Protein Identification Techniques Using Mass Spectrometry
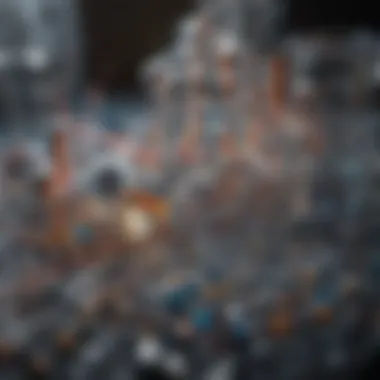
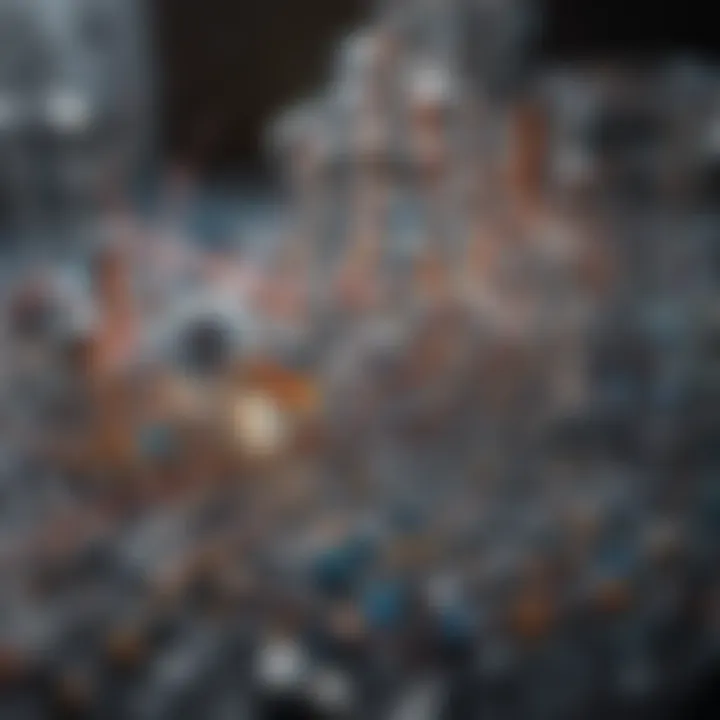
Intro
Mass spectrometry has emerged as a linchpin in the field of proteomics, a domain that focuses on the large-scale study of proteins. As researchers strive to unravel the complexities of biological systems, the need for precise techniques to identify proteins becomes essential. Mass spectrometry provides a robust, versatile method for this task, allowing scientists to delve into the intricate world of proteins at a molecular level. By converting molecules into ions and measuring their mass-to-charge ratios, mass spectrometry offers a clear lens through which the vast landscape of proteins can be explored.
Through the pages of this article, we will embark on a journey that outlines key methodologies and principles of mass spectrometry in protein identification. You will gain insights into sample preparation techniques, data analysis, and the ever-evolving technologies reshaping the field. What’s more, we will cover both the advancements that have driven progress and the challenges that remain, ensuring a nuanced understanding of this fascinating subject.
With an emphasis on biological research and clinical applications, this article will serve as a comprehensive guide for students, researchers, educators, and professionals alike. Prepare to dive deep into the nuts and bolts of mass spectrometry and its pivotal role in advancing our grasp of proteomics.
Prelims to Protein Identification
In the realm of biological research, the ability to accurately identify proteins is tantamount to understanding life at a molecular level. Proteins play critical roles in a myriad of cellular processes, and recognizing their structure, function, and interactions paves the way for advancements in various fields such as medicine, biochemistry, and biotechnology. In this context, the significance of protein identification cannot be overstated—it forms the foundation for discoveries that can drive therapeutic innovations, enhance diagnostic techniques, and unravel complex biological mechanisms.
Importance of Protein Identification
Protein identification is like finding the missing pieces of a jigsaw puzzle that depicts the intricate world of biological systems. When researchers pinpoint protein identities, they gain insights into biological pathways, disease mechanisms, and potential drug targets. This is especially crucial when examining the roles of proteins in diseases such as cancer or Alzheimer’s.
- Understanding mechanisms: By knowing which proteins are involved in specific cellular functions, researchers can theorize about the underlying disruptions that might lead to disease.
- Biomarker discovery: Identifying proteins that serve as biomarkers can lead to earlier diagnosis and personalized medicine.
- Drug development: Targeting specific proteins has become a dominant strategy in drug design, tailored to engage with the proteins involved in particular conditions.
From an educational perspective, imparting knowledge about protein identification enriches the academic curriculum, equipping students with skills pertinent to modern scientific inquiry. Thus, grasping the nuances of this topic benefits not only practitioners in the field but also the next generation of scientists who will carry the torch forward.
