Exploring the Lifespan of Lithium Batteries: Factors & Insights
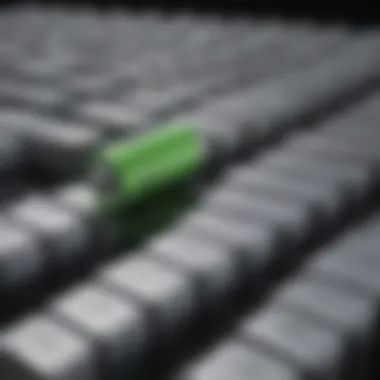
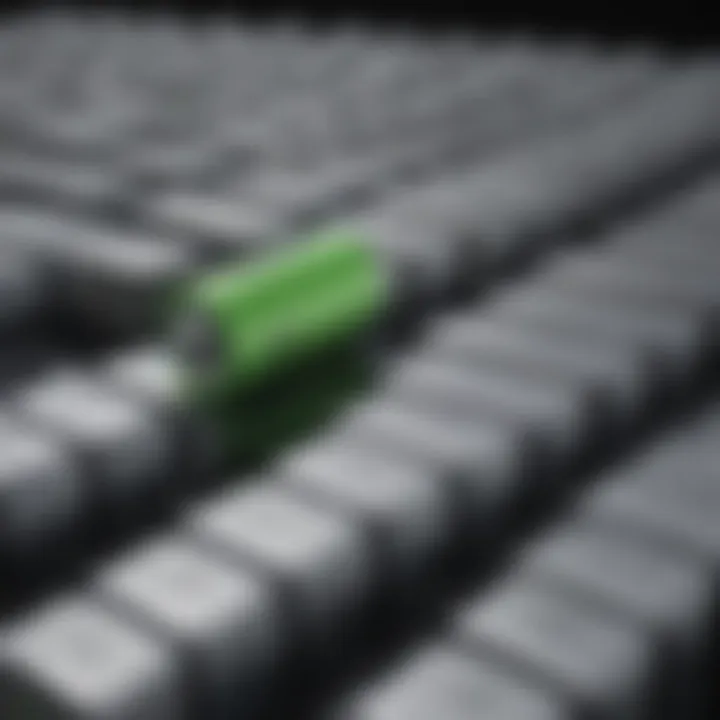
Research Overview
Lithium batteries, specifically lithium-ion technology, have transformed modern energy storage. Their prevalence in devices like smartphones, electric vehicles, and renewable energy systems underscores their importance. Understanding the lifespan of these batteries is critical, as it directly impacts performance and sustainability across various applications. This section aims to provide a detailed analysis of the factors influencing battery longevity, supported by recent research findings.
Key Findings
Research indicates that several factors contribute to the lifespan of lithium batteries:
- Cycle Depth: Shallow cycling can extend battery life, while deep cycling may lead to accelerated degradation.
- Temperature Effects: High temperatures can cause thermal stress and lead to premature failure. Conversely, extremely low temperatures can result in reduced efficiency.
- Electrolyte Composition: Variations in the electrolyte's chemical composition can significantly affect battery performance and durability.
- Charge Techniques: Smart charging practices, such as using optimal voltage settings, have shown to enhance the lifespan.
Study Methodology
To gather this information, various studies utilized a blend of experimental tests and computational models. For instance, researchers conducted accelerated life tests simulating different operating conditions, allowing them to measure the impact of specific stressors on the battery's performance over time. Comprehensive data analytics were employed to interpret results and identify key degradation mechanisms.
Background and Context
Understanding lithium batteries requires an appreciation of their evolution and current trends that shape the field.
Historical Background
Lithium-ion batteries emerged in the early 1990s, providing superior energy density compared to previous technologies such as nickel-cadmium. The innovation heralded a new era in consumer electronics. Over decades, advancements in materials science have continually optimized these batteries, yet challenges remain regarding their lifespan.
Current Trends in the Field
Recently, there has been a marked increase in sustainable practices related to battery production and disposal. Research focuses on:
- Second Life Applications: Repurposing used batteries for energy storage in residential or industrial settings.
- Recycling Technologies: Developing methods to reclaim valuable materials from exhausted batteries.
