Neural Progenitor Cells: Role and Potential Unveiled
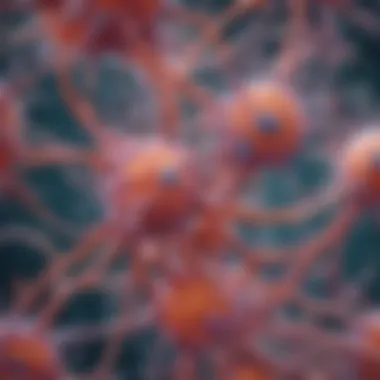
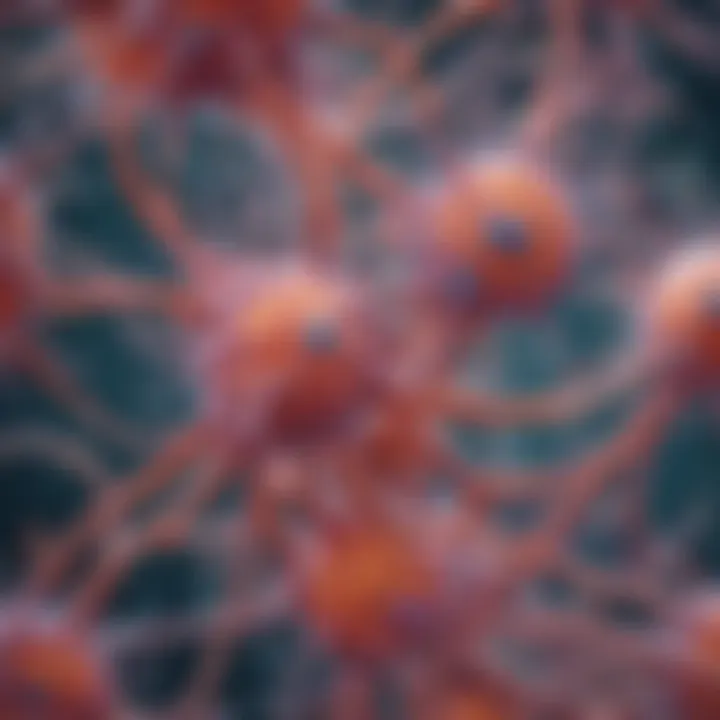
Intro
Neural progenitor cells represent a fundamental component in the landscape of neuroscience and regenerative medicine. Their unique properties allow them to contribute significantly to brain development and repair, making them a focal point of research in these fields. By understanding the mechanisms underpinning their biology, we can open new pathways to address various neurological disorders.
Research Overview
Key Findings
Research over the past two decades has highlighted several critical aspects of neural progenitor cells. Some notable findings include:
- Neurogenesis: Neural progenitor cells are pivotal in generating neurons and glial cells during embryonic development, significantly influencing the architecture of the nervous system.
- Differentiation Mechanisms: Various intrinsic and extrinsic factors regulate their differentiation into distinct cell types. The role of signaling pathways like Notch, Wnt, and Sonic Hedgehog has been heavily documented.
- Regenerative Potential: Studies indicate that these cells possess a remarkable capacity for self-renewal and differentiation, which holds promise for therapeutic approaches to brain injuries and neurodegenerative diseases.
Study Methodology
Investigations into neural progenitor cells utilize a range of methodologies. Key approaches include:
- In Vitro Studies: Researchers often culture these cells to examine their behavior and response to different stimuli, utilizing techniques such as immunocytochemistry and flow cytometry.
- In Vivo Models: Animal studies, particularly in rodents, help elucidate the role of neural progenitor cells in both development and disease contexts.
- Genomic Techniques: High-throughput sequencing and gene editing techniques (like CRISPR) have enhanced our understanding of the genetic factors influencing neural progenitor cell function.
Background and Context
Historical Background
The study of neural progenitor cells can be traced back to the late 20th century when the notion of adult neurogenesis began to emerge. Researchers initially believed that the adult brain was incapable of producing new neurons. However, the discovery of neural stem cells in the adult hippocampus challenged this view and paved the way for further investigations into neural progenitor cells.
