Introduction to CRISPR: Mechanisms and Implications
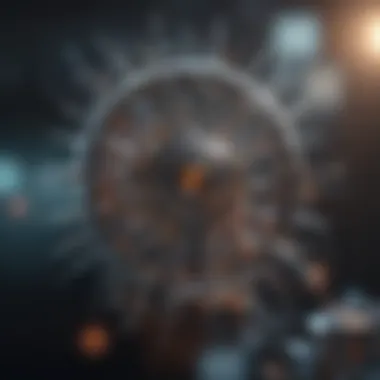
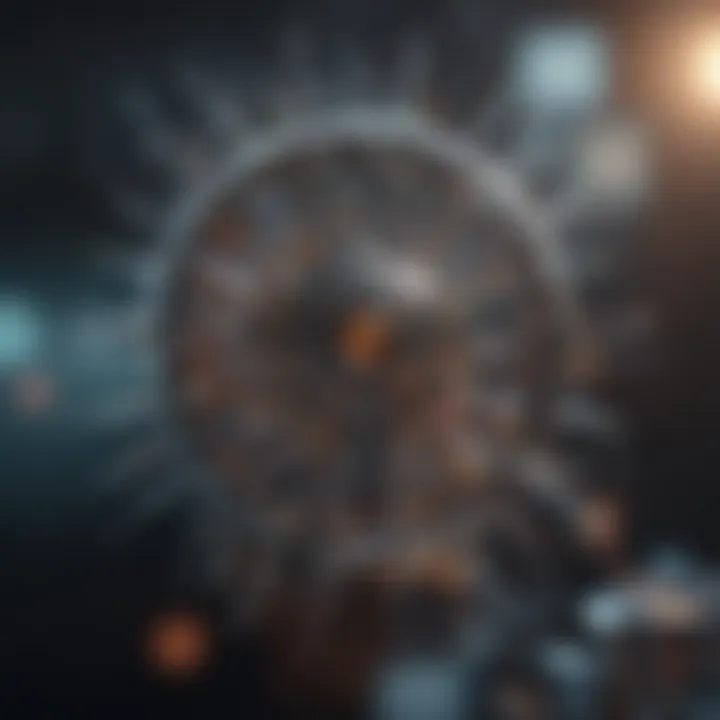
Intro
CRISPR technology stands as a revolutionary tool in the genetic engineering landscape. Its ability to edit genes with precision has garnered significant interest across various scientific fields. Understanding this tool involves delving into its mechanisms and potential applications as well as considering the ethical ramifications it presents.
The emergence of CRISPR has transformed the research paradigm. This section outlines the main findings related to its development and operational mechanics, while also setting the stage for subsequent discussions on its applications in medicine and agriculture. A focus on ethical considerations is crucial, as the power to edit genes brings forth questions about biodiversity, food security, and moral responsibility.
Research Overview
Key Findings
The core of CRISPR's function hinges on its ability to target specific DNA sequences. Key elements of its capability include:
- Targeted Gene Editing: CRISPR can accurately alter DNA at predetermined locations.
- Versatility: It is applicable in various organisms, from bacteria to humans.
- Efficiency: The technique allows rapid experimentation in gene editing.
Furthermore, CRISPR has shown promise in treating genetic disorders, modifying crop traits for resilience, and even combating infectious diseases.
Study Methodology
Research into CRISPR mechanism typically employs a variety of methodologies, including:
- Molecular Cloning: Essential for gene insertion and modification processes.
- Model Organisms: Laboratory models such as Escherichia coli and Drosophila melanogaster are frequently used to study gene editing results.
- In Vitro Techniques: Allow researchers to analyze how CRISPR interacts with the target DNA outside a living organism.
Each of these methodologies contributes to a broader understanding of CRISPR's role in contemporary biological research, providing a solid foundation for its varied applications.