- Alternative Chemistries: Exploring non-lithium options, like sodium-ion batteries, to mitigate supply chain concerns.
"The lifespan of lithium batteries not only effects the technology but also financial sustainability and environmental practices overall."
The narratives surrounding lithium batteries are evolving, driven by an urgent need for greener solutions. Understanding the lifespan and methods for optimization becomes increasingly important for researchers and industry professionals alike.
Prologue to Lithium Batteries
Lithium batteries, pivotal in modern energy storage, are the backbone of a multitude of technologies. Understanding their lifespan is crucial since they power everything from smartphones to electric vehicles. The efficiency and sustainability of these batteries directly influence how we utilize energy in daily life.
Historical Context
Lithium-ion technology emerged in the 1970s, focusing on improving energy density and cycle life. The first commercial lithium-ion battery was introduced by Sony in 1991. This development marked a turning point, allowing portable electronic devices to become more mobile and convenient. Over the decades, research enhanced battery performance, making them safer and more reliable. The transition from earlier technologies, such as nickel-cadmium batteries, showcased a shift not only in performance but also in environmental considerations. As awareness of the eco-footprint increased, lithium provided a favorable alternative owing to its abundance and efficiency. In addition, collaborations among researchers, manufacturers, and regulatory bodies have played a significant role in advancing the technology, adhering to improved safety standards and sustainability measures.
Current Applications
Today, lithium batteries function in various sectors. In consumer electronics, they are essential for powering smartphones, laptops, and tablets. In the automotive industry, electric vehicles like those produced by Tesla rely heavily on lithium-ion technology to achieve long ranges and quick charging capabilities. Furthermore, lithium batteries are also critical in renewable energy systems, serving as storage solutions for solar and wind energy. This versatility not only highlights their importance but also underscores the need for constant improvements in their performance and lifespan. Dedicated research focuses on enhancing battery management systems (BMS) to optimize charging cycles and maximize the effective lifespan of these batteries, ensuring optimal usage across all applications.
"Lithium batteries have revolutionized the way we think about energy storage and utilization, impacting every facet of our modern lives."
Overall, understanding lithium batteries is not just a technical necessity; it is crucial for addressing future energy demands and developing sustainable practices.
Chemical Composition of Lithium Batteries
The chemical composition of lithium batteries plays a critical role in determining their performance, safety, and overall lifespan. Understanding the materials used in these batteries can provide insights into how they function and the implications for their application across various industries. Each component, whether solid or liquid, contributes to the efficiency of energy storage and release. The objective is to ensure that every part maximizes energy density while minimizing potential hazards.
Electrode Materials
The electrodes in lithium batteries are where the electrochemical reactions occur. They consist primarily of lithium compounds, but the exact materials can vary significantly. Common positive (cathode) materials include lithium cobalt oxide, lithium iron phosphate, and lithium manganese oxide. These materials are chosen for their ability to facilitate lithium ion movement while remaining stable during charge and discharge cycles.
On the negative (anode) side, graphite is the most prevalent material due to its satisfactory balance of capacity and conductivity. Recently, other materials like silicon have gained attention for their potential to increase capacity and performance. However, the use of silicon must be approached with caution due to challenges related to expansion during charging.
The ultimate choice of electrode materials not only affects energy capacity but also impacts the thermal behavior and longevity of the battery. An optimal combination of materials ensures efficient operation and durability.
