Exploring AMS Soil Samplers: Types and Techniques
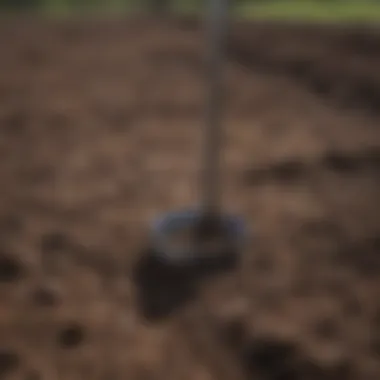
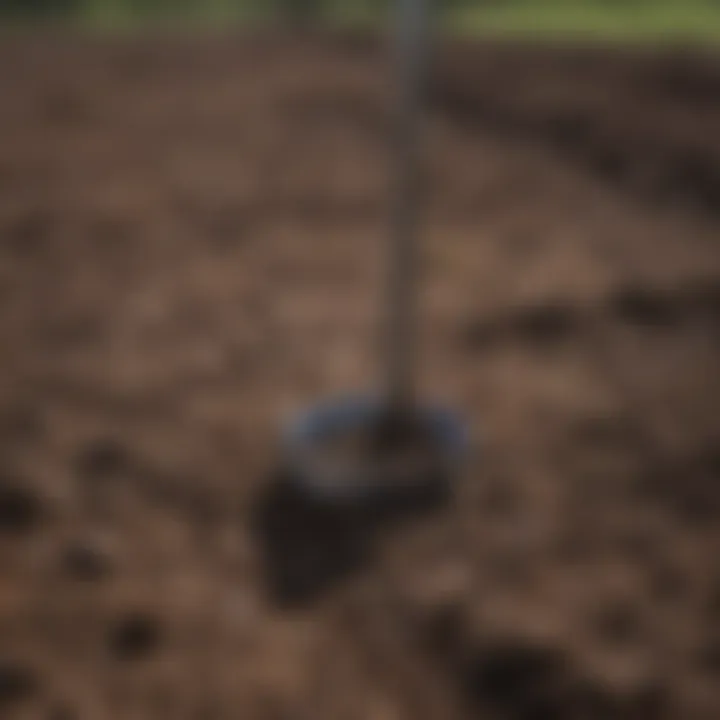
Intro
AMS soil samplers play a critical role in environmental science. These tools are specifically engineered to collect soil samples, enabling various analyses necessary for ecological research, agricultural assessment, and contaminant evaluation. The significance of these samplers extends across disciplines, creating pathways for better understanding of soil health and its impact on the environment. The narrative unfolds several aspects of AMS soil samplers: their types, pertinent techniques, and best practices for effective use.
Researchers, educators, and professionals in the field of environmental science will find this overview enriches their knowledge base. The aim is to facilitate improved methodologies in soil sampling, ultimately contributing to enhanced data quality and research outcomes. This groundwork is essential for comprehending the intricate dynamics within soil ecosystems.
Research Overview
Key Findings
Research consistently illustrates that soil health is directly linked to overall ecosystem function. AMS soil samplers provide a means to accurately collect soil profiles, allowing for comprehensive analysis. Key findings include:
- Improved soil health assessment through precise sampling
- Enhanced data reliability in ecological studies
- Better monitoring of contaminant spread
Study Methodology
The methods employed in soil sampling vary depending on the objectives of the study. Utilizing AMS soil samplers, researchers often follow these steps:
- Site Selection: Identifying representative areas for sampling based on goals.
- Sampler Preparation: Selecting the appropriate AMS sampler model suited for the soil type and sampling depth.
- Sample Collection: Extracting the soil carefully to avoid contamination.
- Sample Processing: Analyzing samples promptly to preserve data integrity.
The methodology emphasizes meticulous attention to detail to ensure reliable results, underscoring the importance of standard practices in soil sampling.
Background and Context
Historical Background
Soil sampling has a long-standing history, rooted in agricultural practices. The evolution of tools has significantly advanced, with AMS being at the forefront of technology. Early methods were limited and often inconsistent. However, as environmental awareness grew, the necessity for more robust tools emerged.