Overview of Mass Spectrometry
Mass spectrometry stands as one of the cornerstones in the toolbox of protein identification. It is a sophisticated analytical technique that measures the mass-to-charge ratio of ions, thereby allowing for the characterization of protein structures, molecular weights, and their abundance in a complex mixture.
At its core, mass spectrometry consists of three key stages: ionization, mass analysis, and detection.
- Ionization: The initial step involves converting molecules into ions. This is where the beauty of methods like Electrospray Ionization (ESI) and Matrix-Assisted Laser Desorption/Ionization (MALDI) shine, enabling the introduction of large biomolecules into the mass spectrometry platform.
- Mass Analysis: Once ionized, the ions are sorted according to their mass-to-charge ratios. Different mass analysis techniques come into play, such as Time-of-Flight (ToF) and Quadrupole Mass Filters, each with its own strengths in terms of resolution and speed.
- Detection: The final stage involves capturing the data generated from the separated ions, providing a spectrum that scientists can interpret to determine the protein's identity.
Mass spectrometry lacks textile flourishes, yet possesses an elegance in its precision. It allows for a detailed examination of complex biological samples, leading to insights that were previously elusive. With the capability to work on low-abundance proteins and complex mixtures, mass spectrometry enhances our understanding of the proteome—a feat indispensable to the modern life sciences.
"Mass spectrometry does not just analyze samples; it reveals stories written in the language of proteins."
By providing clarity on the critical roles that mass spectrometry plays in protein identification, we can appreciate its value as a powerful ally in bridging the gaps in scientific knowledge.
Fundamental Principles of Mass Spectrometry
Understanding the fundamental principles of mass spectrometry (MS) is crucial for interpreting and utilizing its capabilities for protein identification. Mass spectrometry operates on the basis of measuring the mass-to-charge ratio of ions. This method enables researchers to discern the structure, quantity, and composition of different proteins in complex biological samples.
One of the primary benefits of mass spectrometry is its sensitivity. Even at low concentrations, it can provide detailed information while maintaining a high degree of accuracy. This characteristic allows for the identification of proteins in small sample volumes, making it an ideal choice for clinical and research settings where sample availability might be limited. The robustness of mass spectrometry in handling complex mixtures further enhances its appeal, particularly in proteomics, where various proteins might be present in extremely diverse amounts.
Basic Components of Mass Spectrometry
To grasp how mass spectrometry works, it's vital to familiarize ourselves with its basic components. The core elements include the ion source, the mass analyzer, and the detector. The ion source generates ions from the sample to be analyzed. These ions are then sorted by the mass analyzer based on their mass-to-charge ratios. Finally, the detector captures these sorted ions, producing a mass spectrum that provides insightful data about the protein composition.
Mass Analysis Techniques
Time-of-Flight (ToF)
Time-of-Flight mass spectrometry is widely recognized for its high energy resolution and rapid analysis times. The fundamental aspect of ToF is the brief time that ions take to travel a fixed distance; lighter ions travel faster than heavier ones. This property allows ToF to provide precise mass measurements, making it a popular choice for analyzing peptide mixtures. Additionally, its ability to process multiple ions simultaneously defines its utility in high-throughput environments, thus addressing the need for efficiency.
However, the arrangement of the apparatus can be rather intricate. While the technology is generally reliable, the sensitivity to electrical and magnetic fields requires careful attention during operation. Despite these considerations, the benefits often outweigh the disadvantages, particularly in research settings emphasizing speed and precision.
Quadrupole Mass Filters
Quadrupole Mass Filters are notable for their versatility and reliability. This technique utilizes a set of four rods to filter ions based on their mass-to-charge ratios. By varying the electric fields applied to the rods, only ions of a particular mass can pass through to the detector. What sets quadrupole filters apart is their capability for selective analysis, allowing for the identification of specific ions in the presence of others.
The key advantage is the ability to perform tandem mass spectrometry (MS/MS) for deeper insight into molecular structure. On the flip side, while quadrupole filters can handle a range of masses, they can be less sensitive compared to other techniques, particularly when looking at low-abundance peptides.
Ion Trap Mass Spectrometry
Ion trap mass spectrometry employs electric or magnetic fields to trap ions for analysis, which allows for highly sensitive measurements. One unique feature is its ability to conduct various types of experiments like MS/MS, where it can fragment selected ions to provide detailed structural information. This probing capability contributes significantly to elucidating the composition of complex protein structures.
While ion traps can be extremely sensitive, they do have limitations. The smaller the trap, the more challenging it can be to analyze large proteins due to space constraints. Nevertheless, the advantages of versatile structural information often make them invaluable in protein analysis.
