showcasing unique banding patterns that signify genetic differences.
In recent years, advancements have been made in simplifying and automating these processes, furthering the relevance of this technique in large-scale studies.
Advantages and Limitations
Gel electrophoresis is a cornerstone technique in molecular biology, known for its effectiveness and versatility in separating nucleic acids and proteins. However, this method is not without its quirks and drawbacks. Knowing the strengths and limitations of gel electrophoresis is essential, particularly when planning experiments or interpreting results. Understanding these aspects enhances decision-making processes, making it easier to choose the right technique for specific applications.
Strengths of Gel Electrophoresis
One of the standout strengths of gel electrophoresis lies in its ability to separate biomolecules based on size and charge. This separation is not just efficient; it is crucial for various scientific inquiries, allowing researchers to analyze DNA fragments, RNA, and proteins with precision. The range of gel types available, from agarose to polyacrylamide, provides flexibility to cater to different applications. For example, agarose gels are ideal for larger DNA fragments, while polyacrylamide excels in separating smaller proteins due to its enhanced resolution capabilities.
Beyond mere separation, gel electrophoresis offers quantitative insights. The intensity of the bands can be correlated to the amount of biomolecule present, making it a valuable technique for quantifying nucleic acids or proteins in a sample. Further, the compatibility of gel electrophoresis with various visualization methods, such as staining and fluorescence, enriches the data derived from experiments, leading to a more comprehensive understanding of the sample composition.
Challenges and Potential Errors
While gel electrophoresis is a powerful tool, it comes with its share of challenges that can lead to inaccurate results or poor reproducibility. Understanding these challenges is key for researchers in the field to mitigate issues and optimize outcomes.
Contamination Issues
Contamination stands as a pervasive concern in any laboratory process, and gel electrophoresis is no exception. The presence of foreign substances, such as other DNA or proteins in the sample, can skew results significantly. This issue highlights one of the major drawbacks of gel electrophoresis—its reliance on purity for accuracy. Ensuring that samples are free from contaminants is crucial to obtaining reliable data. Moreover, the gels themselves might absorb contaminants from the environment or reagents, introducing errors into the experiment.
Key characteristics of contamination issues include their potential to obscure results, leading to false positives or negatives, which can severely compromise the conclusions drawn from an experiment. Thus, maintaining an aseptic environment and using high-quality reagents are beneficial practices to minimize contamination risk.
Running Artefacts
Running artefacts are another tricky issue faced in gel electrophoresis. These abnormalities can arise during the running phase, often due to bubbles, uneven gel quality, or faulty electrical connections. They can create misleading bands on the gel that resemble the target biomolecules, complicating the analysis significantly.
The uniqueness of running artefacts lies in their diverse manifestations, making them difficult to identify at times. They can be mistaken for genuine results, which can lead to erroneous conclusions being drawn from experiments. To overcome this hurdle, careful monitoring during the run and proper setup of electrophoresis parameters are critical. Paying attention to gel preparation and ensuring consistent running conditions can help minimize the occurrence of these artefacts.
"Effective use of gel electrophoresis not only requires an understanding of its strengths but also a keen awareness of the potential pitfalls that can undermine the results obtained."
Navigating the advantages and limitations of gel electrophoresis allows researchers to appreciate its contributions while remaining vigilant of the challenges it presents. Establishing best practices for sample preparation and experimental execution becomes vital in harnessing the full potential of this significant technique.
Recent Advances in Gel Electrophoresis Techniques
As science progresses at a breakneck pace, it's imperative that methods too evolve to keep up with the demands of modern research. The advances in gel electrophoresis reflect both ingenuity and necessity, making this technique more user-friendly and efficient than ever before. Not just another step forward, these advancements are paving the way for more precise, quicker, and cost-effective applications that benefit a variety of fields, from genetics to proteomics. Understanding these innovations gives us a clearer picture of the future of molecular analysis.
Automation and High-Throughput Methods
The push for automation in gel electrophoresis signifies a shift from traditional, labor-intensive approaches to systems that can process many samples at once with high efficiency. High-throughput methods allow researchers to analyze hundreds, if not thousands, of samples concurrently—something that simply wasn't feasible before.
Benefits include:
- Increased Efficiency: Researchers spend less time setting up and running gels, leading to higher productivity.
- Consistent Results: Automated systems minimize human error, ensuring reproducibility across experiments.
