Proton Therapy Simulation: Comprehensive Insights
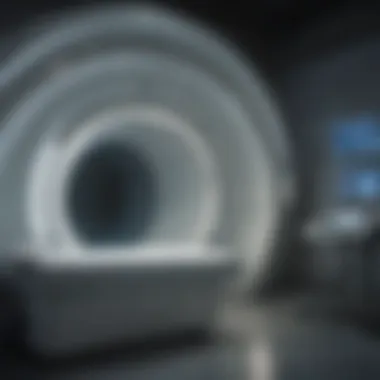
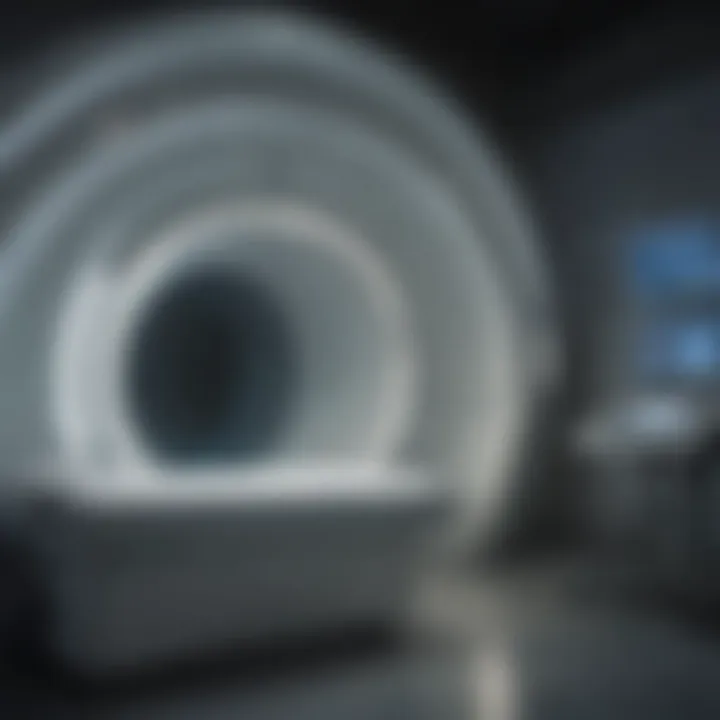
Intro
Proton therapy simulation plays a critical role in the treatment of cancer patients through proton beam therapy. The process involves planning and executing precise treatments tailored to the individual patient's needs. As the field evolves, understanding the fundamentals of proton therapy simulation becomes essential for optimizing patient outcomes.
Research Overview
Key Findings
Proton therapy simulation has been shown to significantly improve treatment precision. A major finding is that it can effectively target tumors while minimizing damage to surrounding healthy tissues. This is particularly crucial for pediatric patients, where preserving healthy tissue is vital.
Another key finding pertains to the technological advancements in imaging techniques. These developments enhance the accuracy of the treatment planning process. Advances have led to the use of magnetic resonance imaging (MRI) and computed tomography (CT) scans, which provide clearer insights into tumor location and size.
Study Methodology
A variety of methodologies are employed in current research. Various clinical studies utilize retrospective analyses of patient data to evaluate the effectiveness of proton therapy simulation. Others explore comparative studies against traditional photon therapy methods. Utilizing these diverse methods helps in obtaining a holistic view of the advantages presented by proton therapy.
Background and Context
Historical Background
The beginnings of proton therapy can be traced back to the mid-20th century. Initially, the technology was limited and primarily experimental. Over decades, as more research emerged, the benefits of proton beam therapy became increasingly evident, leading to its broader application in oncology.
Current Trends in the Field
Currently, the field of proton therapy is witnessing rapid growth. New treatment facilities are being established globally, expanding access to this advanced treatment. Moreover, ongoing research is focusing on integrating artificial intelligence and machine learning into simulation processes, aiming to predict treatment outcomes more accurately.