Ionization Methods
Effective ionization is pivotal in mass spectrometry as it directly affects the quality of the mass spectrum obtained. Several methods exist, each with its own strengths and setbacks.
Electrospray Ionization (ESI)
Electrospray Ionization is widely favored for its ability to create ions from large biomolecules without significant fragmentation. This method works by applying a high voltage to a solution to disperse it into tiny droplets, which then evaporate to release ions into the gas phase. ESI is particularly suitable for analyzing proteins because it maintains their native state, preserving the intricacies of their structure.
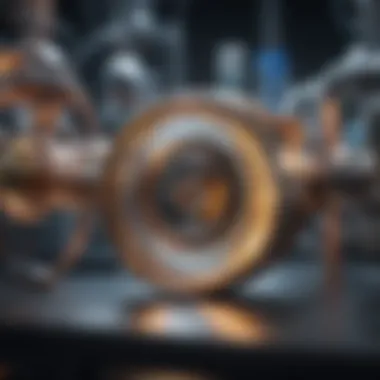
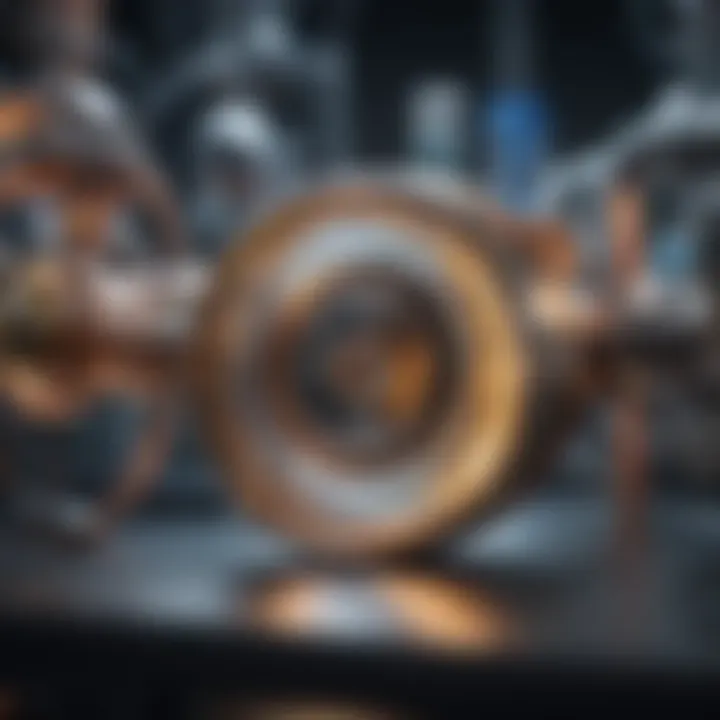
However, a drawback of ESI can be its susceptibility to changes in solution conditions, which can alter the ionization efficiency. Finding the right conditions for a specific protein can be time-consuming. Nonetheless, when optimal conditions are achieved, ESI provides great sensitivity and is an essential tool in the researcher’s toolkit.
Matrix-Assisted Laser Desorption/Ionization (MALDI)
MALDI is another crucial ionization method that stands out for its ability to analyze large molecules, including proteins. The process involves embedding the sample in a matrix material that absorbs laser light, leading to the desorption and ionization of the proteins. One of its chief characteristics is the minimal fragmentation that occurs, making it easy to identify large biomolecules intact.
MALDI's advantage lies in its speed and effectiveness when dealing with complex mixtures. However, it requires careful matrix selection and sample preparation, or else it can lead to issues like signal suppression. Even with these challenges, MALDI continues to be a valuable method in mass spectrometry labs worldwide.
"A deep understanding of these principles equips researchers with the insight needed to navigate the complexities of protein identification and reveal the nuances of proteomics."
In summary, grasping the fundamental principles of mass spectrometry, coupled with the appropriate mass analysis techniques and ionization methods, is essential for effective protein identification. Each method discussed has its unique features, advantages, and certain limitations. Collectively, they serve to enhance our capabilities in exploring the intricate world of proteins.
Sample Preparation for Mass Spectrometry
Sample preparation stands as a pivotal step in the realm of mass spectrometry, significantly influencing the quality and reliability of the results obtained. Preparing samples properly not only enhances the quantification of proteins but also minimizes potential interferences that could skew the data. It is akin to laying a solid foundation before erecting a house; if the base is unstable, the structure can collapse in the face of external pressures. In this context, the goal is to maintain the biological fidelity of the sample while ensuring that it is amenable to mass spectrometric analysis.
Overview of Sample Preparation Techniques
Several techniques are employed during sample preparation to fine-tune the samples for analysis. Each method has its own set of advantages and challenges. Some common techniques include:
- Precipitation methods: These often involve solvents such as ethanol or acetone to selectively precipitate proteins from solutions.