Background and Context
Historical Background
CRISPR's journey began in the 1980s, but its functional potential was not recognized until later. Scientists first identified the CRISPR sequence in bacterial genomes in 1987, which were initially thought to be a form of viral defense system. The breakthrough came in 2012 when Jennifer Doudna and Emmanuelle Charpentier demonstrated its use for precise genome editing. This marked a critical shift in genetic engineering.
Current Trends in the Field
Today, applications of CRISPR span multiple sectors. In medical research, it has been pivotal in developing advanced therapies, particularly for genetic disorders like sickle cell anemia. In agriculture, CRISPR is used to create crops with improved resistance to pests and environmental stress.
As interest in precision medicine and sustainable agriculture grows, the role of CRISPR technology will likely expand. Subsequently, understanding how to harness its power responsibly is imperative for scientists and policymakers alike.
Understanding CRISPR
CRISPR technology stands at the forefront of genetic research today. This section aims to elucidate the significance of comprehending CRISPR in the broader context of scientific discovery and its various applications. Understanding how CRISPR works allows researchers to manipulate genetic material with unprecedented precision. This not only benefits scientific inquiry but also opens doors to profound innovations in medicine, agriculture, and beyond.
The benefits of mastering CRISPR techniques extend further than mere application. It involves an appreciation of the ethical considerations tied to genetic editing. Potential benefits include the treatment of genetic disorders, enhancements in crop resilience, and advancements in ecological preservation. However, it is crucial to recognize the implications those benefits hold for society. With the ability to edit genes comes the responsibility to navigate the ethical landscapes that emerge.
Definition and Overview
CRISPR, short for Clustered Regularly Interspaced Short Palindromic Repeats, is a revolutionary tool for genetic modification. It operates via a natural defense mechanism found in bacteria. When bacteria encounter viral DNA, they incorporate segments of that DNA into their genome. This allows them to “remember” the virus and fight it off in the future. By harnessing this system, scientists can target specific genes within an organism’s DNA for modification.
CRISPR technology allows for precise cuts in DNA. These cuts can be used to either disable genes or replace them with modified versions. This technology has gained immense popularity due to its efficiency and relative simplicity compared to previous genetic editing methods such as TALENs or ZFNs. As a result, CRISPR has become an essential tool in modern biology and genetics.
Historical Context
The journey of CRISPR began in the late 1980s when scientists first identified the unique sequences in bacterial DNA. Significant progress was made in 2012 when Jennifer Doudna and Emmanuelle Charpentier demonstrated the potential of CRISPR for targeted gene editing. Their work marked a pivotal moment in genetics, garnering attention not only within scientific communities but also in public discourse.
The implications of their discovery rapidly spread through various fields, leading to an explosive growth in CRISPR-related research. By 2015, CRISPR had captured the interest of investors and biotech firms. As a result, numerous applications emerged, from disease treatments to agricultural enhancements, forever changing the landscape of genetic research.
Key Terminology
To effectively engage with CRISPR discussions, understanding key terms is paramount. Here are some essential words and phrases:
- Cas9: A protein that acts as a molecular scissors, cutting the DNA at precise locations.
- Guide RNA: A short RNA sequence that directs the Cas9 protein to the specific area of DNA to be edited.
- Gene Editing: The process of making changes to the DNA sequence of an organism.
Understanding these terms provides a solid foundation for exploring deeper topics related to CRISPR, its mechanisms, applications, and the ethical considerations that arise.