Prolusion to Proton Therapy Simulation
Proton therapy simulation is a fundamental aspect of preparing treatment protocols for patients undergoing proton beam therapy. It plays a critical role in refining the accuracy and effectiveness of cancer treatments. The simulation process provides insights that are vital for planning and executing individualized treatment plans. A precise simulation leads to better targeting of tumors, minimizing damage to surrounding healthy tissues.
Defining Proton Therapy
Proton therapy is a form of radiation treatment that utilizes protons rather than conventional x-rays to treat cancer. Protons are positively charged particles that, when delivered with precision, deposit a maximum dose of radiation directly at the tumor site while reducing exposure to adjacent healthy tissue. This ability to concentrate treatment on the tumor increases the likelihood of successful interventions in various types of cancers, particularly those located near sensitive structures, such as the brain and spinal cord. Understanding proton therapy's mechanics is crucial for developing effective treatment plans that can lead to improved patient outcomes.
Importance of Simulation in Treatment Planning
The simulation process is integral to the success of proton therapy. It involves creating a detailed, three-dimensional representation of the patient's anatomy using advanced imaging techniques. This data informs clinicians on how and where to deliver radiation accurately.
Accurate simulation can help in several ways:
- It aids in determining the correct angles and positions for proton beam delivery.
- It allows for the assessment of tumor location relative to critical anatomy to avoid damage during treatment.
- It enhances the personalization of treatment plans based on specific patient characteristics, which is necessary for optimizing therapeutic efficiency.
"Simulation acts as the cornerstone of effective treatment planning in proton therapy, bridging the gap between diagnosis and intervention."
The combination of imaging technologies such as CT and MRI allows for precise targeting of the tumor and better management of dose delivery. By thoroughly understanding the simulation process, healthcare providers can significantly improve therapeutic decisions and outcomes for their patients, ultimately enhancing the quality of cancer care.
The Technical Foundation of Proton Therapy Simulation
Understanding the technical foundation of proton therapy simulation is crucial. This section covers the basic principles that guide the practice of using protons in cancer treatment. Grasping these essentials improves our ability to harness proton therapy's potential effectively.
Understanding Particle Physics
To appreciate proton therapy simulation, one must first understand the nature of protons. Protons are positively charged particles found in an atom's nucleus. Their interactions with matter are complex and determined by basic physics principles.
Proton therapy leverages the unique properties of protons to treat cancer effectively. When protons enter the body, they deposit energy in a controlled way. This energy can destroy cancer cells with minimal damage to surrounding healthy tissues. The physics behind this is mainly due to the Bragg peak phenomenon. This refers to how protons lose energy progressively until they reach a certain point, where they deposit most of their energy. This benefit is why proton therapy is an attractive option compared to traditional photon therapy.
Mechanisms of Proton Interaction with Matter
The interaction between protons and matter occurs via various mechanisms. These include ionization, nuclear reactions, and energy deposition. Understanding these interactions is vital for optimizing treatment plans.
When protons traverse tissue, they cause ionization. This means they knock electrons from atoms, creating charged particles. The extent of ionization depends on the energy of the protons and the type of tissue they traverse. For example, dense tissue absorbs more energy. This phenomenon emphasizes the importance of customizing simulation models according to patient anatomy.