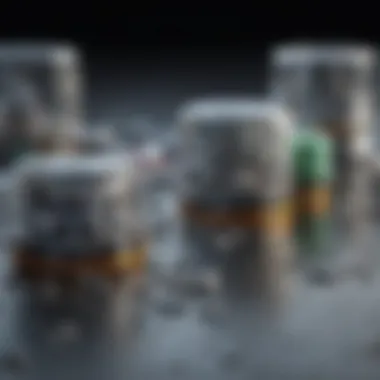
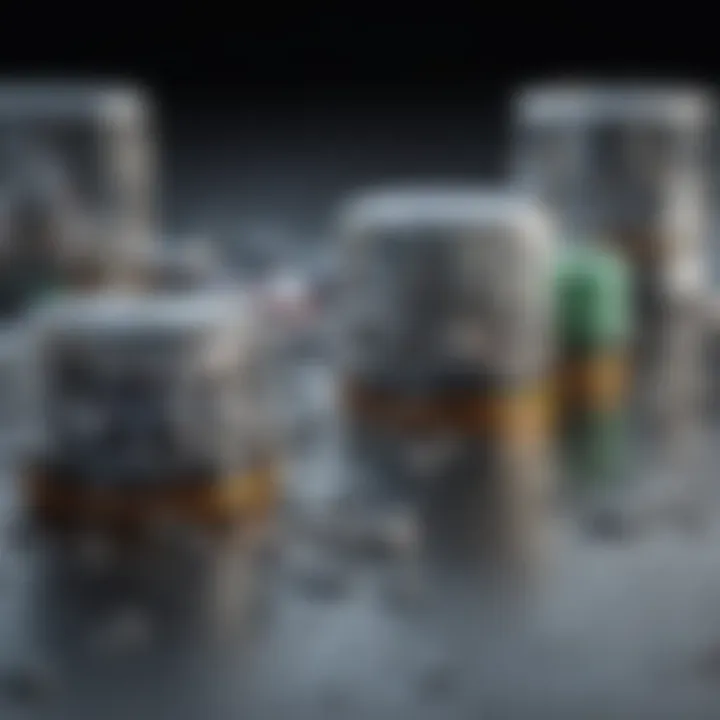
Electrolyte Composition
The electrolyte is crucial in lithium batteries as it facilitates the movement of lithium ions between the electrodes. Most lithium batteries utilize liquid organic electrolytes, which consist of lithium salts dissolved in organic solvents. Popular choices include lithium hexafluorophosphate, as it provides good ion conductivity and stability.
In recent years, there has been a growing interest in solid-state electrolytes. These substances promise higher safety and energy densities compared to their liquid counterparts by eliminating flammability risks. However, current solid-state materials face challenges in terms of conductivity and compatibility with traditional electrode materials.
The composition of the electrolyte can significantly influence a battery's performance. For example, an electrolyte with high ion conductivity can improve charge rates and overall efficiency. Conversely, incompatible materials can lead to poor cycle life and safety risks.
"The chemical composition of lithium batteries is not just a technical detail; it essentialy forms the foundation upon which future advancements can be built."
By understanding the intricacies of these materials—both electrodes and electrolytes—researchers can develop better, more sustainable battery technologies that meet the growing demands of everyone from electric vehicle manufacturers to consumer electronics.
This section aims to shed light on the pivotal role that chemical composition plays in the efficacy and longevity of lithium batteries, guiding further exploration into their application and innovation.
Factors Influencing Battery Lifespan
The lifespan of lithium batteries is a critical topic in both academic and industry contexts. Understanding how various factors influence durability and reliability can lead to improved practices in charging, usage, and recycling. This section delves into the specific elements impacting battery lifespan and their implications in real-world applications.
Temperature Effects
Temperature plays a significant role in the lifespan of lithium batteries. Operating at extreme temperatures can accelerate degradation processes. A higher ambient temperature often leads to increased self-discharge rates. This means that the battery loses charge even when not in use. For example, temperatures above 40°C can degrade the electrolyte and interfere with ion movement, leading to quicker capacity fade. Conversely, low temperatures can reduce the efficiency of charge and discharge cycles.
It is crucial to maintain batteries in optimal temperature ranges. Manufacturers typically recommend a range between 20°C and 25°C for ideal operation. Exceeding these temperatures may cause overheating, while colder conditions can reduce performance and efficiency.
"Maintaining lithium batteries within optimal temperature ranges is vital for extending their lifespan and effective performance."
Charge Cycles
The number of charge cycles directly impacts the overall life of a lithium battery. Each cycle consists of a discharge followed by a complete charge. With each cycle, certain chemical reactions occur that can gradually diminish the battery capacity. Typically, lithium batteries have a specified number of cycles they can endure before reaching significant capacity loss, often cited around 300 to 500 cycles for conventional lithium-ion models.
Understanding how to optimize charge cycles can enhance battery longevity. For instance, partial charging rather than full cycles can minimize stress on the battery chemistry. Many experts recommend avoiding frequent deep discharges since they place additional strain on the battery and hasten degradation.