Current Trends in the Field
Recent trends in soil sampling reflect an increasing integration of technology. Innovations in AMS samplers now feature improvements in durability and precision, catering to a broader spectrum of environmental research. Moreover, there is a growing focus on sustainability, with practices aimed at reducing ecological impact during sampling.
Understanding the evolution and current trends in soil sampling is critical. It helps researchers adapt to ongoing changes in environmental science and contributes to more effective methodologies.
Prelude to AMS Soil Samplers
AMS soil samplers serve a critical role in environmental science, providing the means to collect soil samples efficiently for analysis. The understanding and use of these tools are fundamentally important, and their methods can greatly influence the quality and accuracy of the data gathered. In this section, we will dissect the definition and purpose of AMS soil samplers, as well as their significance in research settings.
Definition and Purpose
AMS soil samplers are specialized devices designed to extract soil samples from various depths and conditions. These tools are engineered to minimize disturbance to the surrounding soil structure during sampling. The primary function of these samplers is to facilitate the collection of undisturbed soil cores, which are essential for analyzing various soil properties such as pH, moisture content, and nutrient levels.
The purpose of using AMS soil samplers extends beyond mere extraction of soil. It also includes ensuring that the samples remain representative of the larger soil body. This is vital when conducting studies that rely on accuracy, such as agricultural assessments or environmental monitoring. The user can better understand soil conditions and variability by obtaining samples that reflect the actual state of the soil in its natural environment.
Importance in Research
In research, the importance of AMS soil samplers cannot be overstated. They enable scientists and practitioners to conduct detailed analyses that inform agricultural practices, environmental policies, and land-use planning. Effective soil sampling is foundational in understanding soil health and fertility. It influences decisions about crop rotation, fertilization and other key agricultural practices.
AMS soil samplers also play a significant role in environmental monitoring and assessment. They help researchers identify contamination levels and understand the distribution of pollutants in soils. Not only does this information support the remediation of contaminated sites, but it also aids in the maintenance of ecological health.
"Inadequate soil sampling can lead to misleading results, affecting not only research projects but also practical applications in agriculture and environmental management."
The efficiency and accuracy of these samplers can impact the overall outcomes of scientific research. By using quality AMS soil samplers, researchers increase the reliability of their findings, leading to informed conclusions and sustainable practices. Therefore, understanding what AMS soil samplers are and their significance in research is crucial for anyone involved in environmental sciences.
Types of AMS Soil Samplers
Understanding the different types of AMS soil samplers is crucial for researchers and environmental professionals. Each type of sampler has unique properties that make it suitable for specific soil sampling situations. Knowing these can enhance the quality of data collected while considering the project's objectives, soil type, and sampling depth.
Core Samplers
Core samplers are specifically designed to extract cylindrical soil cores from the ground. They are vital for providing undisturbed samples that accurately represent the soil layers. The primary use of core samplers is in agricultural assessments and environmental studies.
When using core samplers, the sample integrity is often high because the sampler minimizes disturbances to the soil structure. It allows for detailed analysis of soil composition, moisture content, and others. These attributes make core samplers fundamental in researching soil health and fertility.
The efficiency of core samplers is enhanced by using various diameters to suit different soil types. For example, a smaller diameter core sampler may be chosen for compacted soils where a larger sampler could face resistance. Hence, the selection of an appropriate size can significantly affect the results obtained.
Auger Samplers
Auger samplers are another popular type of soil sampler. They function by drilling into the ground to collect soil samples. The significant advantage of auger samplers is their ability to reach considerable depths, making them suitable for geological or archaeological studies.
The design of auger samplers can vary significantly. Hand-held models are user-friendly and often employed for shallow sampling, whereas powered versions can perform at deeper levels or in harder soil. It is important to choose the right kind based on the context of the sampling.
Furthermore, auger samplers can often collect larger volumes of soil compared to core samplers. This is beneficial whenever bulk analysis is required, such as when analyzing less homogeneous soil profiles.