Three main mechanisms characterize proton interactions with matter:
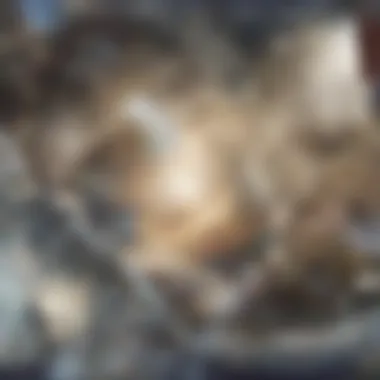
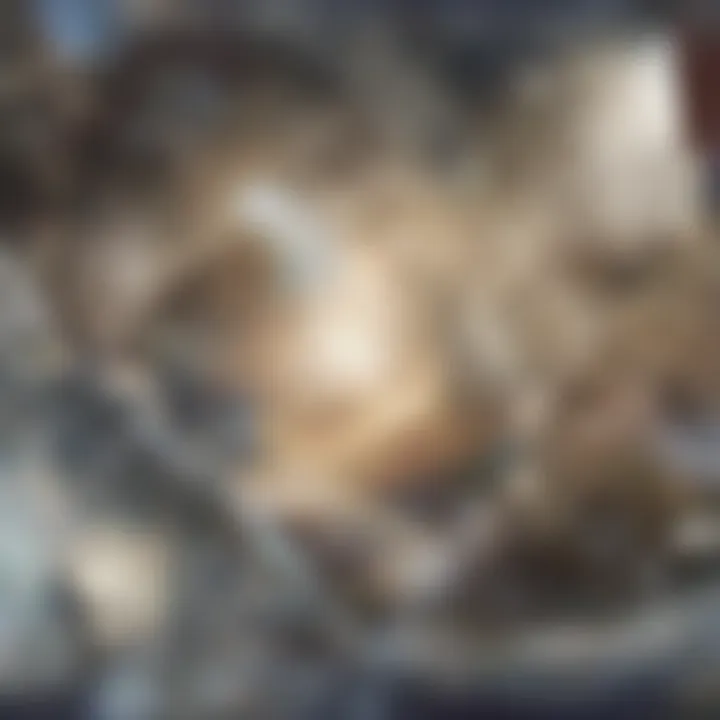
- Elastic scattering: Protons collide with nuclei, changing their direction without losing much energy.
- Inelastic scattering: Protons interact with nuclei, transferring energy and causing nuclear reactions. This is important for understanding radiation dose distributions.
- Nuclear reactions: High-energy protons can induce nuclear reactions, producing secondary particles that may contribute to the dose delivered to the tumor.
In summary, the technical foundation of proton therapy simulation rests on understanding particle physics and the specific interactions of protons with tissues. This knowledge is essential for creating precise treatment plans.
"The accuracy of proton therapy depends not just on the protons themselves but also on how well we simulate their interactions with matter."
Incorporating these principles into practical applications leads us to better utilize imaging modalities and treatment planning strategies mentioned later in this article. The precise control over dose distribution enhances the therapeutic ratio, making proton therapy an effective choice for various cancer types.
Simulation Methodologies and Techniques
Simulation methodologies and techniques are essential components in the realm of proton therapy simulation. These methodologies not only enhance the accuracy of treatment delivery but also improve overall patient safety and care efficiency. As proton therapy continues to gain traction as a preferred cancer treatment method, understanding the various simulation techniques becomes increasingly important.
One of the key benefits of these methodologies is the ability to create precise treatment plans tailored specifically to each patientβs unique anatomy and tumor characteristics. This individualization is crucial, as it ensures that the protons target the tumor effectively while sparing surrounding healthy tissues. Simulation methodologies thus play a vital role in optimizing treatment outcomes and minimizing adverse effects.
Another consideration is the continuous advancements in imaging techniques and software. Improvements in technology have made it easier to implement sophisticated modeling techniques, which help clinicians visualize anatomical structures in three dimensions. This capability significantly enhances the treatment planning phase, allowing for more informed decisions.
Overall, a thorough understanding of simulation methodologies and techniques is critical for any healthcare professional involved in proton therapy.
Patient-Specific Considerations in Proton Therapy Simulation
Patient-specific considerations play a crucial role in the effectiveness of proton therapy simulation. Understanding individual patient needs is essential for developing personalized treatment plans. Such plans enhance therapeutic outcomes, reduce side effects, and improve the overall patient experience. By focusing on anatomical variability and tailoring treatment strategies, providers can optimize the precision of proton therapy. This section will delve into these crucial aspects, highlighting the importance of individualization in treatment protocols.
Anatomical Variability and Its Implications
Anatomical variability refers to the differences in body structures among patients. These differences can significantly influence how proton beams interact with tissues during treatment. Variability can arise from factors such as age, body mass index, and the presence of comorbidities. For instance, children and adults might have different anatomical profiles, affecting where the proton dose is administered.
Accurate mapping of a patientβs anatomy through advanced imaging techniques is vital for effective proton therapy. Understanding these variations not only aids in accurately targeting tumors but also minimizes damage to surrounding healthy tissues. A better understanding of these aspects can lead to enhancements in treatment precision.