- Filtration techniques: This helps to eradicate particulates and concentrate proteins, making it easier for further analysis without clogging.
- Chromatographic techniques: These methods, like affinity chromatography, target specific proteins of interest, allowing for their isolation from complex mixtures.
Understanding these methods thoroughly is essential for researchers aiming to optimize their protocols for protein analysis, which will directly impact downstream applications.
Protein Extraction and Purification
Protein extraction is the first crucial step in sample preparation. It leads directly to the purity and integrity of the proteins being analyzed. The extraction process should be tailored based on the source of the proteins, whether they are derived from cells, tissues, or biological fluids.
- Homogenization: This is often the first step in extracting proteins, involving mechanical forces to break down cells.
- Buffer selection: Choosing the right buffer is essential to maintain protein stability throughout the extraction process. The pH, ionic strength, and presence of inhibitors are critical factors to consider.
The purification stage typically employs chromatographic techniques to isolate proteins based on different properties like size, charge, and affinity, ensuring that contaminants are removed to yield a cleaner sample for analysis.
Digestion Methods
Once proteins are extracted and purified, digestion methods come into play. This step is essential, especially for the identification of proteins by breaking them down into smaller peptides, which are easier to analyze by mass spectrometry.
Enzymatic Digestion
Enzymatic digestion is a popular method within this context. It involves using enzymes, most frequently trypsin, which cleave peptide bonds at specific amino acids. This method is favored primarily because it yields peptides of suitable lengths for mass spectrometric analysis.
- Key characteristic: One of its strengths lies in its specificity; trypsin cleaves at the carboxyl side of lysine and arginine.
- Benefits: With enzymatic digestion, the resulting peptides often exhibit good solubility in the solvent used in mass spectrometric analysis, which is essential for achieving reliable data.
However, one drawback may be the time required for the digestion process, especially when dealing with complex samples.
Chemical Digestion
Chemical digestion, on the other hand, employs reagents to break down protein structures. This method can be particularly effective when proteins are resistant to enzymatic action or when the enzymatic approach is too slow.
- Key characteristic: Chemical digestion is usually more rapid; it can accomplish digestion in a matter of minutes compared to the longer enzymatic method.
- Benefits: It provides greater control over the digestion parameters and can be standardized more easily than enzymatic methods.
The challenge, though, lies in the potential for introducing modifications or alterations to the peptides that may not occur during enzymatic digestion.
As one can see, both enzymatic and chemical digestions are crucial to preparing proteins for mass spectrometry while each has its own suite of advantages and potential pitfalls. A thorough understanding of these methods is imperative for any researcher delving into the intricacies of protein identification through mass spectrometry.
Data Acquisition in Mass Spectrometry
Data acquisition serves as the backbone of mass spectrometry, fundamentally influencing the quality and reliability of the results obtained during protein identification. It encompasses the collection of mass spectral data, which is essential for the analysis of biomolecules. A robust data acquisition strategy is vital as it determines the accuracy of the measurements and the resultant identification of proteins. In this section, we'll delve deeper into the two main aspects of data acquisition: acquisition strategies and real-time data monitoring.
Acquisition Strategies
Several acquisition strategies can dictate how mass spectra are gathered. Various settings accompany these strategies which can have profound effects on sensitivity, resolution, and speed. Some of the commonly employed strategies are:
- Full Scan: Collecting information across a broad mass range, giving a comprehensive overview of all ions present.
- Selected Ion Monitoring (SIM): Targeting specific ions improves sensitivity, allowing for better detection of low-abundance proteins.
- Scan Mode Averaging: This method averages multiple scans to minimize noise, enhancing data quality.
- Data-Dependent Acquisition (DDA): Here, the system makes real-time decisions to select ions for further analysis, providing a balance between broad data collection and specific detailing.
Each of these strategies has its unique set of advantages. The implementation of the right approach can lead researchers to insights far richer than any standalone data would provide. It’s crucial, therefore, to align the choice of acquisition strategy with the experimental goals while considering trade-offs in sensitivity and specificity.
Real-Time Data Monitoring
Real-time data monitoring allows scientists to keep a closer eye on the ongoing analysis, making it easier to adapt quickly if something goes awry. By tracking the data as it comes in, researchers can instantly determine whether their methods are successful or if adjustments are necessary.