Current Trends in the Field
Currently, the field is witnessing significant advancements focusing on:
- Therapeutic Applications: Researchers are exploring the use of neural progenitor cells in treating conditions such as Parkinson's disease and spinal cord injuries.
- Cellular Mechanisms: There is a growing interest in understanding cellular signaling pathways and environmental conditions affecting the differentiation and survival of these cells.
- Ethical Implications: As with any advancing field, ethical discussions surrounding the use of stem cells in research and therapy are increasingly prominent.
This exploration of neural progenitor cells not only highlights their importance in neurobiology but also illuminates their potential to become a cornerstone in developing innovative therapies for neurological disorders.
Intro to Neural Progenitor Cells
Understanding neural progenitor cells is essential for grasping their role in brain development and repair. These cells hold promise for treating various neurological conditions, making them a focal point in regenerative medicine. By discussing the definition and historical context of these cells, we shall illustrate their significance in scientific research and potential applications.
Definition
Neural progenitor cells, or NPCs, are a type of stem cell that can differentiate into various types of neural cells. This classification includes neurons, astrocytes, and oligodendrocytes. NPCs are characterized by their ability to undergo extensive self-renewal and have a defined capacity for differentiation, which sets them apart from fully developed neural cells. They reside mainly in the developing brain, but subsets have been identified in adult neural tissues as well.
NPCs are crucial during both embryonic development and adult brain function. In embryonic stages, they contribute to generating the neural cell population necessary for forming a functional central nervous system. In adulthood, they play a role in neurogenesis, particularly in areas like the hippocampus, influencing learning and memory.
Historical Context
The history of neural progenitor cells has evolved considerably over the past few decades. Early research in the 20th century primarily focused on fully differentiated neural cells. However, the discovery of neural stem cells in the 1990s marked a paradigm shift. In particular, studies revealed that NPCs are not only present in the developing brain but also persist into adulthood.
The realization that these progenitor cells could be harnessed for therapeutic purposes led to extensive investigations. In the early 2000s, scientists identified specific markers indicative of NPCs, such as nestin and Musashi. This identification provided a way to isolate and study these cells in vitro.
Research continued to expand, revealing the unique properties and potentials of neural progenitor cells. Today, these cells are at the forefront of regenerative medicine research, exploring avenues for treatment of conditions like Alzheimer’s, Parkinson's disease, and spinal cord injuries.
"Neural progenitor cells are not only foundational to neurodevelopment but also present an avenue for novel therapies in neurodegenerative conditions."
In summary, examining neural progenitor cells and understanding their definition and historical background is vital. It establishes a foundation for comprehending their role in neurogenesis and their therapeutic potential, which will be further elaborated upon in upcoming sections.
Biological Characteristics
Understanding the biological characteristics of neural progenitor cells is essential for grasping their role in neurogenesis and potential applications in therapeutic settings. These characteristics reveal the underlying mechanisms of how these cells function, proliferate, and differentiate into various cell types. Knowledge of their biological traits also helps in developing strategies to manipulate these cells for clinical use, particularly in regenerative medicine and for treating neurological disorders.