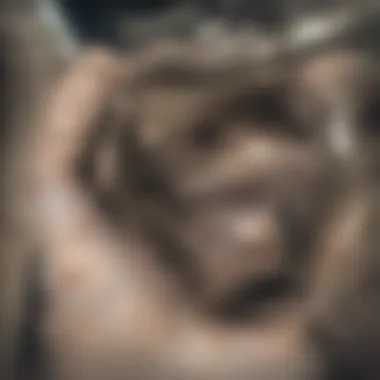
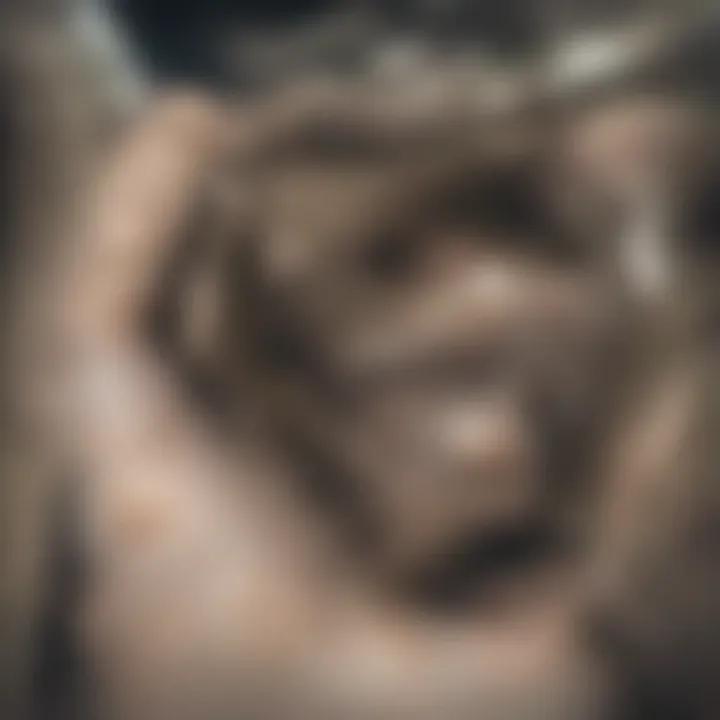
Screw-Type Samplers
Screw-type samplers provide a distinct method for soil sampling. Their spiral design allows them to penetrate the soil easily, making them suitable for various soil textures. This type of sampler can operate effectively in sandy or clay-rich soils, which can challenge other sampling methods.
An important advantage of screw-type samplers is their efficiency in collecting samples from difficult terrains. They are also less labor-intensive, reducing the time spent on each sampling task.
The screw mechanism allows for simple retrieval of samples. This feature helps minimize disturbance to the surrounding soil environment, supporting higher sample integrity, similar to core samplers. As a caution, however, careful handling is necessary to ensure samples are not compromised during extraction.
Components of AMS Soil Samplers
Understanding the components of AMS soil samplers is crucial for both effective usage and accurate results in soil sampling activities. Each part of these samplers plays a distinct role that enhances the overall performance and reliability of soil analysis. Key components include the sampling tubes, handles and extensions, and collection containers. Each of these elements contributes to the efficiency and convenience of soil sampling.
Sampling Tubes
Sampling tubes are the primary component of AMS soil samplers. They are designed to penetrate the soil and retrieve a core sample for analysis. The design and material of the sampling tubes are vital. They must be sturdy enough to endure various soil conditions while also being light enough for easy handling.
The inner diameter of the sampling tube impacts the volume of soil that can be collected. A wider tube collects more soil, which can provide a more representative sample. However, larger tubes can also be heavier and more difficult to maneuver in the field. It's essential to balance these factors based on the specific requirements of the research being conducted.
In addition to diameter, the length of the sampling tubes should be considered. Longer tubes allow researchers to obtain samples from greater depths, which is necessary for understanding soil stratification and composition. This depth capability can be crucial for studies related to contaminant transport or plant root systems.
Handles and Extensions
The handles of AMS soil samplers are another key component that directly affects user experience. A well-designed handle should provide comfort and a secure grip, allowing researchers to apply adequate force without discomfort. Ergonomic designs can reduce user fatigue and improve overall sampling efficiency.
Extensions may be useful when accessing deeper soil layers. These extensions connect to the handle and allow for greater reach without compromising stability. It is important to ensure that extensions are compatible with the sampling tube and handle, as improper fittings can lead to accidents or improper sampling.