Key benefits of real-time data monitoring include:
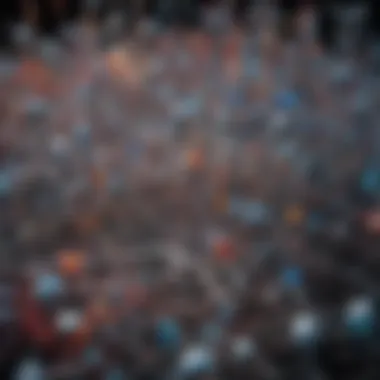
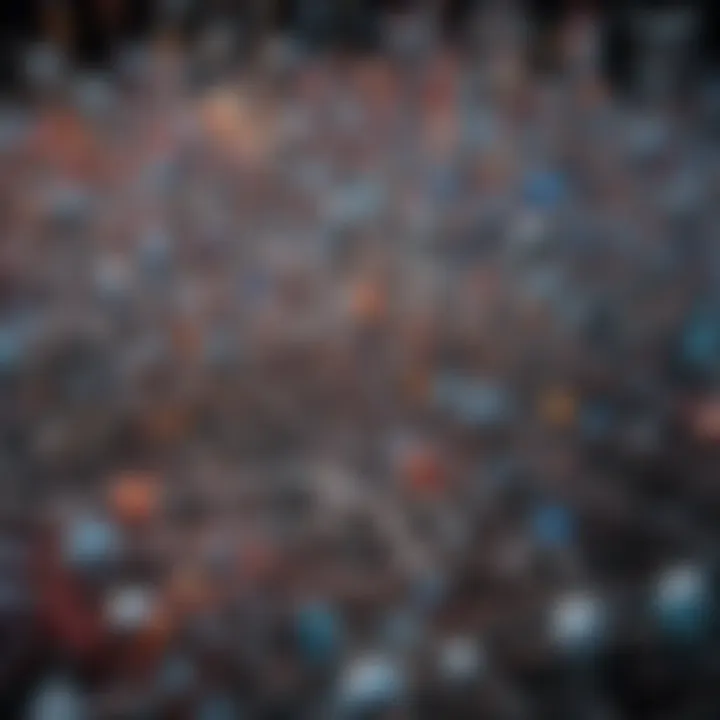
- Immediate Feedback: Researchers can see variations in the data and troubleshoot issues in real-time rather than waiting until the entire dataset is collected.
- Dynamic Experimentation: Adjustments to settings like ionization parameters or acquisition conditions can be made mid-experiment to optimize results.
- Error Reduction: Continuous observation helps in spotting anomalies or instrument drifts, reducing the risk of misinterpretation and allowing for immediate recalibration of instruments.
Incorporating real-time monitoring is not just about collecting more data; it’s about enhancing analytical precision and achieving reliable results.
Acquiring accurate data in real-time can empower scientists to navigate the complexities of sample analysis effectively, reflecting the constantly evolving nature of mass spectrometric techniques.
By understanding and implementing effective data acquisition strategies along with diligent real-time monitoring, researchers can significantly improve their capacity for protein identification, thus driving advancements in various fields like proteomics, clinical diagnostics, and biochemistry.
Data Interpretation and Analysis
Data interpretation and analysis are the backbone of mass spectrometry, particularly in the context of protein identification. Once the spectrum is acquired, the real work begins. Understanding how to decode these often complex data sets is key. The importance can’t be overstated, as incorrect interpretations can lead to flawed conclusions. This section delves into how researchers make sense of the data, what tools they employ, and why it matters so much in the larger field of proteomics.
Mass Spectral Analysis Techniques
When analyzing mass spectra, one must consider various techniques. Each has its own strengths and weaknesses, often dictating whether a specific approach is suitable for a given challenge. For example, some methods provide high sensitivity while others might excel in speed. Several techniques are crucial:
- Fragmentation Analysis: Provides insights into the structure by breaking peptides into smaller pieces.
- Isotope Pattern Analysis: Explores isotopic distributions to confirm identities.
These techniques permit nuanced interpretations of the spectra, allowing scientists to distinguish between closely related proteins or variants of the same protein.
Software Tools for Data Analysis
In today's world, the sheer volume of data generated by mass spectrometers demands powerful software tools for analysis. Researchers increasingly rely on specialized programs tailored for this purpose. Some popular tools include:
- MaxQuant: Great for quantitative proteomics.
- Mascot: A well-regarded search engine for peptide identification.
- Proteome Discoverer: Provides extensive functionalities for protein identification.
These tools streamline the process of matching observed mass ratios to protein databases, facilitating quicker and more accurate identification of proteins within complex mixtures.
Identifying Proteins from Spectra
Choosing the appropriate method to identify proteins from mass spectrometric data is critical. Two notable methodologies stand out: Peptide Mass Fingerprinting and Tandem Mass Spectrometry (MS/MS).
Peptide Mass Fingerprinting
Peptide Mass Fingerprinting revolves around identifying proteins based on the unique mass-to-charge ratios of their peptides. This technique enjoys popularity due to its relatively straightforward approach. Researchers generate a mass spectrum of the digested peptides and compare the resulting peptide masses against known databases.