- Key considerations in anatomical variability:
- Patient age and development.
- Body fat distribution.
- Previous surgical interventions.
By acknowledging these variations, oncologists can develop more effective treatment plans that cater to the unique anatomy of each patient.
"Anatomical variability is a critical factor that must be accounted for in proton therapy simulations. Precision in targeting is directly related to the understanding of each patient's unique body structure."
Tailoring Treatment Plans to Individual Needs
Tailoring treatment plans to individual needs is fundamental in proton therapy. This customization involves adjusting various aspects of the treatment matrix based on the specific characteristics of each patient. Such personalized approaches often include calculating optimal proton dosages, determining beam angles, and identifying proper treatment schedules.
Accurate simulations facilitated by various imaging modalities, including CT and MRI scans, allow clinicians to assess tumor location and size with precision. These assessments aid in the formulation of a comprehensive treatment plan that reflects the unique situation of each patient. With the support of robust data analytics and simulation technologies, clinicians can make informed decisions about the most effective treatment strategy.
- Benefits of tailored treatment plans:
- Increased treatment efficacy.
- Reduced risk of side effects.
- Enhanced patient satisfaction and quality of life.
As researchers continue to investigate the effects of individualized treatment approaches, it is clear that precision in proton therapy simulation leads to more favorable outcomes for patients. More attention to these aspects can lead to significant improvements in cancer treatment methodologies.
Advancements in Proton Therapy Simulation Technology
Advancements in proton therapy simulation technology are critical in refining the overall treatment of cancer. As the demands of precision medicine grow, the sophistication of simulation tools has adapted to provide optimized treatment plans. These advancements enhance not only the efficacy of proton therapy but also ensure patient safety and comfort during treatment.
Innovation in Simulation Software
The innovation in simulation software has transformed the landscape of proton therapy. Modern software solutions incorporate sophisticated algorithms that can more accurately model how protons interact with tissues. These tools allow clinicians to simulate various treatment scenarios effectively.
Several key benefits of improved simulation software include:
- Increased Accuracy: Advanced algorithms in simulation software can minimize uncertainties in dose distribution. This ensures that tumor cells receive a lethal dose while sparing healthy tissues, making treatments more effective.
- User-Friendly Interfaces: Many of today's software solutions provide intuitive interfaces that simplify the planning process. Clinicians can adapt treatment plans quickly based on individual patient anatomy, streamlining workflow.
- Real-Time Updates: Continuous integration of data helps in making real-time adjustments. This ability is crucial in dynamic circumstances, such as changes in tumor size or patient movement during treatment.
As simulation software continues to advance, its integration with other emerging technologies, including artificial intelligence, is expected to further enhance treatment accuracy.
Emerging Technologies in Imaging
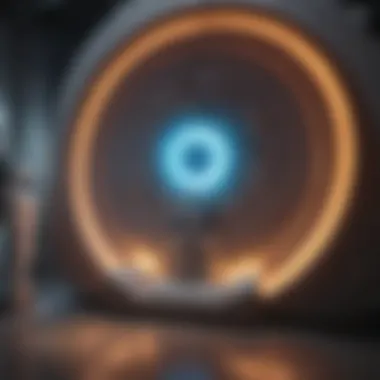
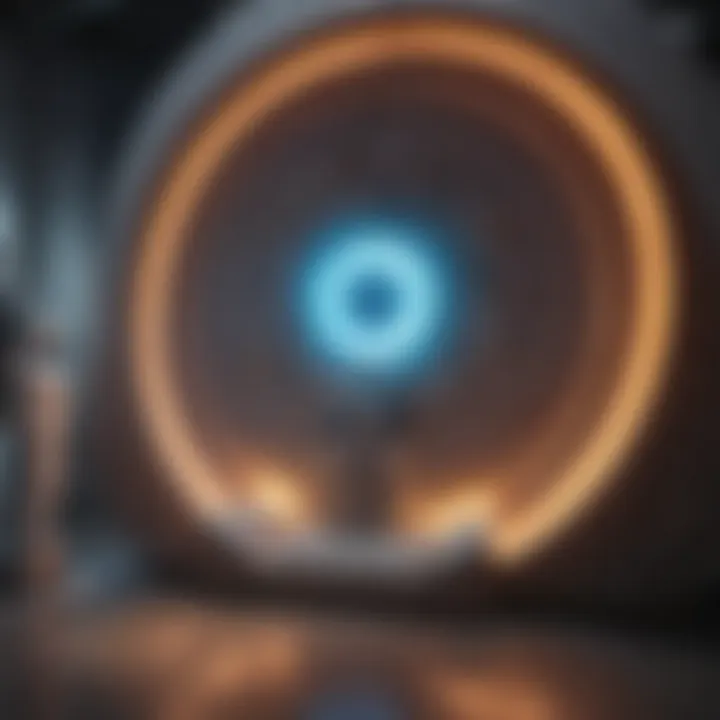
Emerging technologies in imaging play an essential role in enhancing proton therapy simulations. The convergence of imaging modalities and therapeutic planning systems has led to remarkable progress.