Depth of Discharge
The depth of discharge (DoD) refers to the percentage of the battery's capacity that has been used. A lower depth of discharge generally leads to a longer lifespan. For instance, continuously discharging a lithium battery to 80% can significantly reduce its lifespan compared to a more shallow discharge of only 30%. The battery chemistry profoundly influences the effects of DoD. High DoD cycles can lead to lithium plating, solid electrolyte interface formation, and ultimately fail.
To optimize battery lifespan, it is advisable to maintain a higher state of charge and perform regular maintenance checks on the battery management system (BMS). This approach helps in understanding battery health and guiding charging practices accordingly.
Mechanisms of Degradation
Understanding the mechanisms of degradation in lithium batteries is vital for maximizing their lifespan and performance. As technology advances, the reliance on lithium batteries increases, particularly in sectors like electric vehicles and consumer electronics. Therefore, comprehending how these batteries deteriorate over time contributes to developing more effective usage practices. It can lead to innovations in battery design and management systems. This section explores key forms of degradation impacting battery performance.
Solid Electrolyte Interface (SEI) Formation
The Solid Electrolyte Interface (SEI) is a crucial layer formed on the surface of the electrode during initial charge cycles of lithium batteries. This layer arises from complex chemical reactions between the electrolyte and electrode materials. Although the SEI is essential for battery function, its establishment comes at a cost. The formation process can consume lithium ions and reduce overall capacity.
A robust SEI can enhance battery stability and reduce unwanted electrolyte decomposition. However, should the SEI become too thick or unstable, it can significantly impair battery efficiency. Effective monitoring and understanding of SEI dynamics can lead to strategies that promote a stable interface, thus enhancing lifespan and performance of lithium batteries.
Lithium Plating
Lithium plating occurs when lithium deposits accumulate on the anode surface instead of being intercalated within the graphite structure. This phenomenon is more frequent during fast charging or in low-temperature environments. When lithium ions cannot adequately transition into the anode material, they form metallic lithium, which can lead to safety hazards and capacity loss.
Preventing lithium plating requires careful management of charging conditions. Adjusting the charge rates, increasing the temperature during charge cycles, or employing advanced battery management systems can help mitigate this issue. Understanding and addressing lithium plating is critical for improving battery reliability and efficiency.
Electrode Expansion and Shrinkage
During charge and discharge cycles, the electrodes in lithium batteries undergo expansion and shrinkage. This physical change is due to lithium ions moving in and out of the electrode material. While some degree of mechanical strain is acceptable, excessive expansion or shrinkage can lead to electrode cracking and loss of active material.
To combat this degradation mechanism, researchers are exploring various materials that possess better structural integrity and flexibility. Advanced electrode materials can reduce the impact of these dimensional changes, thereby enhancing durability and functionality. Ensuring effective electrode performance is a cornerstone for developing long-lasting lithium batteries.
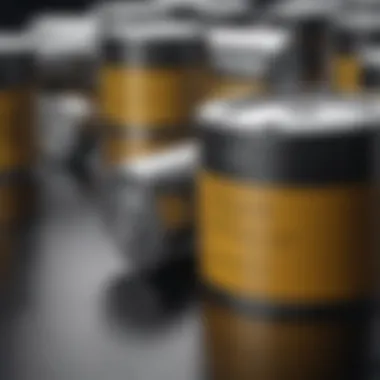
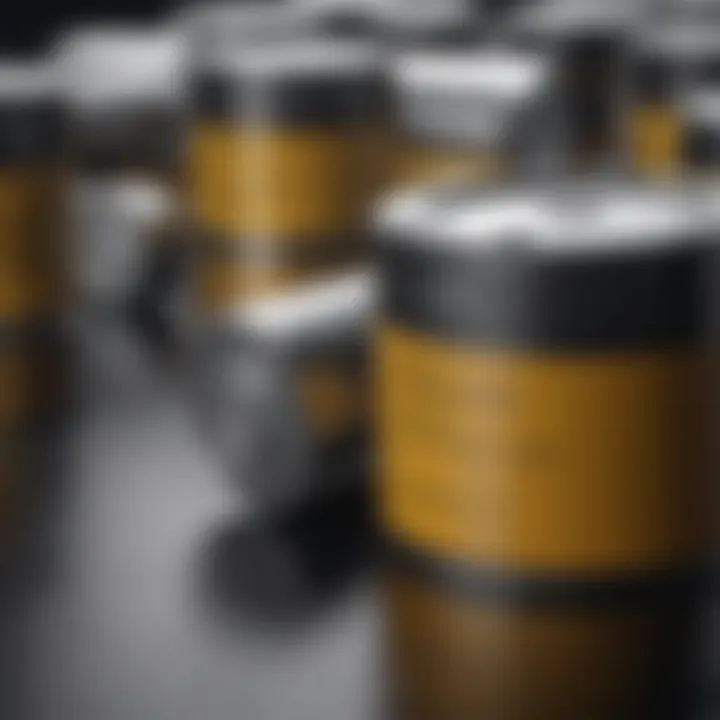
"Understanding these mechanisms provides insights that can lead to performance improvements in lithium batteries, influencing everything from basic research to industrial applications."