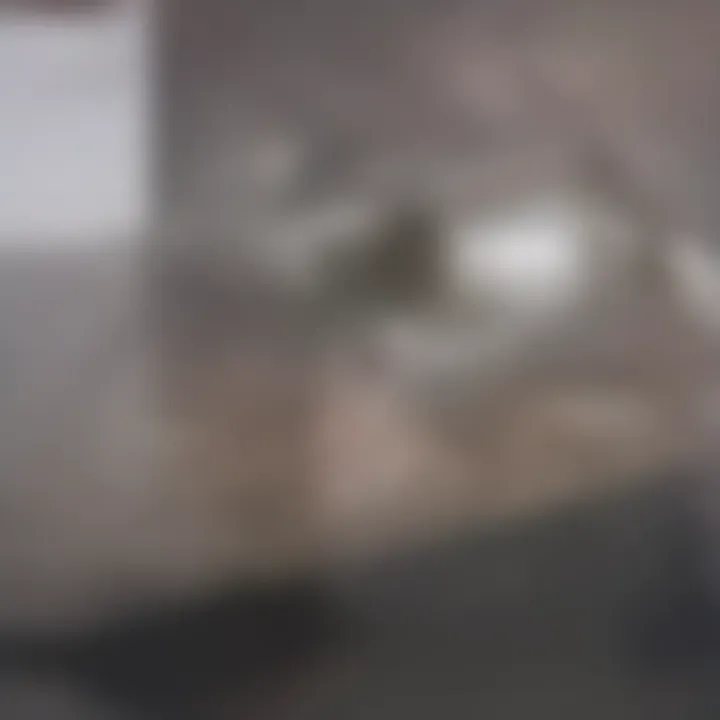
"Understanding CRISPR is not merely an academic pursuit; it's a step towards harnessing the potential of genetic engineering for socially beneficial applications."
With a firm grasp of CRISPR's definition, historical context, and key terminology, readers can appreciate the nuanced discussions that follow in this article.
Mechanisms of CRISPR/Cas9
Understanding the mechanisms behind CRISPR/Cas9 is crucial for realizing its potential across various fields. The effective deployment of this gene-editing technology hinges on its underlying biological processes. This section will elucidate the core components and functions involved, thus providing context for its applications.
Structure and Function of CRISPR
CRISPR, short for Clustered Regularly Interspaced Short Palindromic Repeats, is a segment of DNA containing short, repeated sequences. These sequences are integral as they serve as a genetic memory for the organism. The presence of spacers within these sequences correlates to past viral infections, allowing the organism to recognize and fight the microbes upon subsequent encounters.
A notable aspect of CRISPR's structure is its association with the Cas (CRISPR-associated) proteins. The Cas proteins play a pivotal role in the adaptive immune response, particularly in bacteria and archaea. Their main function is to identify and degrade foreign genetic material.
Role of the Cas9 Protein
Cas9 is arguably the most important protein in the CRISPR system. This endonuclease facilitates the targeted modification of DNA. It possesses a dual function: recognizing and binding to specific DNA sequences guided by RNA and introducing double-strand breaks at those sites. The precision of Cas9 is enhanced by its capability to distinguish the target DNA from non-target sequences based on complementary base pairing.
Cas9's role can also be defined through its complex with guide RNA. This RNA is instrumental for specificity, as it directs Cas9 to the exact locus on the DNA that requires editing. A critical consideration for Cas9 is its off-target effects, which highlight the need for meticulous design to avoid unintended modifications in the genome.
Guide RNA: Design and Functionality
The guide RNA is a fundamental component of the CRISPR/Cas9 mechanism. It is composed of two regions: a 20-nucleotide sequence that is complementary to the target DNA, and a scaffold region that binds to the Cas9 protein. The design of this RNA is paramount; it determines the accuracy of the editing process.
Creating an effective guide RNA involves a balance of specificity and efficiency. Researchers often utilize computational tools to predict off-target interactions, thereby improving the design phase. The success of the CRISPR technology largely depends on how well the guide RNA can navigate the complexities of the target genome without causing unintended alterations.
Mechanism of Target DNA Modification
The process of modifying target DNA using CRISPR/Cas9 involves several critical steps. Once the guide RNA and Cas9 form a complex, this structure binds to the target DNA. If the base pairing is correct, Cas9 introduces a double-strand break at the intended site. This break activates the cell's repair mechanisms, which can either induce non-homologous end joining or homology-directed repair.