When considering field conditions, the type of handle and extension must suit the terrain. For example, a sampler equipped with a robust handle will perform better in rocky soils or difficult terrains compared to a standard model.
Collection Containers
Collection containers serve an essential function in storing the soil samples after they are retrieved. The choice of container can influence sample integrity and subsequent analysis. Containers need to be made of materials that do not react chemically with the soil, ensuring that the sample remains unaltered for accurate testing results.
Specific sizes of containers may be chosen based on the volume of the collected sample. Larger samples may require more substantial containers while smaller volumes can be stored in compact vessels. Ensuring that the container is also sealable is important to prevent contamination during transportation.
In summary, each component of AMS soil samplers is intricately linked and contributes towards the effective collection of soil samples. Understanding the significance of sampling tubes, handles and extensions, as well as collection containers, equips researchers with the knowledge required to select the appropriate tools for their studies. By optimizing these components, the reliability and accuracy of soil sampling can be significantly enhanced.
Techniques for Effective Soil Sampling
Soil sampling is a critical process that determines the quality and integrity of the data collected for environmental studies. Utilizing adequate techniques can enhance the reliability and applicability of soil analyses. An understanding of specific methodologies—such as selecting the appropriate location, considering depth and volume, and ensuring the retention of sample integrity—can significantly influence outcomes.
Choosing the Right Location
Selecting the right location for soil sampling is paramount. The area chosen must represent the site conditions accurately to yield meaningful data. Factors such as topography, drainage patterns, and current or historical land use play a significant role. Remote areas may display variations that may not reflect the broader landscape.
When identifying a sampling site, researchers should consider the following:
- Accessibility: Ensure that the location is reachable for regular monitoring.
- Homogeneity: Select areas with similar soil types and characteristics to minimize variability in samples.
- External Influences: Avoid locations influenced by nearby constructions, industries, or excessive plant growth which might skew analysis.
The most valid conclusions stem from samples that truly represent the conditions of the broader area under study.
Depth and Volume Considerations
Soil properties can change substantially with depth, making it essential to define both the depth and volume of the sample collected. Different applications may require samples from various soil horizons. For instance, nutrient levels often vary higher in the topsoil compared to deeper layers.
Key aspects to consider include:
- Sampling Depth: Depending on the goal of the analysis, shallow samples might suffice for certain assessments, while deeper profiles are required for other analyses.
- Volume of Sample: Collecting larger volumes often yields more reliable results as it minimizes the chance of errors.
- Layer Identification: Distinguishing between layers can reveal critical data regarding soil formation and contamination.
Assessing depth and volume is not just about meeting technical standards but about ensuring that conclusions drawn from the data are well-founded.
Sample Integrity Retention
Sample integrity is crucial for achieving accurate and reliable results. Factors influencing integrity include contamination, soil disruption, and environmental conditions during collection and transport. Adopting best practices to preserve sample integrity can prevent compromised analytical results.
Considerations for maintaining sample integrity include:
- Prompt Handling: Process samples quickly after collection to minimize alterations.
- Proper Containers: Use appropriate, contamination-free containers that suit the specific sample type.
- Temperature Control: Maintain a stable temperature to avoid changes in sample properties during transit.
"Correct sample preservation is essential; even minor mistakes can lead to significant misinterpretations of research conclusions."
Adhering to best practices for sample integrity ensures that soil data remains valid and trustworthy, ultimately supporting more informed decision-making in both environmental research and agricultural practices.