- Cost-Effectiveness: Although initial investment in automated systems can be high, the reduction in labor and time ultimately leads to savings.
However, this does come with some considerations. There are upfront costs associated with automation that can be a barrier for some labs. Furthermore, the need for skilled technicians to operate these sophisticated machines cannot be understated; it's not all push-button simplicity. There’s also the learning curve of getting used to these systems, which may require additional training for the staff. Thus, while automation presents significant advantages, careful planning is needed to make it a viable choice.
Innovative Gel Materials
The development of innovative gel materials is another exciting frontier in gel electrophoresis. Traditional agarose and polyacrylamide gels still rule the roost, but researchers are now exploring new matrices that can enhance resolution, reduce running time, and improve the separation of complex mixtures.
For example, gels incorporating specialized polymers can offer:
- Higher Resolution: More refined separation of biomolecules, allowing for better discrimination between closely related species.
- Faster Run Times: Some new materials require lower voltages and still yield high-quality results, enabling quicker experiments.
- Eco-friendliness: Eco-conscious materials can lessen environmental impacts associated with gel disposal and preparation.
In addition to being more effective, these advancements come with challenges. The compatibility of innovative gels with existing equipment might require upgrades, and the analytical techniques to evaluate results could need refinement. Every leap forward brings its own set of hurdles, and as labs grapple with adopting these new materials, they must balance the urge to innovate with practical considerations.
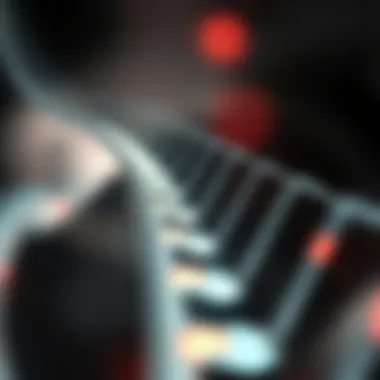
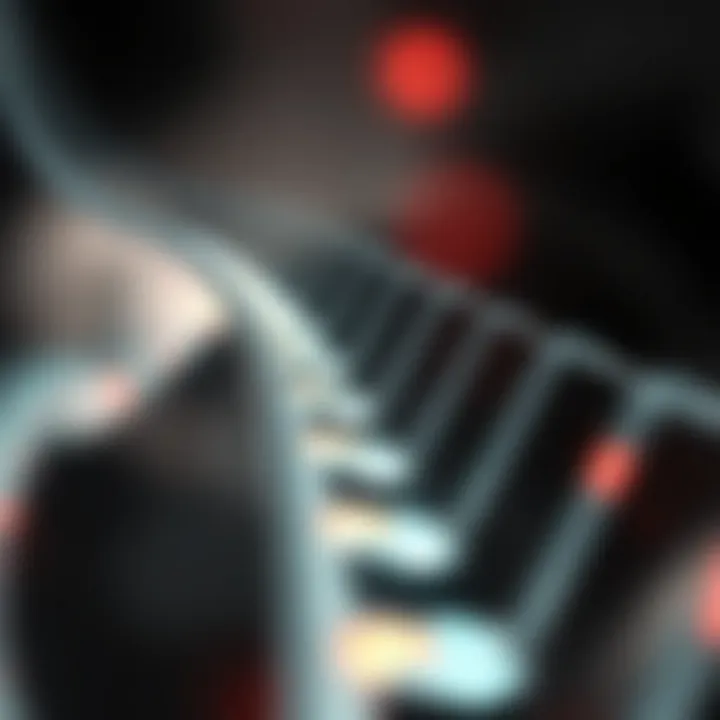
"Innovation in gel materials is not merely a technical enhancement; it’s a foundational shift that may redefine experimental methodologies in molecular biology."
As researchers continue to push the boundaries, they will find that recent advances in gel electrophoresis open exciting avenues for investigation, transforming how we approach molecular analysis.
Comparative Techniques
In the realm of biochemical analysis, one must often evaluate the effectiveness of various methodologies for the separation of biomolecules. Comparative techniques provide essential insights into the strengths, weaknesses, and overall applicability of these methods. Understanding these alternatives to gel electrophoresis enables researchers to choose the most suitable approach to achieve their specific objectives.