Imaging techniques such as MRI and advanced CT scanning have provided detailed anatomical information, which is key for precise targeting.
- MRI: Magnetic Resonance Imaging offers superior soft tissue contrast, making it invaluable in identifying tumor margins and surrounding structures. This capability is particularly relevant in complex cases such as brain tumors.
- Functional Imaging: Techniques like PET scans can assess metabolic activity, helping in the distinction between tumor and healthy tissue.
- Adaptive Imaging: Novel adaptive imaging approaches allow for treatment plans to be adjusted based on the most current patient anatomy. This adaptability is essential for maintaining accuracy as conditions evolve.
"The evolution of imaging technologies alongside simulation software is pivotal in advancing patient-specific treatments in proton therapy."
The Role of Machine Learning in Proton Therapy Simulation
Machine learning has emerged as a transformative force in various fields, including healthcare. In proton therapy simulation, its role is increasingly becoming critical. This section delves into how machine learning enhances treatment accuracy and improves predictive modeling in the context of proton therapy, ultimately aiming to optimize patient outcomes.
Enhancing Treatment Accuracy with AI Algorithms
Machine learning algorithms can analyze vast amounts of data quickly. In proton therapy simulation, this capability is particularly valuable. Algorithms can process imaging data from modalities such as CT and MRI to provide precise tumor localization. This is important because accurate tumor localization ensures that proton beams target the cancerous cells directly while sparing healthy tissues.
Additionally, machine learning can identify patterns in treatment response based on historical patient data. These algorithms can inform clinicians about the most effective treatment plans for individual patients, improving overall accuracy. This could potentially lead to better patient outcomes.
Benefits of using AI algorithms include:
- Improved accuracy in tumor localization
- Enhanced prediction of treatment responses
- Reduced chances of side effects from misdirected beams
- Efficient analysis of large datasets to derive actionable insights
Machine learning also assists physicians by streamlining workflows. Algorithms can automate parts of the treatment planning process, allowing oncologists to focus more on patient care rather than administrative tasks.
Predictive Modeling in Treatment Planning
Predictive modeling uses machine learning to simulate various treatment scenarios based on patient-specific data. By analyzing a patient's unique anatomical features, prior treatment history, and tumor characteristics, machine learning models can forecast the probable outcomes of different proton therapy plans. This approach is significant for tailoring interventions that align with individual patient needs.
Key aspects of predictive modeling include:
- Simulating multiple treatment approaches rapidly
- Providing quantifiable risk assessments for treatment options
- Forecasting long-term outcomes based on short-term responses
These insights allow clinicians to make informed decisions about the most effective treatment paths for their patients. Moreover, incorporating predictive modeling into treatment planning can enhance the personalization of care, making each therapy session uniquely aligned with the patientβs condition.
"Machine learning's predictive capabilities can change how clinicians approach proton therapy, focusing on precision rather than trial-and-error methods."