Cellular Structure
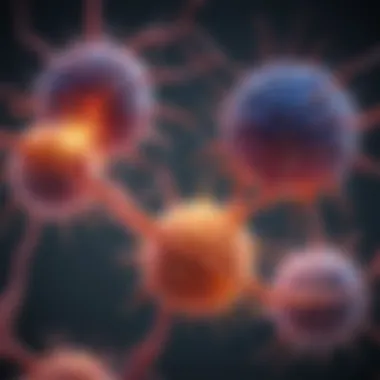
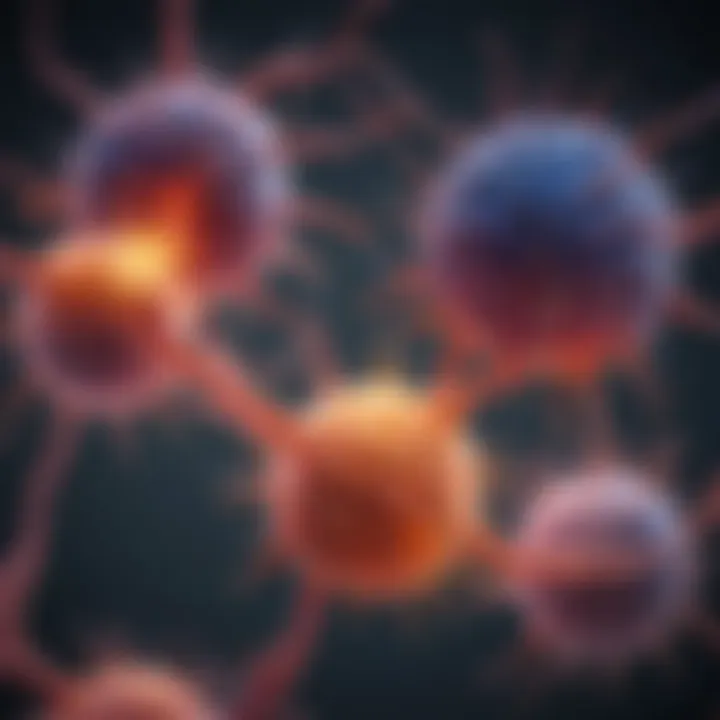
Neural progenitor cells exhibit unique features in their cellular structure that distinguish them from other cell types. They typically have a round cell body, with short and branched processes known as neurites, which play a role in cell communication. This structure is crucial for their ability to respond to environmental signals and to proliferate effectively.
The morphology can vary depending on the developmental stage and the specific type of progenitor cell. For instance, cells in the early stages of development tend to be smaller and exhibit less complexity in their structural features. On the other hand, more mature neural progenitor cells take on a more elaborate architecture, reflecting their readiness to differentiate.
In addition to their shape, the cellular structure of neural progenitor cells includes organelles that support their unique functions. For example, a well-developed endoplasmic reticulum and Golgi apparatus indicate high levels of protein synthesis, which is necessary for creating new cellular components as the cells divide and differentiate.
Genetic Markers
Genetic markers are critical for identifying and studying neural progenitor cells. These markers are specific genes or proteins that are upregulated in progenitor cells compared to other cell types. Understanding these markers allows researchers to track the development and fate of progenitor cells in various contexts.
Common genetic markers include Nestin, which is often associated with neural progenitors, and Sox2, a transcription factor crucial for maintaining stemness. Additionally, CD133 is another marker frequently used to isolate and characterize these cells. These markers assist in the identification of progenitor cells in tissues or cultures, thus enabling advancements in both research and therapeutic applications.
Proliferation Mechanisms
The mechanisms by which neural progenitor cells proliferate are complex and tightly regulated. Several signaling pathways govern this process, ensuring that cells maintain a balance between proliferation and differentiation. Understanding these mechanisms is essential for manipulating progenitor cells for scientific and medical purposes.
Key pathways include the Notch signaling pathway, which plays a fundamental role in regulating the balance between neural progenitor proliferation and differentiation. Activation of Notch can maintain progenitor cells in an undifferentiated state. Conversely, signaling through the Wnt pathway has been implicated in promoting proliferation, linking extracellular cues to intracellular responses.
This regulation of proliferation offers potential for exploiting neural progenitor cells in research and therapy, especially in the context of injuries or degenerative diseases. By understanding these mechanisms, scientists can better strategize how to harness the regenerative potential of neural progenitor cells.
The Role in Neurogenesis
Understanding the role of neural progenitor cells in neurogenesis is critical for both scientific research and clinical applications. Neurogenesis refers to the process of generating new neurons, a phenomenon primarily occurring during early development but also continuing to some extent in adulthood. Neural progenitor cells are foundational in this process, acting as precursors that can develop into various types of neural cells including neurons and glial cells. The study of neural progenitor cells opens up avenues such as regenerative medicine, where there is potential for repairing or replacing damaged neural tissues in conditions like Alzheimer’s disease or spinal cord injuries.