- Non-homologous end joining tends to be error-prone and may result in insertions or deletions at the target site, thereby disrupting gene function.
- Homology-directed repair, on the other hand, can facilitate more precise modifications if a donor DNA template is provided. This method is pivotal for accurate gene editing and correcting mutations.
Overall, understanding these mechanisms allows researchers to harness CRISPR technology more effectively. As this field of study continues to evolve, it opens the door for advancements in medicine, agriculture, and beyond. The intricacies of CRISPR/Cas9 applications underscore its transformative capacity in a variety of contexts.
Applications of CRISPR Technology
The applications of CRISPR technology are vast and varied. Its significance lies in its potential to revolutionize numerous fields, particularly in biomedical research, therapeutics, agriculture, and environmental science. CRISPR's precise editing capabilities allow for unprecedented levels of targeted intervention, making it a powerful tool in advancing scientific understanding and practical applications. This section will explore these areas in detail, providing insight into the benefits and considerations of utilizing CRISPR technology.
CRISPR in Biomedical Research
CRISPR technology is fundamentally changing biomedical research. Its ability to edit genes with high precision enables scientists to investigate genetic functions and disease mechanisms more effectively. Before CRISPR, the tools available for gene editing were less precise and often limited in scope. The introduction of CRISPR has allowed for easier modeling of diseases in various organisms, including mice and cell lines.
Some key points of CRISPR's role in biomedical research include:
- Gene Function Analysis: Researchers can knock out specific genes to understand their roles in health and disease.
- Disease Modeling: Creation of genetically modified models that mimic human diseases, aiding drug development and testing.
- Screening for Therapeutics: High-throughput screening methods can identify potential drugs targeting specific genes.
In summary, CRISPR has become indispensable in unraveling the complexities of genetic disorders and is paving the way for innovative research methodologies.
Therapeutic Applications
The therapeutic potential of CRISPR is immense. From treating genetic disorders to hindering the progression of cancer, the possibilities seem endless. Clinical trials have already begun to test CRISPR-based therapies, showing promising preliminary results.
Some therapeutic applications include:
- Genetic Disease Correction: Targeting mutations that cause diseases like cystic fibrosis and sickle cell anemia.
- Cancer Treatment: Editing immune cells to better recognize and attack cancer cells, a process known as CAR-T cell therapy.
- Infectious Diseases: Developing treatments aimed at targeting viral genomes, potentially altering the course of viral infections.
Therapeutic CRISPR applications raise important questions about safety and efficacy, but they also offer hope for previously untreatable conditions.
Agricultural Biotechnology
CRISPR's impact on agriculture is another area of considerable advancement. With the growing global population and environmental challenges, CRISPR offers methods to enhance crop resilience and productivity.
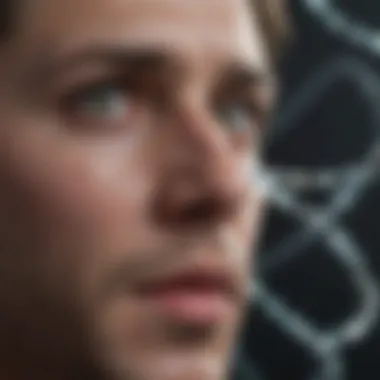
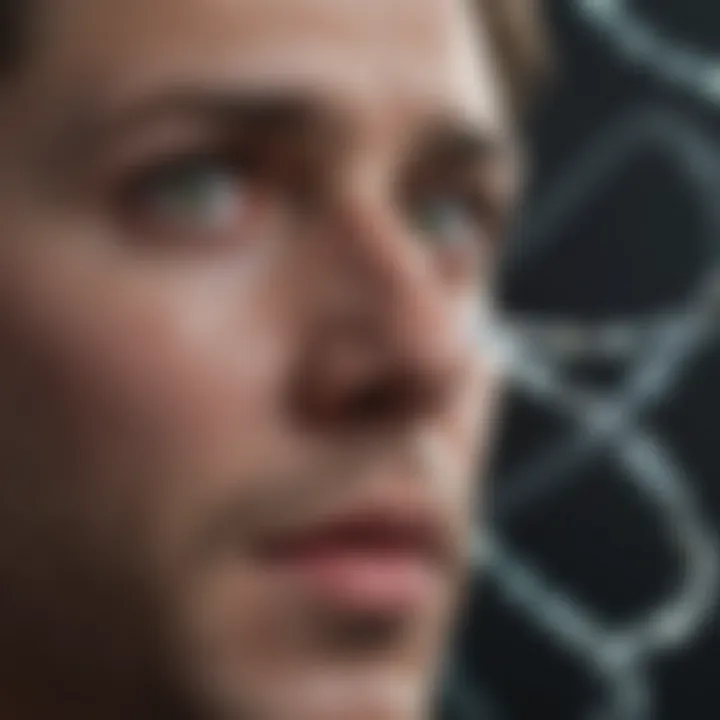
Important points include:
- Enhanced Crop Traits: Editing traits to resist pests, tolerate drought, or improve nutritional content.