Regulatory and Standards Framework in Proton Therapy Simulation
The regulatory and standards framework in proton therapy simulation plays a critical role in ensuring safe and effective treatment delivery. It provides guidelines that direct the implementation of proton therapy within clinical practices. Adherence to these regulations not only lays a foundation for treatment consistency but also promotes patient safety and quality assurance.
Regulatory bodies, such as the Food and Drug Administration (FDA) in the United States, oversee the approval and monitoring of medical devices and treatment protocols used in proton therapy. These regulations help in minimizing risks associated with unintended radiation exposure and encourage the adoption of best practices across treatment centers.
A comprehensive regulatory framework includes the following key components:
- Clarity in Treatment Protocols: Regulations outline clear protocols for patient selection and treatment planning. This clarity enhances the precision of doses administered, ensuring that patients receive tailored therapy based on their specific needs.
- Training and Certification: Proper training and certification are mandated for practitioners. This ensures that professionals are well-versed in the complexity of proton therapy and simulation techniques, leading to improved patient outcomes.
- Benchmarking: Standards provide benchmarks for evaluating results from different treatment centers. This benchmarking facilitates continuous improvements in practices and technologies in proton therapy simulation.
As proton therapy continues to advance, these regulations adapt to integrate emerging technologies and methodologies. Keeping abreast of these regulatory updates is essential for professionals in the field who aim to provide the most effective and safe treatments.
Guidelines for Clinical Implementation
The guidelines set forth for the clinical implementation of proton therapy simulation aim to standardize practices and mitigate risks. These guidelines suggest protocols on:
- Patient Assessment: Comprehensive assessment of patient eligibility based on anatomical features, tumor characteristics, and overall health is crucial. This process must follow established criteria that ensure the appropriate use of proton therapy.
- Dose Calculation: Accurate dose calculations should follow standardized methodologies. This includes ensuring that treatment planning systems have been tested and validated under regulatory guidelines.
- Documentation: Complete and accurate documentation of each patient's treatment plan, simulation details, and outcomes is essential. This assists in maintaining continuity of care and supports quality assurance activities.
Using these guidelines, hospitals and clinics can formulate treatment plans that prioritize both efficacy and safety for patients undergoing proton therapy simulations.
Quality Assurance and Safety Protocols
Quality assurance and safety protocols are intrinsic to the successful application of proton therapy simulation. Their main objectives are to:
- Ensure Safety: Safety audits and checks are vital in monitoring the delivery of radiation. Safety protocols involve regular calibration and maintenance of equipment, ensuring that radiation doses administered align with prescribed standards.
- Monitor Outcomes: Quality assurance measures include systematic reviews of treatment outcomes. This involves collecting and analyzing data on patient responses, which can inform necessary adjustments to protocols.
- Continuous Education: Ongoing training and education for staff involved in proton therapy simulations reinforce safety standards. Regular workshops and updates on evolving practices contribute to a culture of safety and compliance.
Implementing robust quality assurance and safety protocols leads to improved patient confidence and treatment outcomes. The assurance that there are comprehensive measures in place to protect patient health reinforces the vital importance of these frameworks in proton therapy simulation.