Developmental Pathways
Developmental pathways in neural progenitor cells are essential for understanding how these cells differentiate into mature neural cells. These pathways are regulated by a complex interplay of genetic and molecular mechanisms. It is observed that specific transcription factors and signaling cues dictate the fate of neural progenitor cells. For instance, the Notch signaling pathway maintains progenitor cell properties, while the Wnt pathway influences the transition towards neuronal differentiation. The balance between proliferation and differentiation must be finely tuned to ensure proper brain development. Misregulation can lead to various neurodevelopmental disorders, highlighting the significance of these pathways in neurogenesis.
Differentiation into Neurons and Glia
Differentiation into neurons and glia is the outcome of the complex interactions that neural progenitor cells undergo during the neurogenesis process. After receiving appropriate signals, neural progenitor cells may become either neurons, which are responsible for signal transmission, or glial cells, which provide support and protection for neurons. This differentiation is not merely a linear process but involves multiple intermediate stages. Different types of neurons and glial cells are generated based on the timing and environmental context of the differentiation signals. Research illustrates that the specific conditions and factors influencing this differentiation are crucial for producing the right types and numbers of neural cells.
Signaling Pathways
Signaling pathways play a key role in the neurogenic process facilitated by neural progenitor cells. Various pathways including Sonic Hedgehog (Shh), Bone Morphogenetic Proteins (BMPs), and fibroblast growth factors (FGFs) modulate the behavior of these progenitor cells.
Signaling pathways provide the critical information necessary to guide neural progenitor cells towards specific differentiation outcomes, ensuring the proper development of the nervous system.
These pathways often communicate with each other in a cascade, leading neural progenitor cells to make fate decisions that shape neural circuitry. Understanding these pathways not only informs basic neuroscience research but also has implications for developing therapies targeting neurological conditions. As researchers delve deeper into these signaling mechanisms, potential therapeutic strategies continue to emerge, focusing on harnessing the power of neural progenitor cells to restore function in damaged neural networks.
Isolation and Culture Techniques
Isolation and culture techniques are crucial for the study and application of neural progenitor cells. Understanding how to effectively isolate these cells from their original environment and maintain them in vitro allows researchers to explore their properties and potential applications in medicine and biology. This section covers the various approaches used to obtain and culture neural progenitor cells, highlighting the significance of each method.
Embryonic Origin
Neural progenitor cells can be isolated from embryonic tissue, particularly from developing brain regions. The embryonic origin of these cells is essential since they exhibit higher proliferation potential and greater plasticity compared to adult neural progenitor cells. In the embryo, the neural tube provides a rich source of progenitor cells. Techniques such as enzymatic digestion, microdissection, and the use of specific growth factors help isolate these cells. These methods focus on obtaining specific subpopulations of progenitor cells that can lead to more effective in vitro cultures.
The isolation process is significant because it influences the characteristics of the cultured cells. For instance, the source determines the differentiation capacity of progenitor cells. When working with embryonic neural progenitor cells, it is possible to utilize them in various research contexts, including studying developmental processes and modeling neural disorders.
Adult Neural Progenitor Cells
Adult neural progenitor cells, found in specific niches like the hippocampus and subventricular zone, are vital for maintaining the adult brain's health and function. The isolation of these cells presents unique challenges. Their numbers are limited compared to embryonic progenitors. Techniques include tissue dissociation followed by filtration to remove debris and non-cellular material.
These cells typically exhibit more restricted differentiation potential, primarily giving rise to neurons and glial cells. Nonetheless, adult neural progenitor cells have been extensively studied for their role in brain repair and neurogenesis. The ability to culture these cells offers insights into age-related changes and potential therapeutic strategies for neurodegenerative diseases.
In Vitro Culturing Methods
Culturing neural progenitor cells in vitro involves maintaining the right conditions for their growth and differentiation. This requires a careful selection of culture media, which often contains supplements like fibroblast growth factor-2 or epidermal growth factor. Such factors promote cell proliferation and prevent differentiation during the early culture phases.