Capillary Electrophoresis
Capillary electrophoresis (CE) has emerged as a significant alternative to traditional gel electrophoresis. The technique operates on similar principles, involving an electric field to separate analytes based on charge and size; however, its execution differs profoundly. In CE, capillaries devoid of gel are used, which reduces the distance molecules travel, thereby enhancing resolution and speeding up the process. The high surface area-to-volume ratio in capillaries allows for efficient heat dissipation, minimizing the risk of sample degradation.
The benefits of capillary electrophoresis include:
- High sensitivity: CE can detect low concentrations of biomolecules due to the reduced sample volume.
- Rapid analysis: The electric field in a narrow capillary leads to faster separations compared to gels.
- Less sample preparation: The absence of gel casting simplifies the workflow.
One must also consider the limitations of CE. For instance, the initial setup can be expensive, and skilled labor is often required to operate and maintain the apparatus. Furthermore, while it's excellent for certain samples, it may not provide the same level of separation for complex mixtures compared to gel methods.
Polymerase Chain Reaction (PCR)
Although Polymerase Chain Reaction (PCR) is not a direct alternative for separation like capillary electrophoresis, it plays a complementary role in biomolecule analysis. This technique amplifies specific DNA segments, making it crucial when quantities are limited. The amplified DNA can subsequently be analyzed using gel electrophoresis, bridging the gap between these two methodologies.
Key aspects of PCR include:
- Specificity: By using specific primers, PCR targets only desired sequences for amplification.
- Sensitivity: Even a small amount of DNA can produce significant results, making it valuable in forensic and diagnostic applications.
- Speed: PCR can generate millions of copies within hours, facilitating downstream applications.
Yet, PCR is not without its caveats. Issues of contamination can yield erroneous results, and the amplification reaction must be carefully monitored to prevent biases in DNA representation. It requires a solid understanding of the process to optimize conditions and ensure high fidelity.
"PCR is a real game-changer in molecular biology, but ensuring the integrity of samples can't be stressed enough. Contamination can lead you on the wrong path."
When you juxtapose gel electrophoresis, capillary electrophoresis, and PCR, it becomes evident that each offers unique strengths. This understanding is vital for researchers striving for accuracy and efficiency in the separation and analysis of biomolecules. Thus, recognizing these comparative techniques is crucial to advancing methodologies in molecular biology.
Future Directions in Gel Electrophoresis Research
As the field of molecular biology continues to evolve, so too does the need for refined methods in gel electrophoresis. This technique has been a mainstay in laboratories for years; however, researchers are ever so keen on advancing its capabilities. Future directions for gel electrophoresis research include improved resolution techniques and integration with other technologies. These advancements promise to enhance its utility and applications significantly.
Improved Resolution Techniques
Resolution in gel electrophoresis refers to the clarity with which distinct bands can be observed. One of the foremost challenges faced by practitioners is separating biomolecules that are closely related in size or charge. Here’s where innovations are stepping in. New materials and modified gel compositions are emerging to enhance separation efficiency. For example, researchers are exploring the use of agarose gels with specialized additives, which help to create a more uniform matrix structure. This results in sharper banding patterns and minimizes effects like diffusion that can muddy results.
Moreover, advancements in electric field manipulation also play a pivotal role. Using non-linear electric fields has shown promise in separating nucleic acids and proteins more effectively than traditional linear methods. By allowing these molecules to experience variable field strengths, the separation can be sharper and more defined.
In practice, integrating these new materials and techniques may involve additional training and adjustments in existing protocols. However, the resulting clarity of band patterns would justify this investment of time and resources.
Integration with Other Technologies
The future of gel electrophoresis isn’t just about refining existing techniques; it’s also about building bridges with other technologies. For instance, coupling gel electrophoresis with mass spectrometry is gaining traction. This combination can provide detailed insights where gel electrophoresis alone may fall short. When a sample is first separated using electrophoresis and then analyzed via mass spectrometry, it provides both size and mass information, leading to enhanced identification and characterization of biomolecules.
Furthermore, the integration of digital imaging and analysis software can greatly enhance the output from gel electrophoresis. Advanced imaging techniques allow for automated data capturing, leading to faster processing without sacrificing accuracy. Such collaborations can streamline workflows and enable more comprehensive data analysis.
"Innovations in gel electrophoresis are not merely incremental; they symbolize a paradigm shift, merging traditional methods with cutting-edge technologies to address contemporary challenges in biomolecular separation."