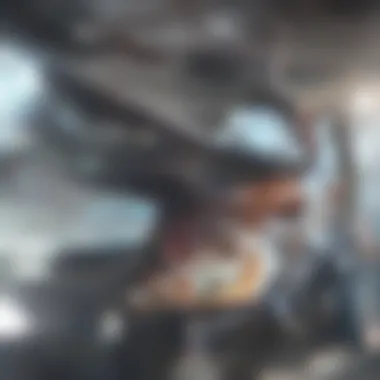
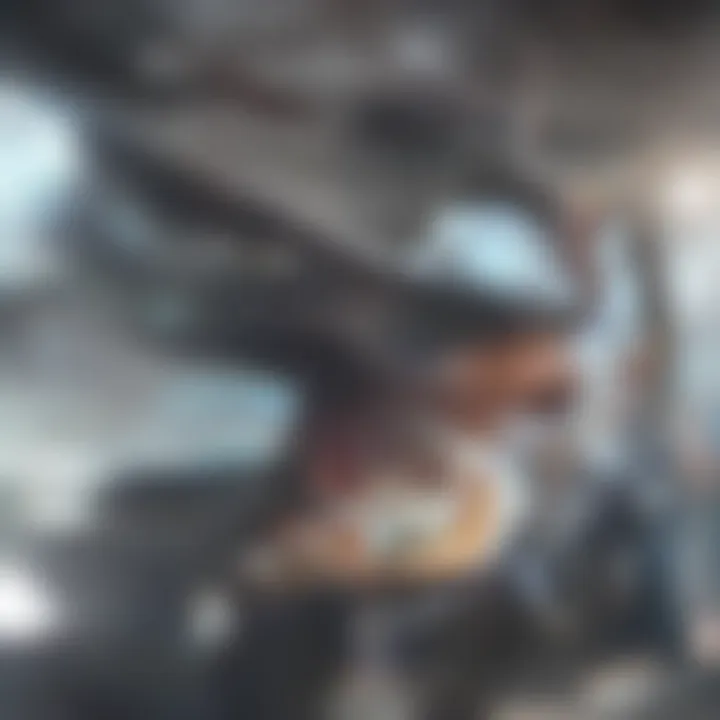
"Effective regulation in healthcare, particularly in innovative fields like proton therapy, is non-negotiable for patient safety and treatment efficacy."
Clinical Applications and Case Studies
Clinical applications of proton therapy simulation are essential in understanding how this technology impacts patient outcomes. The specificity and precision of proton therapy have led to numerous applications in different cancer types. It is vital to explore these applications to grasp the therapy's full potential and its role in modern oncological practices.
Proton Therapy for Pediatric Patients
Pediatric patients often present unique challenges in cancer treatment, largely due to their developing bodies and different disease presentation compared to adults. Proton therapy stands out as a particularly advantageous option for this demographic. The delicate nature of children's tissues makes them more susceptible to damage from conventional radiation therapy, which indiscriminately affects healthy surrounding areas. Proton therapy, with its ability to deposit most of its energy directly at the tumor site, minimizes this collateral damage.
Researchers have documented various cases that showcase the benefits of proton therapy for children. For instance, treating brain tumors with proton therapy has shown promising results, significantly reducing long-term cognitive side effects. As the survival rates improve, the focus on enhancing the quality of life becomes paramount.
The importance of accurate simulation in this context cannot be overstated. Customized treatment plans that account for the unique anatomical considerations of pediatric patients ensure optimal results. A thorough simulation process allows for precise planning and targeting, making it indispensable for this population.
Efficacy in Treating Specific Cancer Types
Various types of cancers benefit from proton therapy simulation, but certain malignancies demonstrate particularly compelling outcomes. For example, tumors located near critical structures, such as the spinal cord or optic nerves, are prime candidates for proton therapy. Prostate cancer and certain forms of head and neck cancer have become well-documented cases where proton therapy has rendered effective results while preserving surrounding healthy tissue.
The simulation process plays a key role in confirming the efficacy of proton therapy for these cancers. By utilizing advanced imaging techniques, oncologists can outline the tumor meticulously, thus enhancing targeting and improving treatment outcomes. Evidence suggests that patients undergoing proton therapy for prostate cancer experience fewer side effects compared to those receiving traditional radiation therapy.
Specific research studies indicate that in head and neck cancer, proton therapy can lead to a lower instance of xerostomia or dry mouth, which is a common side effect of standard radiation. By preserving salivary glands in treatment planning, proton therapy simulation takes advantage of its precision in targeting tumors while limiting damage to adjacent healthy structures.
In summary, the application of proton therapy simulation across various cancer types showcases its relative efficacy and flexibility. The insights drawn from clinical cases reinforce its potential in enhancing patient outcomes. By focusing on tailored treatment plans through rigorous simulation methodologies, the future of proton therapy appears promising for both pediatric and adult patients.
Challenges and Limitations of Proton Therapy Simulation
Proton therapy simulation is a vital component in the effective planning and delivery of cancer treatment. However, there are notable challenges and limitations that need to be addressed. Understanding these obstacles is crucial for researchers and healthcare professionals engaged in this field. Exploring these challenges can shed light on current research directions and necessary advancements in technology.