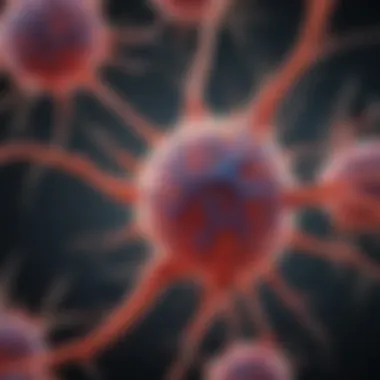
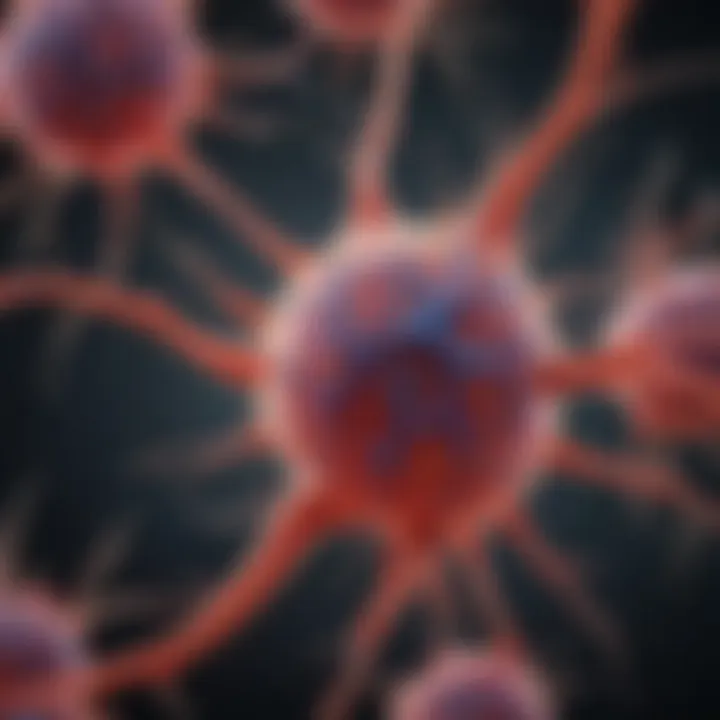
Here are key aspects of in vitro culturing methods:
- Attachment: Choosing appropriate substrates that support cell adhesion is crucial. Coating dishes with poly-L-lysine or laminin enhances cell attachment and growth.
- Environment: Maintaining a stable environment, including temperature and CO2 levels, is essential for ensuring the health of neural progenitor cultures.
- Passaging: Regular passaging helps maintain a healthy population density and overall cell health. This involves detaching cells using enzymatic methods and transferring them to new culture vessels.
"The proper isolation and culture of neural progenitor cells not only allow researchers to study fundamental biological processes but also enable advancements in regenerative medicine."
In summary, isolation and culture techniques are foundational elements in the study of neural progenitor cells. Whether derived from embryonic or adult sources, these methods provide insights into cell behavior, differentiation, and potential therapeutic uses. Ongoing improvements in these techniques will continue to enhance our understanding and applications of neural progenitor cells.
Current Research Trends
Research on neural progenitor cells is expanding rapidly. This section outlines key trends that significantly impact understanding their roles and potential applications. Two areas of focus are studies involving neural progenitor cells in disease models and advancements in gene editing. These trends are crucial because they explore innovative ways to utilize these versatile cells in medical research and treatment.
Neural Progenitor Cells in Disease Models
Neural progenitor cells are pivotal in studying various neurological disorders. These cells help in mimicking disease conditions in vitro, allowing researchers to grasp the disease mechanisms better. For instance, models using Alzheimer’s disease or Parkinson's disease have benefited from the introduction of these cells. They can replicate the disease progression effectively, which is essential for testing therapeutic strategies.
In addition to testing drugs, these models illuminate the cellular changes that occur in disease states. Understanding how neural progenitor cells respond to pathological stimuli can lead to novel interventions that restore normal function. For instance, research into how these cells react to amyloid-beta, a hallmark of Alzheimer’s, is vital. Identifying specific signaling pathways involved may offer targets for new therapies.