Moreover, incorporating machine learning algorithms to analyze electrophoresis data and interpret results might soon see the light of day. These algorithms could recognize patterns that are not immediately evident to the human eye, providing additional layers of insight into the biological samples being analyzed.
The combination of improved resolution and technology integration is sure to open new doors in research and clinical applications. The significance of these advancements lies not just in their technical merit but in how they meet the growing demands of precision in the scientific community.
Ending
In wrapping up the exploration of gel electrophoresis, it’s vital to acknowledge its profound impact on the fields of molecular biology and biochemistry. This technique not only enables the effective separation of biomolecules but also cultivates a deeper understanding of their inherent properties. The nuanced mechanisms behind gel electrophoresis pave the way for numerous applications, from clinical diagnostics to cutting-edge research.
Summary of Key Points
- Separation of Molecules: Gel electrophoresis is primarily employed for the separation of DNA, RNA, and proteins based on size and charge.
- Technique Versatility: The versatility in gel types and structures, coupled with different staining methods, enhances its utility across various biological disciplines.
- Application Areas: This technique plays a pivotal role in genetic fingerprinting, DNA fragment analysis, and protein characterization, making it indispensable in both research and clinical settings.
- Recent Advancements: New methods and technologies continue to emerge, pushing the envelope of what gel electrophoresis can achieve in terms of resolution and speed.
The Significance of Gel Electrophoresis in Modern Science
Gel electrophoresis serves as a cornerstone technique in modern scientific inquiry. Its ability to generate reliable results has significant ramifications:
- Precision in Analysis: It provides high-resolution data that is key in research contexts, allowing scientists to ascertain molecular weights and purify biomaterials with precision.
- Cross-Disciplinary Applications: Beyond biology, it finds relevance in fields like forensics and environmental science, evidencing its versatility.
- Educational Importance: For students and researchers alike, mastering this technique is foundational. It cultivates critical analytical thinking and problem-solving skills necessary for advancements in science.
- Integration with Emerging Tech: The ongoing developments in gel electrophoresis hint at greater integration with technologies like CRISPR and next-generation sequencing, enhancing its value further.
"The ability to separate biomolecules efficiently and effectively forms the backbone of myriad experimental protocols, ultimately leading to significant scientific breakthroughs."
In essence, the journey through gel electrophoresis illustrates not just a method, but a sophisticated interplay of mechanics, analysis, and application that keeps advancing the frontiers of science. Embracing these concepts enriches one’s understanding and opens doors to innovative practices that are quintessential in today’s research landscape.
Importance of References
References in scientific literature carry significance that extends beyond mere acknowledgment.
- Credibility: By citing reputable sources, the article gains credibility, reassuring readers that the information presented is based on established facts and research.
- Comprehensiveness: They help create a detailed narrative that fully encompasses the mechanisms of gel electrophoresis, ensuring that all readers—novices and experts alike—have the opportunity to deepen their understanding of the subject.
- Resources for Further Reading: They encourage curiosity and exploration. For those looking to dive deeper, these references often serve as a springboard towards additional literature or emerging studies in molecular biology and biochemistry.
Specific Elements
References are chosen with care to highlight various aspects of gel electrophoresis.
- Technical Manuals: These provide in-depth explanations of methods and equipment.
- Research Studies: These highlight practical applications and current innovations in the field.
- Review Articles: They summarize critical developments and discuss the evolution of gel electrophoresis over time.
Benefits of Citing References
- Facilitates Critical Thinking: When readers engage with cited materials, they can evaluate the findings and form their own conclusions, leading to a better grasp of complex concepts.
- Promotes Academic Rigor: Citing sources reinforces the academic integrity of the work, essential in scientific communication.
"References are not only a testament to one’s scholarship but also a map that guides others towards knowledge."
Considerations Surrounding References
Being meticulous about references is crucial.
- Relevance: The cited works must directly relate to the topics discussed in the article. This ensures that every reference adds value to the reader’s journey through the text.
- Currency: Scientific fields are rapidly advancing; thus, newer studies should be prioritized to reflect the latest discoveries and techniques.
In a nutshell, the references section transcends its basic function; it is a vital cog in the mechanics of this article on gel electrophoresis. It enriches the text, making it not only a source of information but also a robust tool for continued learning in the ever-evolving world of molecular biology.