Measuring Battery Lifespan
Measuring the lifespan of lithium batteries is significant for several reasons. Knowing the lifespan helps users make informed choices about battery management and application. It affects not just performance, but also the economic aspects of battery usage. With a more precise understanding of lifespan, stakeholders can implement strategies that maximize utility and reduce costs. Moreover, discussions about sustainability connect closely with lifespan measurements. Batteries with longer lifespans create less waste and improve environmental outcomes.
Cycles and Calendar Life
Cycles and calendar life are critical metrics for assessing battery lifespan. Cycle life refers to the number of complete charge-discharge cycles a battery can undergo before its capacity falls below a specific threshold, usually 80%. For instance, the Tesla Model S utilizes lithium-ion batteries, designed to last approximately 1,500 cycles. This cycle life is valuable, but understanding how it relates to real-world use is essential. If a battery is used frequently in deep discharge conditions, its cycle life may decrease despite its theoretical capacity.
On the other hand, calendar life deals with the duration a battery can remain functional over time, independent of usage cycles. Factors influencing calendar life include temperature and humidity, which can have significant effects. Batteries stored at high temperatures may degrade faster than those kept at optimal conditions. Manufacturers often provide both cycle and calendar life ratings. This dual perspective helps in understanding how a battery performs over time, allowing users to choose batteries that best suit specific applications.
Capacity Fade Metrics
Capacity fade metrics are vital indicators of a battery's health over time. They measure how much usable energy a battery retains as it ages. Capacity fade occurs due to several factors, including chemical reactions within the battery, loss of active material, and increased internal resistance. The rate at which a battery fades affects its utility in practical applications. For example, if a lithium-ion battery in a smartphone loses capacity significantly within a couple of years, it forces users to replace it sooner than expected.
"Monitoring capacity fade is essential for planning replacements and understanding battery performance."
To quantify capacity fade, metrics such as the Coulombic efficiency and capacity retention rate are commonly employed. Coulombic efficiency measures the charge output against the charge input for each cycle, providing insight into the efficiency of the battery. Capacity retention rate, expressed as a percentage, indicates how much of the original capacity is maintained over time. For example, a battery maintaining 80% capacity after 5 years is deemed effective.
Improving Battery Lifespan
In the context of lithium batteries, improving lifespan is vital for several reasons. First, as these batteries power essential devices across sectors including electric vehicles and consumer electronics, their reliability directly affects user experience. Second, lengthening the operational life of lithium batteries can reduce total costs for consumers and industries alike. By minimizing replacements, users save resources and reduce waste, contributing to sustainable practices. Finally, understanding how to maximize battery lifespan aligns with broader goals of energy efficiency, crucial for advancing technology while minimizing environmental impacts.
Optimal Charging Practices
Effective charging practices play a significant role in extending the lifespan of lithium batteries. Users often overlook the implications of how they charge their devices. Here are some key practices:
- Avoid Full Charges: Regularly charging to 100 percent can stress battery chemistry. Keeping charge levels between 20 and 80 percent can minimize wear.
- Slow Charging: Utilizing slower charging options, such as trickle chargers, can be beneficial. Fast charging generates heat, which is detrimental for lithium batteries.