- Faster Breeding Programs: Traditional methods of breeding can take many years, while CRISPR can create desired traits in a fraction of the time.
- Sustainable Practices: Reducing reliance on chemical pesticides and fertilizers leads to more sustainable agriculture.
These innovations have the potential to increase food security and provide solutions to agronomic challenges.
Environmental and Ecological Applications
CRISPR technology also finds applications within environmental science. It can be leveraged to understand ecosystems and manage ecological challenges, including conservation efforts.
Key aspects of environmental applications include:
- Biodiversity Conservation: Using CRISPR to edit populations to resist disease or adapt to changing climates.
- Invasive Species Control: Targeting invasive species with gene drives to control their populations and mitigate ecological harm.
- Bioremediation: Editing microbes to enhance their abilities to break down pollutants in various environments.
These applications of CRISPR in the environment highlight how gene editing can be utilized as a tool for ecological restoration and resilience against anthropogenic pressures.
Ethical Considerations
Ethical considerations are crucial when discussing CRISPR technology. As this powerful gene-editing tool evolves, its implications stretch into various domains, from medicine to agriculture. It is necessary to address the complexities associated with gene editing, especially concerning human subjects. The discussions around these ethical boundaries help to shape policies and regulations that can ensure responsible use of such technologies.
Ethics of Gene Editing in Humans
Gene editing in humans introduces profound ethical dilemmas. One primary concern is the distinction between therapeutic and enhancement purposes. Therapeutic gene editing aims to prevent or treat diseases, while enhancement can lead to modifications for non-medical purposes, such as physical appearance or intelligence. This raises questions about equity and access. If gene editing becomes available only to certain socioeconomic groups, it could exacerbate existing disparities.
Another critical aspect is the potential for unintended consequences in the human germline. Changes made at this level can be passed down through generations, possibly leading to unforeseen health issues. This possibility necessitates a cautionary approach to editing the genes of embryos or reproductive cells.
Additionally, the concept of consent becomes complicated. When editing genes in embryos, it is impossible to obtain consent from the future individual. This aspect challenges the fundamental principles of autonomy and respect for persons in bioethics.
Regulatory Frameworks and Guidelines
The regulatory framework surrounding CRISPR and gene editing is still developing. Various countries have started to establish guidelines that govern the research and application of these technologies. The frameworks often fall into three categories: regulation of research, clinical applications, and agricultural modifications.
In the United States, the National Institutes of Health (NIH) and the Food and Drug Administration (FDA) play a pivotal role. The NIH oversees the ethical conduct of research, ensuring that it complies with safety protocols. The FDA, on the other hand, evaluates the safety and efficacy of gene-editing therapies before they enter the clinical setting.
Internationally, organizations like the World Health Organization (WHO) and UNESCO contribute to setting norms in gene editing. In 2021, WHO released a report outlining recommendations for governance in human genome editing, stressing transparency, oversight, and public engagement.