Gene Editing Applications
Gene editing tools, such as CRISPR-Cas9, are making significant inroads in research involving neural progenitor cells. These advancements allow for precise modifications of the genome, facilitating the exploration of gene functions associated with neurodevelopment and neurodegeneration. By manipulating specific genes, researchers can observe how changes affect cell behavior and differentiation.
Moreover, gene editing can enhance the regenerative capacities of neural progenitor cells. For example, editing genes linked to neuroplasticity could improve recovery outcomes in neurotrauma or stroke. This area holds promise for personalized medicine, where tailored treatments are designed based on an individual’s genetic profile.
The integration of gene editing with neural progenitor cell research is not just theoretical. It presents feasible pathways toward transforming treatments for neurological disorders.
In summary, current research trends highlight the relevance of neural progenitor cells in disease modeling and gene editing. Both areas carry potential to propel forward understanding and treatment of complex neurological conditions, enhancing the scope of regenerative medicine.
Therapeutic Potential
The study of neural progenitor cells (NPCs) presents significant therapeutic potential. As advancements in regenerative medicine continue, understanding how NPCs can be utilized for therapeutic purposes is essential. Their unique ability to differentiate into various cell types offers hopeful applications in treating neurological disorders that are currently difficult to manage effectively.
Regenerative Medicine Approaches
Regenerative medicine involves techniques that aim to restore the structure and function of damaged tissues or organs. Neural progenitor cells are central to these approaches, as they can potentially replace lost or dysfunctional neurons. They contribute to neurogenesis, the process of generating new neurons, particularly in areas of the brain that suffer from injury or disease.
Some approaches in regenerative medicine utilizing NPCs include:
- Cell Replacement Therapy: This involves transplanting NPCs into affected areas to promote recovery and function.
- Biomaterials: Coating NPCs with bioengineered materials can enhance their survival and integration within damaged tissues.
- Gene Therapy: This approach employs NPCs as vectors to deliver therapeutic genes to diseased tissues, potentially correcting underlying genetic disorders.
These strategies hold promise for conditions like Alzheimer's, Parkinson's, and spinal cord injuries.
Transplantation Studies
Transplantation studies have been critical in exploring the therapeutic potential of NPCs. These studies often focus on animal models to assess the effectiveness of NPC transplants in restoring function. For instance, researchers have successfully demonstrated that transplanted NPCs can integrate into neural circuits and promote functional recovery after brain injuries. Such results are particularly relevant in the context of neurodegenerative diseases.
Recent advancements have included:
- Autologous Transplants: Utilizing a patient's own NPCs reduces rejection risks and enhances compatibility.
- Long-term Survival Studies: Investigating NPC behavior post-transplantation informs researchers about their survival, integration, and functionality over time.
A current focus is on optimizing conditions for transplant success, including the microenvironment and timing of transplantation.
Challenges and Limitations
Despite the promising nature of NPCs in therapeutic applications, several challenges exist. These challenges include:
- Ethical Concerns: The methods to derive NPCs, particularly from embryonic sources, raise ethical issues that affect public and regulatory acceptance.
- Tumorigenesis: There is a risk that transplanted NPCs could proliferate uncontrollably, leading to tumor formation, which poses significant health risks.
- Integration: Successful integration into native brain tissue remains an issue, as transplanted cells must connect correctly to existing neural circuits to function properly.
In conclusion, while the therapeutic potential of neural progenitor cells is vast, continued research is necessary to address these hurdles and pave the way for effective, safe treatments.