- Key Characteristic: Its simplicity in interpretation.
- Unique Feature: It requires minimal sample preparation compared to other methods.
- Advantages: Quick identification can significantly speed up research processes.
- Disadvantages: It may struggle with highly complex protein mixtures, as overlapping masses can lead to inaccuracies.
Tandem Mass Spectrometry (MS/MS)
Tandem Mass Spectrometry (MS/MS) takes protein identification a step further by allowing fragmentation of selected precursor ions. This approach leads to detailed interactions that can more accurately determine the structure of proteins.
- Key Characteristic: Its ability to produce high-resolution data by analyzing fragments.
- Unique Feature: It generates information not just about the molecular weight but also the sequence of amino acids.
- Advantages: Capable of identifying proteins from extremely complex samples.
- Disadvantages: It requires more time and is often technically more challenging than Peptide Mass Fingerprinting.
"Unlocking the potential of spectral data requires not just technical skill but insight into the biological relevance of findings."
Technological Advancements in Mass Spectrometry
Technological advancements in mass spectrometry have fundamentally reshaped the landscape of protein identification. These innovations have not only enhanced the sensitivity and resolution of mass spectrometric techniques but have also broadened the applicability of mass spectrometry in both research and clinical diagnostics. With the rapid pace of technological development, it is pivotal to understand how these advancements play a crucial role in increasing the accuracy and efficiency of protein analyses.
One noteworthy benefit is the increased throughput achieved through next-generation mass spectrometry methods. These newer systems are designed to handle complex samples more effectively and are capable of processing larger volumes of data simultaneously. The result is a more detailed, multi-faceted view of protein populations within a sample, allowing for comprehensive insights that earlier methods simply could not provide.
Another significant advancement is the integration of mass spectrometry with other analytical techniques. This combination not only provides complementary information but also enhances detection capabilities for compounds that might otherwise remain elusive. Through such integrations, researchers can glean information about protein localization, interactions, and modifications with a granularity that was previously unimaginable.
Next-Generation Mass Spectrometry
Next-generation mass spectrometry marks a significant leap forward in the world of protein identification. These systems harness cutting-edge technologies that leverage increased resolution, sensitivity, and dynamic range to analyze proteins.
Specifically, these advanced spectrometers often employ enhanced ionization techniques and improved detectors. Such upgrades lead to clearer spectral data and a greater ability to identify low-abundance proteins that might go undetected with traditional mass spectrometry approaches. Moreover, with automation and software improvements, data acquisition has become faster and more user-friendly.
In practical terms, Next-Gen technologies, such as high-resolution mass spectrometry, enable researchers to distinguish subtle differences in similar protein species. This is especially critical for applications in drug development, where identifying precise protein targets can make all the difference in therapeutic efficacy.
Integration with Other Technologies
The integration of mass spectrometry with complementary technologies exponentially increases its power and versatility in protein identification. By combining mass spectrometry with other analytical methodologies, researchers can extract more comprehensive data from their samples.
Liquid Chromatography-Mass Spectrometry (LC-MS)
Liquid Chromatography-Mass Spectrometry, commonly referred to as LC-MS, is a hallmark example of integration in mass spectrometry. This pairing allows for the efficient separation and identification of complex protein mixtures.
The key characteristic of LC-MS lies in its two-step process: the liquid chromatography step separates proteins based on their chemical properties, while the mass spectrometry phase quantifies and identifies them. This synergy is advantageous because it systematically simplifies complex samples, making it easier to pinpoint specific proteins of interest.
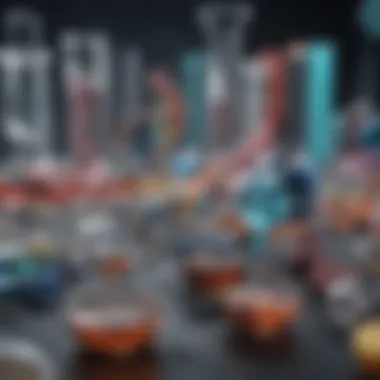
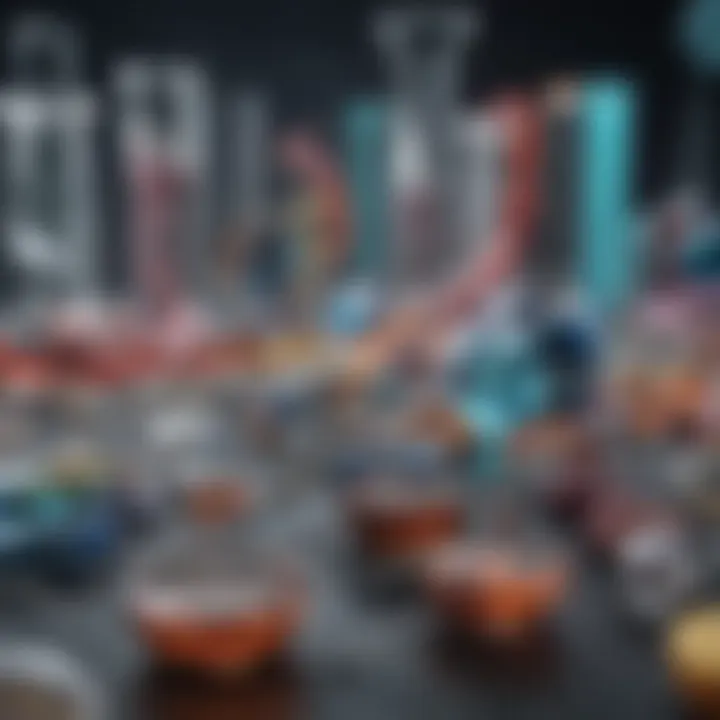
A unique feature of LC-MS is its high-throughput capability, which makes it a beneficial choice for both large-scale proteomic studies and clinical applications. However, one must consider that while LC-MS enhances sensitivity, it often requires meticulous sample preparation and method validation to achieve optimal results.