"The need for robust regulatory frameworks cannot be overstated as technological advancements continue to challenge existing laws and ethical guidelines."
The international nature of gene editing means that inconsistent regulations can lead to ethical and safety issues. Therefore, collaboration among global stakeholders is necessary to ensure that CRISPR research and applications proceed responsibly.
Public Perception and Acceptance
Public perception of CRISPR technology varies widely and influences its acceptance. Trust in scientific advances depends heavily on public understanding and communication efforts. Many individuals express concern regarding the potential risks associated with gene editing, leading to fear and hesitation toward its adoption.
Surveys show a dichotomy in attitudes. While many people see the potential for CRISPR to treat genetic diseases positively, there is significant apprehension over its capability to create 'designer babies.' Misunderstanding the technology often fuels fears regarding its misuse. Therefore, public health communication plays a crucial role in educating the community about CRISPR's mechanisms and its ethical implications.
Engagement with the public is essential. Scientists and ethicists should prioritize transparent dialogues about CRISPR, focusing on both benefits and risks. When public engagement occurs, it fosters a sense of inclusivity in decision-making processes surrounding biotechnological advancements. This can lead to balanced policies that reflect societal values and norms, ultimately paving the way for more robust support of responsible research and application of CRISPR technology.
Challenges and Limitations
Understanding the challenges and limitations of CRISPR technology is crucial for grasping its practical implementation and future prospects. While CRISPR holds significant potential in various fields, several significant factors can hinder its effectiveness and acceptance. Addressing these challenges is relevant not only for scientific research but also for regulatory and societal implications. This section dissects critical issues surrounding CRISPR, including off-target effects, delivery mechanisms, and societal and legal challenges.
Off-Target Effects
One of the primary concerns with CRISPR technology is the occurrence of off-target effects. These happen when the CRISPR system unintentionally edits genes other than the targeted DNA sequence. The implications can be serious, as unintended modifications can lead to harmful mutations, unpredictable cellular behavior, or even oncogenesis. Research shows that off-target modifications may vary based on the guide RNA design and the target genome. As scientists work to enhance the precision of CRISPR, the importance of meticulous design and rigorous testing cannot be overstated.
Minimizing off-target effects is crucial not only for therapeutic applications but also for enhancing the reliability of CRISPR in research settings. Advances are being made, such as employing high-specificity versions of Cas9 enzymes, which may reduce unintended interactions. However, ongoing investigations and transparent reporting are essential to better understand the extent and nature of off-target effects in specific contexts.
Delivery Mechanisms
Effective delivery of CRISPR components into cells poses another challenge. The system requires not only the Cas9 protein and guide RNA but also a method of getting these entities into the target cells. Currently, several delivery mechanisms are in practice, each with its advantages and limitations.