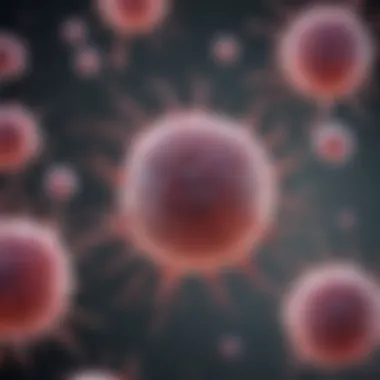
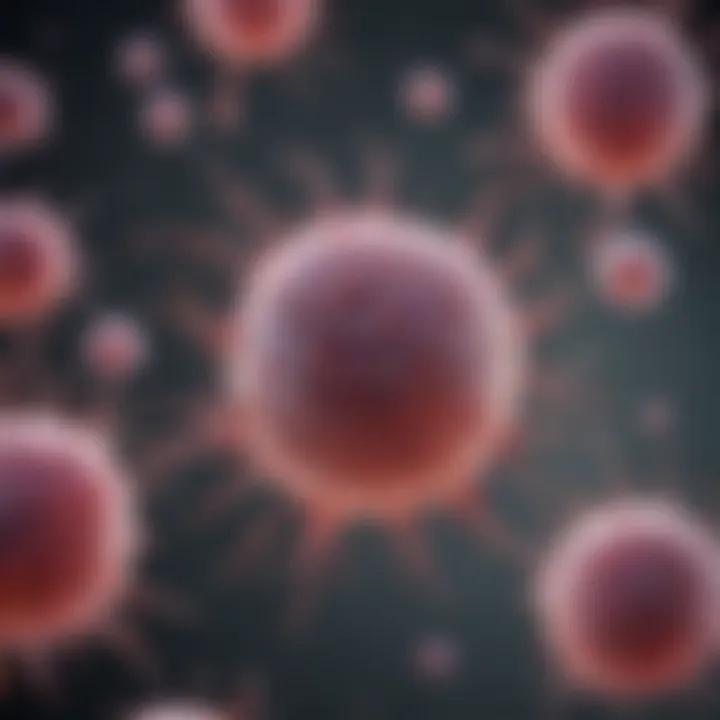
Ethical Considerations
In the realm of scientific research on neural progenitor cells, ethical considerations play a pivotal role. This topic signifies the need to address the moral implications arising from the sourcing, utilization, and applications of these cells. Understanding ethical standards is essential to advance research without compromising the dignity and rights of living beings. Scientists and institutions are compelled to consider the potential consequences of their work on society as well as the environment. Moreover, navigating this field requires a balance between innovation and responsible conduct.
Source of Cells
The source of neural progenitor cells is a pressing ethical concern. Cells can be derived from various origins, primarily embryonic tissue, adult tissues, and induced pluripotent stem cells. The use of embryonic progenitor cells often draws significant scrutiny because acquiring them involves the destruction of embryos. Such practices raise questions about the moral status of human embryos and provoke debate among different cultural and religious groups.
Conversely, adult neural progenitor cells, sourced from the brain or other tissues, present an alternative. Their ethical standing is typically less contentious, as these cells come from non-embryonic origins, thus avoiding the complexities related to embryonic cell use. Furthermore, advances in technology have enabled the generation of induced pluripotent stem cells. These cells, reprogrammed from somatic cells, inherit the potential of embryonic cells, yet their procurement is less ethically charged.
The choice of source impacts not only the scientific agenda but also public perception of research efforts. Maintaining transparency about cell sources is key to fostering trust and public engagement in research initiatives.
Regulations in Research
Research involving neural progenitor cells is governed by a complex framework of regulations. These regulations ensure that ethical standards are upheld throughout various stages of research, from cell sourcing to clinical applications. In different countries, agencies like the National Institutes of Health (NIH) in the USA and the European Medicines Agency (EMA) establish guidelines that dictate permissible practices concerning neural progenitor cells.
Specific regulations outline the requirements for informed consent, particularly in human-derived cells, ensuring that donors are fully aware of how their cells will be used. Furthermore, monitoring is essential to prevent exploitation and to uphold the rights of participants.
Moreover, guidelines address safety, ethical review processes, and animal welfare in preclinical studies. The aim is to balance scientific inquiry with the respect for living entities involved in research, thus fostering ethical advancements in the field of regenerative medicine.
Informed consent and regulatory oversight are foundational to maintaining ethical standards in research on neural progenitor cells.
Understanding these regulations not only protects ethical practices but also enhances the credibility of scientific endeavors. As the field evolves, continuous dialogue about ethical considerations will be vital in guiding responsible research and its applications for societal benefit.