Mass Spectrometry Imaging
Mass Spectrometry Imaging, or MSI, is yet another innovative advancement worth discussing. This technique allows researchers to visualize the spatial distribution of proteins directly within tissue samples or complex biological matrices.
The key characteristic of MSI is its ability to provide spatial context to proteomic studies, offering an insightful glimpse into how proteins are localized within biological systems. This feature is particularly beneficial for understanding disease progression and underlying mechanisms at the tissue level. The unique integration of imaging at the molecular level with mass spectrometric analysis enables a holistic understanding of protein behavior.
Nevertheless, the adoption of MSI comes with its challenges, notably high computational demands and the need for substantial technical expertise. Yet, the rewards—detailed spatial mapping and complementary biological contexts—underscore its promising potential.
Challenges in Protein Identification
Identifying proteins through mass spectrometry is no cakewalk; even seasoned researchers often face hurdles. Understanding these challenges is crucial in refining the methods used and improving the reliability of results. Precision in protein analysis isn’t just beneficial—it’s vital in both research contexts and clinical applications. To truly grasp the landscape of protein identification, it’s essential to shine a light on the specific elements causing difficulties.
Complex Mixture Analysis
Biological samples are rarely simple; they resemble a mixed bag more than a neatly packaged product. Complex mixtures, such as those found in cell lysates or tissue extracts, present unique challenges. For instance, if you’ve ever tried to make sense of a crowded event, you feel the challenge: spotting an acquaintance in a throng can be daunting. Similarly, mass spectrometry has to sift through a myriad of substances to find the proteins of interest.
Dealing with this level of complexity brings several considerations. First, there’s the issue of dynamic range. Some proteins exist in such low concentrations compared to others that they’re practically invisible in mass spectrometric analysis. Techniques like fractionation or enrichment may be employed to counteract this.
- Fractionation helps to separate proteins before analysis, potentially revealing high-value targets lost in the mix.
- Enrichment could involve focusing on specific classes of proteins, which significantly improves detectability.
In addition, the presence of contaminants can lead to misinterpretation of data. There are cases where a protein in a minor quantity gets overshadowed by a more abundant contaminant, skewing results. The galling part about this is that even with state-of-the-art instruments, without careful preparation and planning, one might get misleading results.
Post-Translational Modifications
Protein functionality often hinges on post-translational modifications (PTMs) like phosphorylation, glycosylation, and acetylation. These modifications are akin to alterations in a recipe that tweak the final dish’s flavor. While mass spectrometry is adept at identifying these modifications, it doesn’t always make it easy. Identifying a protein in its modified state requires in-depth analytical know-how.
Why is this significant? PTMs play crucial roles in regulating biological processes. They can determine protein functionality, localization, or interaction with other molecules. Ignoring them opens a Pandora’s box of potential misinterpretations regarding how proteins perform their roles within the cell.
Consider the challenge this way: imagine you are trying to follow a recipe without noticing that certain ingredients (or modifications) have been swapped out. You’d end up with a completely different dish!
In the protein identification realm, failing to recognize these modifications can lead researchers astray. Bioinformatics tools, and databases that catalog known modifications, are of utmost importance here. They assist scientists to identify potential PTMs during analysis but also require constant updating as new research surfaces.
Identifying proteins and understanding their PTMs is not simply about cataloging. It's about understanding their function, which is crucial for drug development and disease understanding.
In summary, both complex mixture analysis and the scrutiny of post-translational modifications introduce nuances to protein identification that cannot be overlooked. Navigating these challenges is essential in leveraging mass spectrometry effectively to make advancements in proteomics.
