Exploring Quantum Computing Trends: A New Era
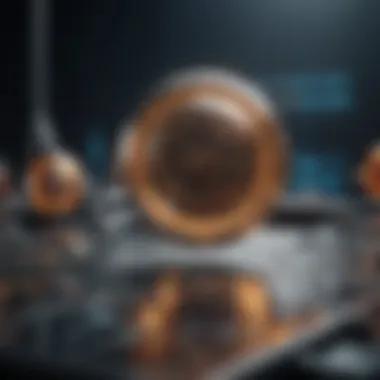
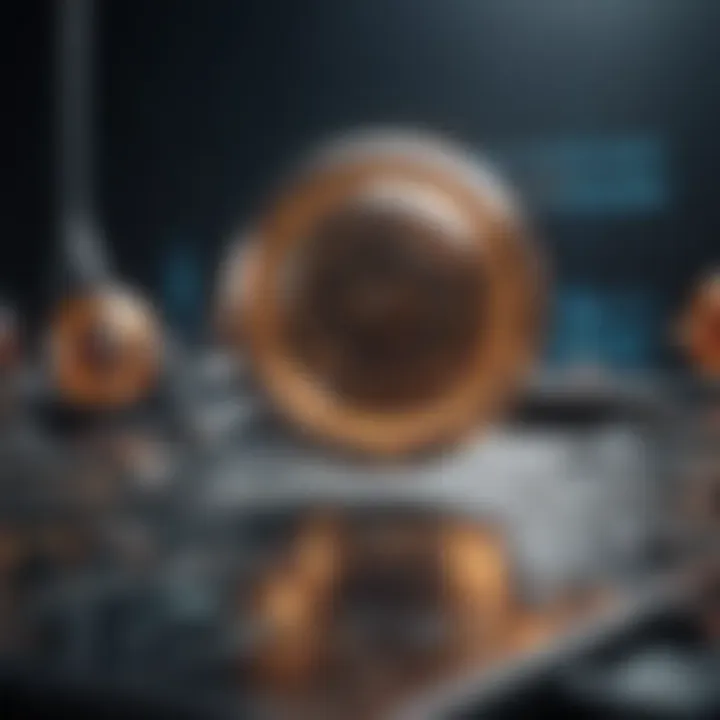
Intro
The field of quantum computing continues to evolve. Recent advancements create new opportunities in scientific research and technology. As the industry grows, understanding its trends becomes crucial for students, researchers, educators, and professionals alike. This article aims to synthesize knowledge around quantum computing, especially focusing on hardware, algorithms, and current applications.
Research Overview
Key Findings
The latest research in quantum computing shows several important developments. Key findings suggest:
- Increased fidelity in quantum bits, known as qubits.
- The emergence of quantum supremacy claims by companies like Google and IBM.
- Advancements in quantum error correction methods.
- A surge in quantum applications across sectors such as cryptography, materials science, and drug discovery.
These findings indicate a growing maturity of the field, pushing boundaries further. Quantum computing is now transitioning from theoretical studies to technological application.
Study Methodology
To analyze the current trends in quantum computing, a multi-faceted approach was utilized. This includes:
- Literature Review: Recent academic papers, journals, and articles were examined to identify key developments.
- Interviews: Insights were gathered from leading experts in quantum computing.
- Industry Analysis: Studies tracking investments and progress in the quantum tech industry were reviewed.
This methodology provides a holistic understanding of the landscape of quantum technologies.
Background and Context
Historical Background
Quantum computing began in the late 20th century. The theoretical foundations were laid by pioneers such as Richard Feynman and David Deutsch. They highlighted the limitations of classical computers when dealing with complex quantum phenomena. Over the years, various models and algorithms emerged, leading to the first practical demonstrations of quantum algorithms.
Current Trends in the Field
Currently, several trends are shaping the landscape of quantum computing:
- Quantum Hardware Development: Enhanced qubit designs and new materials such as superconductors and trapped ions.
- Algorithm Improvement: More efficient quantum algorithms that optimize performance.
- Interdisciplinary Collaboration: Interactions between quantum computing and fields like artificial intelligence and machine learning.
Intro to Quantum Computing
Quantum computing represents a paradigm shift in computational capabilities. Its significance lies in its potential to solve complex problems that classical computers cannot address efficiently. For researchers and professionals, understanding quantum computing is crucial due to its implications across multiple scientific fields, including physics, chemistry, and computer science. Furthermore, as technological advancements progress, recognizing quantum computing's evolution helps in appreciating its capabilities and applications.
Defining Quantum Computing
Quantum computing utilizes the principles of quantum mechanics to store and process information. Unlike classical bits, which exist in a binary state (0 or 1), quantum bits—often referred to as qubits—can exist in multiple states simultaneously. This property, known as superposition, allows quantum computers to execute many calculations in parallel, vastly increasing their computational power.