Comparative Analysis of Soil Sampling Methods
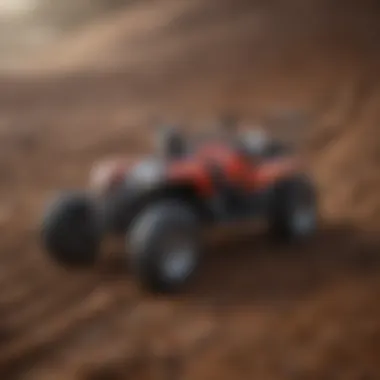
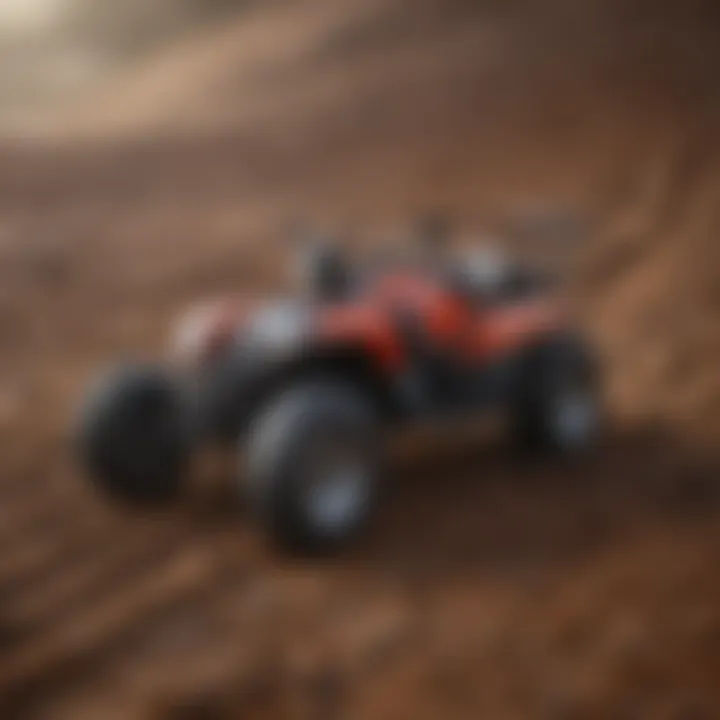
The exploration and understanding of soil properties are crucial for a range of applications, including agriculture, environmental monitoring, and scientific research. Therefore, a comparative analysis of soil sampling methods becomes imperative. This section sheds light on the different approaches available for soil sampling. It addresses the importance of choosing the right method, as this can significantly influence the quality and reliability of data collected.
Manual vs. Automated Sampling
Manual soil sampling is a traditional method that has been used for decades. This approach requires an operator to physically collect soil samples using hand tools or basic equipment. The advantages of manual sampling include flexibility and control over sample locations. Researchers can select specific areas based on visual assessment or prior knowledge. However, manual sampling can be labor-intensive and time-consuming, especially in large-scale studies.
Automated sampling, on the other hand, employs machinery or robotic systems to perform soil collection. This method offers high efficiency and consistency. Automated systems can quickly sample multiple locations, reducing the time needed to complete a study. Additionally, these systems can minimize human error, ensuring uniformity in sample collection. However, automated methods often come with higher upfront costs and may require technical expertise for operation and maintenance.
In summary, the choice between manual and automated sampling methods depends on various factors, including project scale, budget, and required precision. Each has its merits and limitations, and understanding these can lead to more effective soil collection strategies.
Cost-Benefit Evaluation
When considering soil sampling methods, a cost-benefit analysis is essential. This evaluation compares the financial aspects of different sampling techniques against the expected outcomes and data quality.
Cost Factors:
- Initial investment: Automated systems typically require a significant initial investment in equipment.
- Operational costs: Manual sampling may incur lower machinery costs but requires more labor and time.
- Maintenance: Automated equipment needs regular maintenance, adding to ongoing costs.
Benefit Considerations:
- Data quality: Higher quality data can justify the higher costs associated with advanced methodologies.
- Time efficiency: Automated systems can deliver quicker results; thus, their initial cost may be recouped through time savings.
- Labor considerations: If labor costs are high in a region, automation could prove beneficial in the long run.
An effective cost-benefit evaluation requires a thorough understanding of project goals. Selecting the most appropriate method isn't merely about cost; it also involves weighing the impact of the method on research outcomes and efficiency.
“Choosing the right soil sampling method can lead to improved data integrity, ultimately benefiting environmental assessments.”
Applications of AMS Soil Samplers
AMS soil samplers play a crucial role in various scientific assessments. Their adaptability allows for usage across multiple fields, including agriculture, environment monitoring, and contaminant detection. Understanding these applications enhances one’s ability to select the right tools and techniques for effective soil sampling. This section delves into specific areas where AMS soil samplers prove invaluable.