Technical Barriers in Simulation Accuracy
One key challenge in proton therapy simulation involves achieving high levels of accuracy. Technical barriers can often undermine the precision required for effective treatment planning. Factors such as image resolution, alignment protocols, and the effects of motion during patient treatment all contribute to the complexities involved. Deficiencies in imaging technologies, for example, can lead to misrepresentation of tumor geometry, which in turn affects dose distribution. This is particularly critical when treating tumors located near sensitive organs.
A significant element impacting simulation accuracy is the calibration of imaging devices. Regular maintenance and calibration ensure consistent performance. Furthermore, discrepancies between imaging results from different modalities, such as CT and MRI, pose another layer of complexity. Integrating these varied outputs requires sophisticated algorithms capable of accurately fusing data from multiple sources. Without these advancements, the reliability of treatment plans may suffer, ultimately affecting patient outcomes.
"Accurate simulation is necessary to deliver targeted therapy that minimizes damage to surrounding healthy tissue while maximizing treatment efficacy."
Patient Accessibility and Cost Implications
Another important aspect to consider is patient accessibility to proton therapy. The cost implications related to advanced proton therapy systems often limit the availability of such treatments. Proton therapy centers require significant financial investments for infrastructure, personnel training, and ongoing operational expenses. As a result, fewer facilities can offer proton therapy, leading to longer travel distances for patients seeking this advanced treatment option.
Additionally, the overall cost of proton therapy compared to conventional treatments can pose challenges. These include insurance coverage limitations and lack of patient awareness concerning treatment benefits. Due to these financial barriers, patients may be discouraged from pursuing proton therapy, regardless of its potential effectiveness for their condition.
- Key Considerations:
- High costs of initial investment for proton therapy facilities.
- Ongoing operational and maintenance costs.
- Insurance coverage variability.
- Need for patient education and awareness programs.
Ultimately, addressing these challenges is essential for the continued development of proton therapy simulation and ensuring it becomes more widely accessible. The integration of streamlined processes and cost-effective solutions may lead to better patient outcomes in the future.
Future Perspectives in Proton Therapy Simulation
The future of proton therapy simulation is marked by an exciting confluence of research, technological advancement, and clinical application. This section focuses on understanding the trends and integrating proton therapy with other treatment modalities. By examining these perspectives, we can better comprehend how future developments may enhance treatment efficacy and patient outcomes.
Trends in Research and Development
Research is continuously evolving in the field of proton therapy simulation. A significant trend is the incorporation of advanced imaging techniques. These innovations allow for a more precise delineation of tumor boundaries. Improved imaging supports higher accuracy in proton beam delivery which is critical for sparing healthy tissues.
Moreover, artificial intelligence is gaining traction. AI algorithms are being developed to enhance treatment planning. This development not only streamlines processes but also allows for predictive modeling that assesses treatment responses. Machine learning is a robust tool that analyzes vast datasets, facilitating personalized treatment strategies.
Collaboration across institutions is also a vital trend. This interdisciplinary approach fosters sharing of knowledge and resources, pushing the boundaries of what is achievable in proton therapy simulation. The emphasis on clinical trials is important. These studies breed a deeper understanding of proton therapy applications and contribute to the body of evidence supporting its use.
Integrating Proton Therapy with Other Modalities
The integration of proton therapy with other treatment modalities promises a more holistic approach to cancer treatment. One critical integration is the combination of proton therapy with immunotherapy. This partnership has potential benefits according to preliminary studies. The synergy may enhance the bodyβs capability to fight cancer while minimizing the side effects typically associated with traditional therapies.
Another notable integration is with chemotherapy. Combining proton therapy with systemic therapies may improve treatment responses. This strategy allows for targeted radiation while providing a broader therapeutic effect on circulating cancer cells.
Furthermore, the combination of proton therapy with conventional radiotherapy models can be promising. Utilizing both methods may maximize tumor control while reducing the risk of radiation-induced complications. \n
"The future is not something we enter; the future is something we create." β Leonard I. Sweet