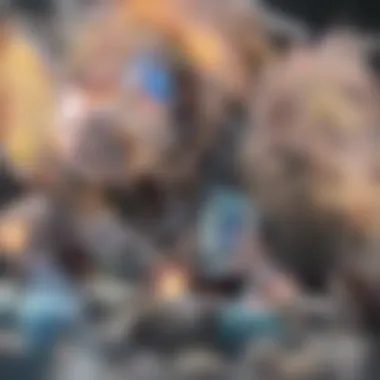
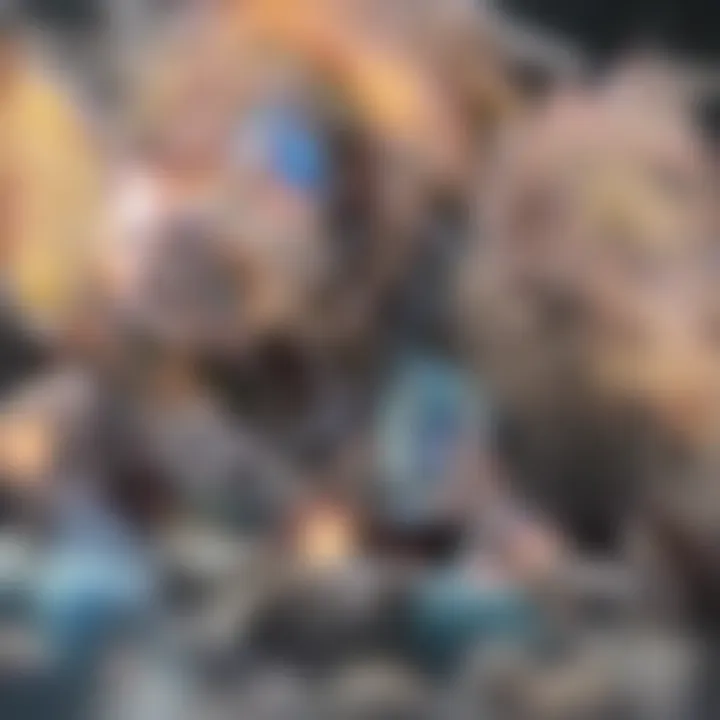
- Viral Vectors: Often used due to their efficiency in gene delivery. However, they can provoke immune responses and are limited in size capacity.
- Liposomes: These artificial vesicles can carry CRISPR components without harmful effects but may not always achieve high efficiency in targeting cells.
- Electroporation: This method uses electrical pulses to facilitate entry into cells. It is effective, but not suitable for all cell types and can result in cell damage.
- Nanoparticles: They offer a promising avenue by allowing specific targeting capabilities. Yet, their scalability and stability pose challenges.
As researchers continue to explore innovative delivery systems, it is essential to weigh the benefits against the limitations of each to ensure the efficiency and safety of CRISPR applications.
Societal and Legal Challenges
The implementation of CRISPR technology raises profound societal and legal questions. Public perception plays a significant role in shaping regulatory policies and influencing research funding. Many people express concerns about the ethical implications of gene editing, particularly in humans. Fears of designer babies or unregulated enhancements are prevalent. Such issues lead to calls for strict regulations and guidelines governing its use.
Legal frameworks vary significantly across countries, reflecting different societal values and priorities. Some nations have embraced CRISPR for agricultural advancements and medical therapies, while others impose stringent restrictions. Further complicating matters are the intellectual property rights related to CRISPR patents, which can impact research and development globally.
Regulating CRISPR use requires careful consideration of both opportunities and risks. Open discussions among researchers, ethicists, legal experts, and the public are vital to forging a common ground that balances innovation with responsible oversight.
The integration of CRISPR into various fields is not simply a scientific challenge; it also encapsulates complex societal and legal dimensions that must be addressed for sustainable progress.
In sum, the challenges and limitations of CRISPR technology encompass a broad spectrum of issues that must be carefully navigated. Awareness of off-target effects, efficient delivery mechanisms, and societal attitudes will play a significant role in the future trajectory of CRISPR research and applications.
Future Prospects of CRISPR Technology
The future of CRISPR technology holds significant importance in the context of genetic engineering, medical therapy, and agricultural innovation. This section aims to illuminate various advancements anticipated in CRISPR methodologies, the promising impact on personalized medicine, and the essential role it could play in ensuring global food security.
Advancements in CRISPR Techniques
Research is continually yielding new techniques to enhance CRISPR's efficacy and specificity. Recent innovations include CRISPR variations like CRISPR/Cas12 and CRISPR/Cas13, which have potential beyond traditional gene editing. Cas12 is noted for its ability to target DNA with increased accuracy and fewer off-target effects. Cas13, on the other hand, can edit RNA, which opens avenues for treating diseases where DNA editing isn't effective.
Additionally, advancements in delivery systems are crucial. Viral vectors, nanoparticles, and other means are evolving to improve how CRISPR components enter cells effectively. Scientists also explore base editing and prime editing, which offer even more precise ways to modify genetic sequences without introducing double-stranded breaks in DNA.