Agricultural Assessments
In the agricultural domain, soil quality is fundamental to crop yield. AMS soil samplers facilitate detailed soil analysis, enabling farmers to gauge nutrient levels and soil health. By collecting samples from different locations and depths, they can establish a comprehensive profile of the land.
Such assessments can reveal important information like:
- Nutrient Deficiencies: Identifying the lack of essential nutrients helps in applying appropriate fertilizers.
- Soil pH Levels: Adjusting soil pH can improve nutrient availability and promote healthy plant growth.
- Moisture Content: Understanding soil moisture is key for irrigation management.
The impact of these assessments leads to improved agricultural practices and sustainable land management. Farmers are able to maximize yield while minimizing environmental impact.
Environmental Monitoring
Environmental scientists utilize AMS soil samplers to monitor the condition of ecosystems. They collect data that helps in assessing soil health, assessing biodidiversity, and understanding the effects of various environmental factors.
This monitoring allows for:
- Baseline Data Collection: Establishing reference points for future studies.
- Impact Assessment: Evaluating the effects of pollution, climate change, or land use practices on ecosystems.
- Restoration Projects: Providing crucial data to inform ecological restoration efforts.
Through these efforts, AMS soil samplers contribute to maintaining ecological balance.
Contaminant Detection
Contaminant detection is another vital use of AMS soil samplers. Contaminants can severely affect soil health and water quality. By collecting soil samples at suspected contamination sites, researchers can analyze chemical and biological properties.
The benefits include:
- Identifying Contaminants: Soil samples can reveal the presence of heavy metals, pesticides, or other hazardous substances.
- Monitoring Remediation Efforts: Assessing the effectiveness of cleanup operations over time.
- Risk Management: Delivering data that assists in regulatory compliance and public health protection.
In summary, the applications of AMS soil samplers are diverse and impactful. Their role in agriculture, environmental monitoring, and contaminant detection cannot be underestimated. As research and technology progress, these soil samplers will undoubtedly continue to provide essential data, promoting both sustainability and scientific advancement.
Best Practices for Using AMS Soil Samplers
The effective use of AMS soil samplers requires understanding key practices that enhance the sampling process. Best practices ensure the reliability of the collected data and promote the longevity of the equipment. Adhering to these practices is crucial for researchers and professionals who aim to obtain accurate insights from soil analysis.
Maintenance and Care
Proper maintenance and care are essential for ensuring the optimal performance of AMS soil samplers. Regular cleaning after each use can prevent contamination and buildup of soil residue. Users should disassemble the sampler components, such as the sampling tubes and collection containers, and wash them with water. If necessary, a mild detergent can be used, but thorough rinsing is important to remove any soap traces.
Additionally, inspect the equipment for any signs of wear or damage. Problems such as rusting parts or bent components can lead to inaccurate sampling. Keeping a maintenance log can be beneficial to track repairs and servicing schedules. Regular maintenance not only preserves the quality of the samplers but also extends their lifespan.
Proper Storage Techniques
Storage techniques play a significant role in prolonging the life of AMS soil samplers. After cleaning, components should be dried completely before storage to prevent mold and corrosion. Each piece should be stored in a cool, dry place, away from direct sunlight, which can degrade materials over time.