- Temperature Control: Always charge batteries in a moderate temperature range. Extreme cold or heat negatively impacts chemical stability.
By adhering to these practices, users can significantly impact the longevity of lithium batteries, enhancing performance and reliability.
Battery Management Systems (BMS)
Battery Management Systems are critical for managing the health of lithium batteries. A BMS monitors various parameters like voltage, current, and temperature throughout battery operation. The importance of this system cannot be overstated:
- Safety Monitoring: A BMS prevents overcharging and deep discharging, protecting the battery from conditions that could lead to failure.
- State of Charge (SOC) Estimation: It provides accurate readings of charge levels, guiding users in optimal usage and charging practices.
- Cell Balancing: Uneven charge distribution among battery cells can lead to shorter lifespan and performance issues. A BMS ensures even charging, thus prolonging life.
Implementing effective Battery Management Systems means understanding their role in protecting the investment that is lithium batteries. Long-term benefits include increased reliability and reduced maintenance costs.
"Extending the lifespan of lithium batteries is not only about technology but also about wise usage and management practices that can truly be game changers."
By focusing on optimal charging practices and leveraging Battery Management Systems, users can take significant steps toward enhancing the performance and lifespan of lithium batteries.
Impact of Environmental Factors
The impact of environmental factors on lithium batteries is a crucial aspect of understanding their lifespan. Environmental conditions such as temperature, humidity, and even altitude play significant roles in the performance and longevity of these batteries. Addressing these elements is essential for effective battery management and ensuring that devices operate under optimal conditions. An awareness of environmental impacts can lead to better design and usage practices for lithium batteries across various applications.
Temperature and Humidity Variations
Temperature and humidity are key environmental factors that affect the performance and degradation of lithium batteries.
- Temperature Extremes: Lithium batteries generally perform best at moderate temperatures. When exposed to high temperatures, the chemical reactions inside the battery can accelerate, leading to faster degradation. This can result in reduced capacity and increased risk of thermal runaway, which poses significant safety hazards. Conversely, low temperatures can impede ion movement within the battery, causing capacity loss during cold weather operations.
- Humidity Effects: Elevated humidity levels can lead to corrosion and short-circuiting within batteries. Humidity can affect the integrity of the electrodes and the quality of the electrolyte, reducing efficiency. If batteries are stored or operated in high-humidity environments, it could lead to premature failure.
- Optimizing Conditions: To improve lifespan, it is important to monitor and manage the environmental conditions where lithium batteries are utilized. Keeping batteries at stable temperatures and low humidity can enhance lifespan and performance. Understanding the specific operating ranges for the battery technology in use is essential for maximizing longevity.
Recycling and Disposal Considerations
The recycling and disposal of lithium batteries are as important as their performance aspects. These considerations not only affect the environment but also shape future applications of lithium-ion technology.
- Environmental Hazard: Improper disposal of lithium batteries poses environmental risks. They contain hazardous materials, which can contaminate soil and water if not handled properly. Therefore, responsible recycling and disposal practices are critical.
- Recycling Processes: Recycling lithium batteries involves processes that recover valuable materials such as lithium, cobalt, and nickel. These materials can be reused in new battery production, reducing the demand for raw materials and minimizing environmental impacts. Various companies are working on efficient recycling technologies that can extract these elements effectively.
- Legislation and Guidelines: Different regions have specific regulations governing the disposal of lithium batteries. Understanding these regulations is crucial for consumers and manufacturers alike. Initiatives promoting battery take-back programs have been instrumental in ensuring safe disposal.