"The adaptability of CRISPR technology signifies a turning point in genome editing, enhancing precision and expanding its applications."
Through these advancements, CRISPR's potential will continue to broaden, paving the way for innovative approaches to some of humanity's most pressing challenges.
Potential Impact on Personalized Medicine
Personalized medicine aims to tailor healthcare based on individual genetic profiles. CRISPR technology is set to advance this field significantly. By enhancing gene therapy approaches, it allows for more targeted treatments of genetic conditions. Conditions like cystic fibrosis, sickle cell disease, and certain cancers may see revolutionary treatment options.
Furthermore, the integration of CRISPR with genomic sequencing technologies will facilitate more accurate diagnostics. Medical professionals can identify genetic predispositions to diseases and adopt proactive strategies accordingly. With CRISPR capabilities, researchers will enhance their understanding of complex diseases, ultimately helping to develop effective personalized therapies and improving patient outcomes.
CRISPR and Global Food Security
The growing global population demands innovative solutions to food production. CRISPR technology has great potential in this arena by enabling rapid development of crop varieties that are resistant to pests, diseases, and environmental stresses like drought.
A few ways CRISPR can enhance food security include:
- Development of crops with improved nutritional content
- Creation of plants that require fewer chemical pesticides
- Breeding crops with extended shelf life and enhanced yields
These advancements could lead to sustainable agricultural practices that help meet food demands while reducing environmental impact. Likewise, CRISPR could facilitate the development of livestock with desirable traits, further contributing to food security.
The future of CRISPR technology is promising. With continued research, it may transform various sectors including healthcare and agriculture, addressing crucial global challenges. Ultimately, understanding these prospects is essential for fully grasping the implications of CRISPR on society.
End
The conclusion serves as a pivotal section in this article. It encapsulates the essential themes explored throughout our discussion on CRISPR technology, emphasizing its profound impact across various fields including biotechnology, medicine, and agriculture. In this final analysis, we synthesize the key insights derived from previous sections, reinforcing the importance of CRISPR technology in shaping the future of scientific research and practical applications.
Summarizing Key Insights
CRISPR technology is fundamentally altering how we understand and manipulate genetic materials. Key insights from this article include:
- Mechanisms of Action: CRISPR's ability to edit DNA with precision has revolutionized genetic engineering. The clarity of its operating mechanisms allows both researchers and practitioners to utilize it effectively, diminishing the chances of mishaps.
- Wide Applications: From developing therapies for genetic disorders to enhancing crop resilience in agricultural settings, the applications of CRISPR are vast and diverse. This versatility speaks to its significance in addressing critical global challenges.
- Ethical and Societal Considerations: Despite its potential, the ethical dilemmas surrounding CRISPR, such as gene editing in humans, demand careful consideration. Ongoing debates highlight the need for responsible governance and public engagement to navigate these moral complexities.
"The transformative power of CRISPR is tempered by ethical responsibilities that cannot be overlooked."
The Path Forward for CRISPR Research
The future of CRISPR technology is promising yet still fraught with challenges. As research progresses, several directions emerge:
- Enhanced Precision: Ongoing advancements aim to minimize off-target effects, increasing the reliability of CRISPR edits.
- Regulatory Developments: Establishing clear frameworks will be crucial in guiding the ethical deployment of CRISPR technology. This ensures research aligns with societal values and safety standards.
- Global Collaboration: The complexity of genetic issues and their implications requires a cooperative global approach. Sharing knowledge can facilitate breakthroughs that benefit humanity as a whole.
- Increased Public Awareness: Promoting an informed public discourse about CRISPR will help demystify its applications and implications. Engaging with the public fosters understanding and trust.
To conclude, CRISPR technology stands at the forefront of a scientific revolution. The path forward requires a balance between innovation and ethical stewardship to harness its full benefits for future generations.