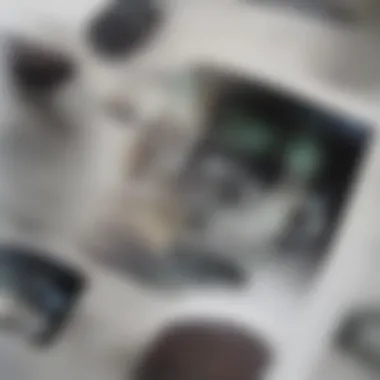
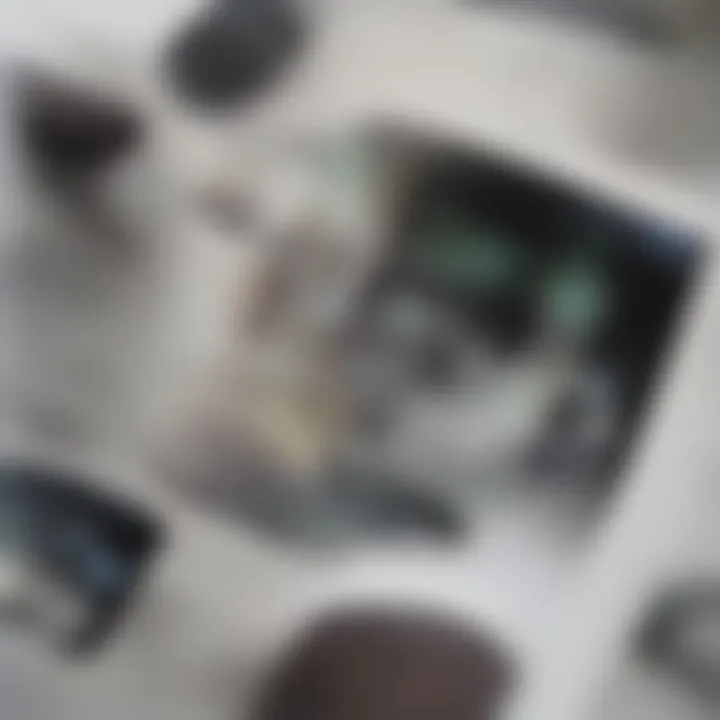
When possible, use protective cases or bags to prevent accidental damage. Organizing the equipment in a systematic manner can help in easy retrieval and reduce the likelihood of losing important components.
"Proper care and storage can significantly reduce replacement costs and ensure accuracy in soil sample results."
Some best practices for storage include:
- Label all components clearly for easy identification.
- Keep samplers secured to avoid movement or damage if stored in a shared space.
- Use silica gel packets in storage containers to absorb moisture.
By following these maintenance and storage practices, users of AMS soil samplers can enhance their sampling effectiveness and ensure their equipment remains in optimal condition for future use.
Challenges in Soil Sampling
Soil sampling is a critical process in environmental research and assessment. However, it is not without its challenges. Recognizing these challenges is essential for maximizing the accuracy and reliability of the data collected. This section addresses two primary factors that can significantly impact soil sampling: environmental variables and human or instrumental error. Both elements can affect the quality and interpretation of results.
Environmental Variables
Environmental variables are factors outside the control of the researcher that can influence the soil sampling process. These include weather conditions, soil moisture, temperature fluctuations, and even the presence of vegetation. For instance, wet conditions may lead to soil compaction, making it difficult to obtain representative samples. Additionally, high moisture levels can alter the chemical composition of soils, which may subsequently affect results.
To mitigate these issues, researchers should consider the following strategies:
- Conduct sampling during optimal conditions: Aim for periods of dry weather to reduce variability caused by excess moisture.
- Document environmental conditions: Track weather data at the time of sampling to provide context for any anomalies in results.
- Use standardized methods: Employ consistent procedures during sampling to reduce reliance on fluctuating environmental factors.
By implementing these measures, researchers can reduce the adverse effects of environmental variables, thus enhancing the validity of their findings.
Human and Instrumental Error
Human and instrumental errors are significant sources of potential bias in the soil sampling process. Human error can arise from incorrect sample collection techniques, poor judgment in selecting sampling sites, or failure to adhere to established protocols. Instrumental error, on the other hand, may occur due to faulty or improperly calibrated sampling equipment.
To address these issues, the following practices can be useful:
- Training and continuous education: Ensure that personnel involved in sampling are well-trained. Regular workshops can help maintain high standards.
- Routine equipment checks: Regular calibration and maintenance of soil sampling tools minimize instrumental errors.
- Implementing double-checking protocols: Having a second person verify the collection process can significantly reduce errors caused by oversight.
"Awareness of potential errors and fostering a culture of precision can greatly enhance the reliability of soil sampling data."
By focusing on both human capability and instrument reliability, researchers are better equipped to conduct effective soil sampling. Overall, understanding these challenges allows for more rigorous and careful planning of soil sampling efforts. This proactive approach ultimately leads to more accurate and meaningful results.
Future Directions in Soil Sampling Techniques
The realm of soil sampling is witnessing a transformation driven by advancements in technology and methodological approaches. As environmental challenges become more intricate, the demand for accurate and efficient soil analysis grows. Future directions in soil sampling techniques are crucial for improving data quality, enhancing precision, and streamlining workflows, which is particularly relevant for researchers and professionals aiming for more robust data in their studies. Understanding these emerging trends can lead to significant improvements in agricultural and environmental research.