Future Directions
The investigation surrounding neural progenitor cells (NPCs) is crucial as it opens up potential avenues for therapies aimed at neurological disorders and injuries. This segment highlights the significance of forward-looking research in this field, focusing on innovative methodologies and their long-term implications. Understanding the future directions of NPC research not only enriches the academic discourse but also aligns scientific inquiry with practical applications.
Innovations in Research Methodologies
Recent advancements in research methodologies for studying NPCs are transforming our approach to neurogenesis. Notable methods include single-cell RNA sequencing and CRISPR-Cas9 gene editing, which allow researchers to dissect the complexities of NPC differentiation and function at an unprecedented resolution. These methods enable the identification of individual cell types and their specific roles in brain development and repair.
Moreover, the development of three-dimensional culture systems further enhances the study of NPC behavior in a more naturalistic environment relative to conventional two-dimensional cultures. This evolution also provides insights into the spatial dynamics of cellular interactions that are instrumental during neurogenesis.
"Innovative research methodologies help uncover the hidden complexities of neural progenitor cells, facilitating novel therapeutic strategies."
The incorporation of machine learning into data analysis is another significant advancement. By analyzing large datasets, machine learning algorithms can identify patterns and predict outcomes related to neurogenesis, thereby accelerating the discovery of effective treatments.
Long-term Implications
The long-term implications of advancements in NPC research are vast and multifaceted. The ability to manipulate these cells holds promise for developing regenerative therapies that could transform conditions such as Alzheimer’s disease, multiple sclerosis, and spinal cord injuries. Understanding NPCs provides critical insights into brain development and aging, which has ramifications not just for medicine but for how society approaches brain health.
Furthermore, as researchers uncover more about the specificity of NPC functions, it may pave the way for targeted therapies that minimize side effects associated with broader treatments. Insights from NPC studies could also have a ripple effect in other biomedical fields, especially in tissue engineering and organoid development, enhancing the overall landscape of biomedical research.
In summary, as we advance into new methodologies and approaches, the future of neural progenitor cell research appears promising with the potential to redefine treatment paradigms. Addressing these future directions can lead to groundbreaking discoveries that significantly enhance our understanding of the nervous system and its regeneration.
Culmination
The conclusion of this article serves as a pivotal moment, emphasizing the significance of neural progenitor cells in contemporary scientific research and their future prospects. These cells are not merely a point of interest within neurobiology; they represent a frontier in understanding the complexities of the nervous system. Neural progenitor cells hold incredible potential for regenerative medicine, particularly in addressing neurological disorders where current treatments often fall short.
Summary of Findings
Throughout this article, key elements about neural progenitor cells have been explored in depth.
- Biological Role: These cells are crucial for neurogenesis and maintaining neural homeostasis.
- Differentiation: Their ability to differentiate into neurons and glial cells illustrates a fundamental aspect of brain development.
- Therapeutic Applications: Insights into their application in regenerative medicine promote hope for treating conditions like Alzheimer’s and Parkinson’s disease.
Emphasis has been placed on the isolation and culture techniques, which are essential for maximizing their utility in research and therapy. Studies focusing on disease models demonstrate how neural progenitor cells can be leveraged to develop innovative treatments.
The Importance of Continued Research
As we conclude, it is clear that the journey into understanding neural progenitor cells is far from over. Continued research is necessary for several reasons:
- Unraveling Mechanisms: Understanding the underlying mechanisms that govern cell behavior and differentiation can unlock new therapeutic strategies.
- Ethical Considerations: With advancements in gene editing and cell sourcing, ethical frameworks must evolve simultaneously to address emerging dilemmas.
- Application in Treatment: Focusing on clinical trials will ensure that findings translate effectively into viable therapies, benefiting patients with debilitating neurological disorders.
In summary, the exploration of neural progenitor cells is not just an academic exercise; it is a crucial step toward future medical breakthroughs. Ongoing investment in this area will yield significant benefits for both the scientific community and society as a whole.