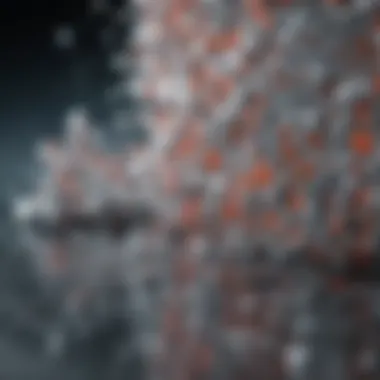
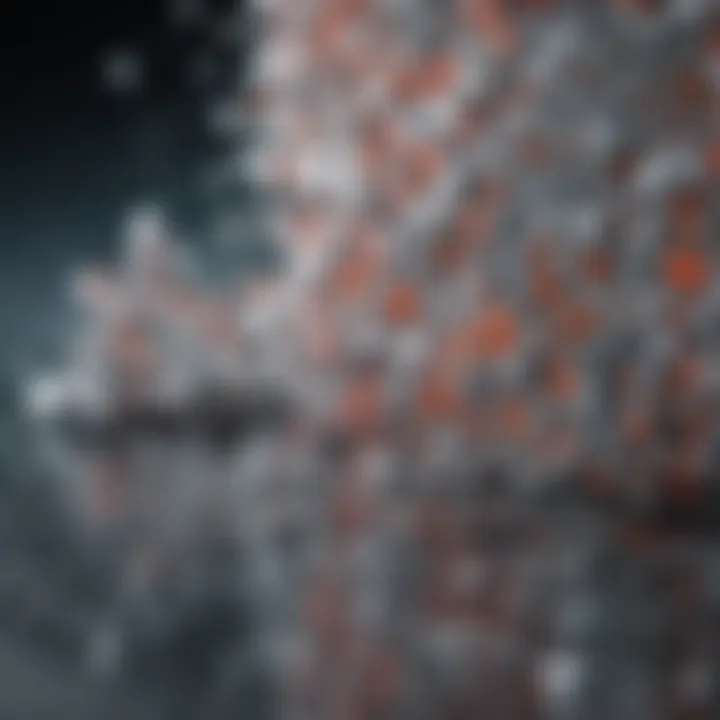
Proper handling of lithium batteries at the end of their life cycle is vital for both environmental protection and resource recovery.
Future Trends in Lithium Battery Technology
The future of lithium battery technology is paramount to various sectors, including automotive, consumer electronics, and renewable energy. As the demand for efficient energy storage grows, advancements in battery technology must keep pace to meet these challenges. This section discusses significant developments and shifts in the landscape of lithium batteries, particularly in solid-state batteries and advanced lithium-ion chemistries.
Solid-State Batteries
Solid-state batteries represent a pivotal evolution in lithium battery technology. Unlike traditional lithium-ion batteries, which use liquid electrolytes, solid-state designs employ solid electrolytes, significantly enhancing safety and efficiency. This change reduces the risk of leakage and flammability, leading to higher reliability for applications where safety is critical, such as electric vehicles.
Additionally, solid-state batteries can potentially offer greater energy density. This means more energy storage in the same physical space, making them ideal for compact devices without compromising performance. The longevity of these batteries is also promising; with the right materials, they may have improved cycle life and reduced decay rates when compared to their liquid counterparts. Some challenges persist, including manufacturing scale-up and cost-effectiveness, yet ongoing research continues to show promise in overcoming these barriers.
Advanced Lithium-Ion Chemistries
As the lithium-ion market matures, advanced chemistries in battery composition emerge as a focal point for innovation. Enhanced lithium-ion chemistries like lithium iron phosphate (LiFePO4) and lithium nickel manganese cobalt oxide (NMC) enable batteries that better balance energy density, power output, and thermal stability.
These advancements lead to batteries that last longer, charge faster, and operate more effectively under varying conditions. For example, LiFePO4 offers enhanced safety and longevity but has somewhat lower energy density. Meanwhile, NMC batteries provide better energy density but carry a risk of thermal runaway.
To ensure optimal performance, manufacturers often seek to balance these chemistries in customized configurations, thus producing batteries tailored to specific applications. This tailoring elevates the overall effectiveness of lithium batteries across diverse uses, from electric vehicles to personal gadgets and large-scale energy storage systems.
By exploring advanced lithium-ion chemistries, companies can push the boundaries of battery performance, ensuring that they meet the future’s energy demands.
In summary, the future trends in lithium battery technology, particularly regarding solid-state batteries and advanced lithium-ion chemistries, promise a landscape where efficiency, safety, and performance drive innovation. Addressing the challenges of these advancements is critical as they shape the future of energy storage.
Case Studies and Real-World Examples
Case studies and real-world examples are essential to understanding the practical implications of lithium battery lifespan. These illustrative narratives provide insight into how theoretical concepts apply in actual situations. They help readers grasp the nuances of lithium battery performance across different applications. Examining case studies can reveal common challenges, innovative solutions, and the adaptability of lithium technologies in various sectors.