Technological Innovations
Emerging technologies play a pivotal role in advancing soil sampling practices. Innovations such as drone-assisted soil sampling, remote sensing, and portable soil analysis tools are reshaping the landscape. Drones can cover large agricultural areas quickly, providing spatial data that human operators cannot match. Remote sensing technologies offer insights into soil health by measuring parameters like moisture levels and nutrient concentrations from the air.
Moreover, portable devices that analyze soil composition on-site are gaining traction. These tools dramatically reduce the wait time associated with laboratory testing. As these technologies develop, they may integrate seamlessly into existing methodologies, allowing for real-time data collection.
Consider the following benefits of these innovations:
- Increased Efficiency: Reducing the time required for sampling and analysis.
- Cost-Effectiveness: Minimizing transportation and laboratory expenses.
- Enhanced Accuracy: Leveraging advanced sensors to obtain more precise readings.
Integration of AI in Soil Analysis
Artificial intelligence (AI) is set to revolutionize soil analysis further. Machine learning algorithms can process vast amounts of data from diverse sources, identifying patterns that would be difficult for humans to discern. By implementing AI in soil sampling techniques, researchers can generate predictive models that assess soil health and its suitability for specific crops.
AI can also optimize sampling strategies by:
- Identifying Optimal Sampling Locations: Suggesting where to sample based on historical data and real-time conditions.
- Predicting Soil Variability: Offering insights into how different conditions affect soil properties.
- Automating Data Analysis: Streamlining the interpretation of results.
The integration of AI not only enhances the analytical capabilities but also contributes to more sustainable agricultural practices by aligning crop choices with local soil conditions. As these technologies advance, their adoption will likely lead to more informed decisions and effective interventions in both environmental management and agricultural productivity.
"The future of soil analysis relies heavily on continuous innovation and adaptability to meet the needs of our changing environments."
In summary, the future directions in soil sampling techniques underscore the importance of technological advancements and AI integration. These innovations promise to improve the precision, efficiency, and effectiveness of soil analysis, thereby enabling more sustainable practices in agriculture and environmental conservation. Continued investment in these areas will empower researchers and professionals to address pressing ecological challenges with greater efficacy.
Epilogue
The conclusion serves as a vital component in any comprehensive article on AMS soil samplers. It consolidates the information presented throughout the document and reiterates the significance of such samplers in various spheres of environmental research. The focus here is on three specific elements: the key takeaways, the practical implications, and the ongoing relevance of soil sampling techniques.
Summarizing Key Points
In summarizing the key points, it is crucial to revisit the main themes discussed in the previous sections. AMS soil samplers are designed specifically for accurate soil sampling, enabling researchers to conduct effective analysis. The various types of samplers—core, auger, and screw-type—each offer unique benefits and are suited to differing contexts and requirements.
Moreover, the discussion around techniques for effective sampling highlights the importance of correct location selection, depth determination, and integrity of samples. Best practices for maintenance and care have also been articulated, ensuring that samplers remain in optimal condition for use. As a final point, the challenges faced in soil sampling, including environmental variables and human errors, have been addressed, emphasizing the need for constant improvement.
Implications for Scientific Research
The implications for scientific research from the findings of this article are significant. First, the understanding of AMS soil samplers enhances the capability of researchers in collecting reliable data. Accurate soil sampling is fundamental for agricultural assessments, environmental monitoring, and contaminant detection. The importance of using the right type of sampler cannot be overstated—it influences the integrity of the data collected and the conclusions drawn from these analyses.
Furthermore, technological innovations, such as the integration of artificial intelligence in soil analysis, signal a future where research methodologies will be even more refined. These advancements will likely facilitate quicker and more accurate assessments in various fields, further enhancing the value of effective soil sampling practices.
In summary, the avenues for research opened by the effective use of AMS soil samplers have meaningful implications for policy, practice, and theoretical understanding within environmental science. Researchers must continue to adapt and evolve in their techniques, embracing new technologies to ensure accuracy and relevance in their studies.