Future Directions in Mass Spectrometry for Proteomics
The role of mass spectrometry in proteomics continues to expand, reflecting the increasing complexity of biological systems. As we look ahead, we can see significant advancements that promise to redefine how proteins are identified and analyzed. This section is crucial, as it outlines the innovations that are shaping the future landscape of protein analysis.
Emerging Trends and Innovations
Several trends are on the horizon, driving the next wave of discoveries in mass spectrometry:
- Microfluidics Integration: This technology allows for the manipulation of fluids at a microscopic scale. Enhanced capabilities in sample handling and reaction control can improve the sensitivity and efficiency of mass spectrometric analysis.
- Artificial Intelligence and Machine Learning: AI algorithms are becoming a game changer in data interpretation. They assist in identifying patterns within complex datasets, which can expedite the search for specific proteins or modifications that might otherwise go unnoticed.
- High-Resolution Mass Spectrometry: Instruments with improved resolution are increasingly accessible, greatly enhancing our ability to distinguish between closely related protein species, which is vital for accurate identification.
- Single-Cell Proteomics: Advancements in technology allow scientists to analyze proteins at the single-cell level, providing deeper insights into cellular heterogeneity and the dynamics of biological processes.
- Spatial Proteomics: Techniques that combine mass spectrometry with imaging are gaining traction. This fusion enables researchers to observe the spatial distribution of proteins in tissues, enhancing the understanding of their functional roles.
As these trends develop, they promise more accurate and detailed proteomic analyses, leading to exciting new findings in biology and medicine.
Potential Applications in Therapy and Diagnostics
The innovations in mass spectrometry are not just theoretical; they hold the key to revolutionary applications in various fields:
- Biomarker Discovery: By identifying specific proteins associated with diseases, mass spectrometry can play a pivotal role in the development of diagnostic tools that allow for early detection of conditions such as cancer or autoimmune disorders.
- Personalized Medicine: Tailoring treatments based on an individual’s proteomic profile is becoming a reality. Mass spectrometry can help in creating targeted therapies that are more effective and reduce adverse effects.
- Vaccine Development: Understanding the protein profiles of pathogens can improve vaccine design, making them more effective in eliciting the desired immune response.
- Drug Development: Mass spectrometry aids in identifying how drugs interact with their targets at the protein level, facilitating the creation of more efficient therapeutics.
As these applications unfold, it paints a promising picture of how mass spectrometry can not only improve our understanding of biological systems but also lead to tangible advancements in healthcare and treatment methodologies.
"The future of proteomics is poised to redefine our approach to medicine, offering insights and treatments tailored to individual needs."
By staying attuned to these advancements, researchers, students, and professionals can prepare for a future where mass spectrometry is an even more integral part of biological and clinical research. The journey ahead is filled with potential, awaiting those willing to explore its depths.
The End
Mass spectrometry stands out as a cornerstone in the identification of proteins, driving forward the field of proteomics and setting the stage for advancements in biological and clinical research. This article laid the groundwork for understanding how mass spectrometry operates, the methodologies involved in protein analysis, and the challenges facing researchers today.
Summary of Key Insights
Throughout the discussion, several pivotal points emerged that underscore the significance of mass spectrometry in identifying proteins. First and foremost, the intricate sample preparation processes—including protein extraction, purification, and digestion methods—are essential for obtaining quality data. The techniques utilized for data acquisition are equally crucial, ensuring that real-time monitoring and effective acquisition strategies yield reliable results. Furthermore, the exploration of data interpretation and analysis techniques, from peptide mass fingerprinting to tandem mass spectrometry, provided a comprehensive picture of how raw data transitions into meaningful insights about protein identities.
The role of technological advancements emerged as a prominent theme, highlighting innovations such as next-generation mass spectrometry and integrations with technologies like liquid chromatography. These advancements not only enhance the breadth and accuracy of protein identification but also catalyze new applications in therapeutic and clinical contexts. In response to the various challenges, such as the complexities of analyzing protein mixtures and understanding post-translational modifications, it becomes apparent that the field is evolving continuously as researchers seek innovative solutions.
Final Thoughts on the Future of Protein Analysis
As we peer into the future, it is evident that mass spectrometry will only become more integral in the realm of protein analysis. Emerging trends suggest that the ongoing refinement of mass spectrometry techniques will facilitate deeper insights into protein functions, interactions, and structures. Additionally, the potential applications in areas like personalized medicine and targeted therapies continue to expand, signaling a promising horizon.
In essence, the field of mass spectrometry remains ripe with possibilities. As technologies and methodologies continue to advance, the capacity for researchers to unlock the secrets hidden within proteins will flourish, paving the way for groundbreaking discoveries that could redefine the landscape of biological research.