Electric Vehicles
Electric vehicles (EVs) serve as a prominent example of lithium battery use. The automotive industry relies heavily on lithium-ion batteries for energy storage and propulsion. These batteries are chosen for their relatively high energy density and ability to undergo numerous charge cycles. Case studies of manufacturers like Tesla and Nissan shed light on practical experiences concerning battery lifespan.
For instance, Tesla's Model S has demonstrated longevity in its battery life under optimal conditions, often exceeding 300 miles per charge. However, some users have reported premature battery degradation due to rapid charging and temperature extremes. This has prompted Tesla to enhance their Battery Management Systems to optimize performance based on user habits.
The importance of temperature management is clear in these cases. Higher temperatures may accelerate degradation of the battery materials, which leads to shortened lifespan. Thus, Tesla’s implementation of thermal management systems illustrates practical strategies to extend battery life in electric vehicles.
Consumer Electronics
In the realm of consumer electronics, lithium-ion batteries are ubiquitous. Devices such as smartphones, laptops, and tablets depend heavily on these batteries. Key case studies include Apple and Samsung, two major players in the tech industry. Both companies focus on maximizing battery performance while ensuring user convenience.
For Apple, the iPhone series has integrated battery health management features. These features inform users about their battery’s capacity relative to when it was new. Users are encouraged to enable optimized battery charging, which helps in prolonging battery lifespan by learning charging habits.
Samsung, in contrast, faced challenges with the Galaxy Note 7 due to battery failures that resulted in overheating. This incident led to extensive investigations into safety and longevity, emphasizing the necessity of stringent quality control in battery manufacturing. Following this, they adopted more rigorous testing protocols and design improvements to avoid similar issues in future devices.
Battery lifespan in consumer electronics is often influenced by charging habits and battery management software. Users play a crucial role in extending the life of batteries through proper usage.
The End and Implications
The lifespan of lithium batteries is a critical aspect of their functionality and usability. Understanding this topic encompasses more than just the technical specifications of the batteries themselves; it extends to how they perform in real-world applications. The implications of battery lifespan affect a wide range of sectors, including electric vehicles, consumer electronics, and renewable energy storage solutions. By examining the factors influencing longevity, one can make informed decisions regarding usage, maintenance, and product design.
This article emphasizes several key benefits derived from an in-depth understanding of lithium battery lifespan. Improved decision-making empowers users to enhance the operational efficiency of devices. Awareness of charging practices and environmental impacts fosters sustainable usage, thereby extending battery life and minimizing waste. Furthermore, research into innovative solutions, like solid-state batteries, highlights future pathways for enhanced performance and safety.
Considering these aspects, it becomes clear that a nuanced understanding of lithium battery lifespan is necessary not only for engineers and researchers but also for average users. This knowledge underpins better battery management and informs purchasing decisions, aligning both economic and environmental considerations.
"The lifespan of a lithium battery dictates the overall effectiveness and sustainability of the technology it powers."
Thus, grappling with the implications of battery lifespan equips stakeholders in various industries with the tools needed for responsible use and innovation.
Summary of Key Points
- Lifespan Analysis: The longevity of lithium batteries is influenced by chemical composition, charging practices, and environmental factors.
- Degradation Mechanisms: Understanding solid electrolyte interface formation and lithium plating is crucial in improving battery performance.
- Improvement Strategies: Optimal charging techniques and the use of advanced battery management systems can significantly extend the life of batteries.
- Environmental Concerns: Consideration of recycling and disposal provides a sustainable framework for battery use, reflecting a broader responsibility towards environmental health.
Future Directions
Future trends in lithium battery technology hint at exciting developments. Solid-state batteries present a promising alternative, potentially offering greater safety and efficiency. On the other hand, advanced lithium-ion chemistries, like lithium-sulfur and lithium-air, are under exploration for their high energy density and sustainability.
Moreover, as electric vehicles and renewable energy systems gain traction, the need for longer-lasting and more efficient batteries will drive innovations. Research initiatives focusing on recycling methods and second life applications will also gain importance.