Moreover, quantum entanglement is another critical feature. When qubits become entangled, the state of one qubit is directly related to the state of another, no matter their distance apart. This interconnectedness further enhances the processing speed and capabilities of quantum systems. In practical terms, this means that quantum computers can tackle certain problems, such as factoring large numbers or simulating molecular structures, much more efficiently than classical counterparts.
The Evolution of Quantum Technologies
The emergence of quantum computing technology dates back several decades. Initial theoretical frameworks began to take shape in the 1980s, with pioneers like Richard Feynman and David Deutsch laying the groundwork for quantum theory applications in computation. Fast forward to the 21st century, we have witnessed an influx of funding and research focused on realizing quantum devices.
Key milestones in the evolution include:
- The development of quantum algorithms, such as Shor's and Grover's, which demonstrate potential computational advantages.
- Advances in quantum hardware, particularly in the realms of superconducting qubits and trapped ions, which serve as foundational components for quantum systems.
- Increased collaboration between academic institutions and industry, fostering innovation and practical applications.
As technology advances, the path of quantum computing continues to unfold, making it imperative for professionals in the field to stay informed about ongoing developments.
Recent Advancements in Quantum Hardware
Recent advancements in quantum hardware mark a pivotal shift in the realm of quantum computing. As researchers strive to create systems capable of performing complex calculations, developments in hardware play a crucial role. These innovations pave the way for practical applications and demonstrate the feasibility of quantum solutions to problems that classical computers struggle with. Understanding these advancements is essential for grasping the potential impact of quantum technology on various sectors.
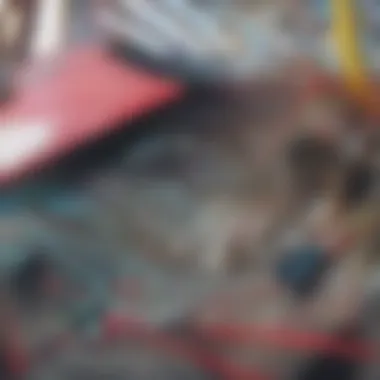
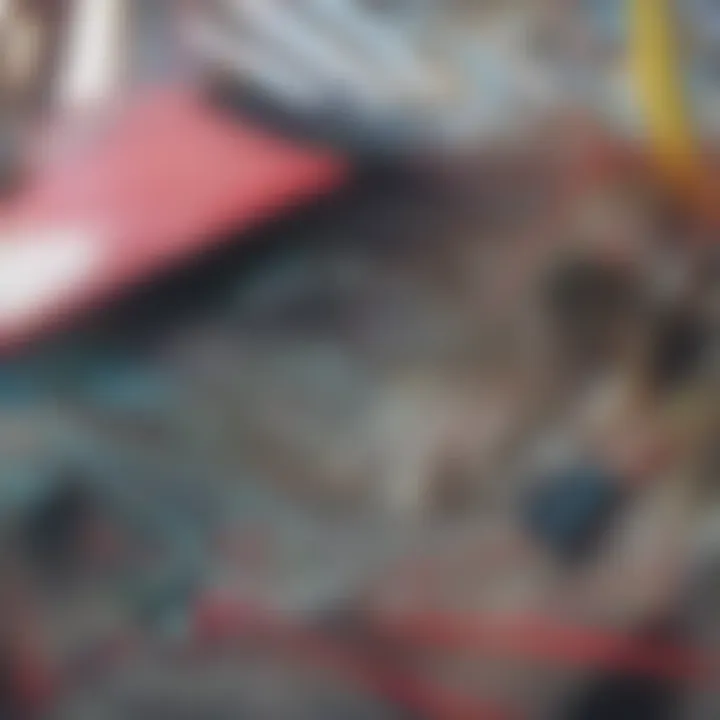
Superconducting Qubits
Superconducting qubits have emerged as one of the leading technologies in quantum computing. They leverage superconductivity to perform quantum computations at incredibly low temperatures, enabling faster processing speeds and enhanced coherence times. The advantage of superconducting qubits lies in their scalability, which can support larger qubit arrays crucial for solving complex problems.
A notable example is the IBM Quantum Hummingbird processor, featuring 65 superconducting qubits. The architecture of superconducting qubits allows for intricate gate designs that facilitate precise control of quantum states.
Trapped Ion Quantum Computers
Trapped ion quantum computers utilize charged atoms trapped by electromagnetic fields. This method enables high levels of precision in manipulating qubits, as light interacts with the ions to perform operations. The coherence times of trapped ion systems are exceptionally long, which reduces the rate of errors during computations.
Companies like IonQ and Honeywell have showcased the potential of trapped ion systems. Their designs incorporate advanced error correction techniques, making these systems reliable for both research and commercial use.
"Trapped ion systems offer some of the highest fidelity qubit operations available, making them a leading choice in quantum hardware research."
Topological Quantum Computing
Topological quantum computing represents an innovative approach designed to overcome some limiting factors of conventional qubit designs. This method relies on anyons, quasiparticles that exist in two-dimensional spaces. The information processed in topological quantum computers is stored in the braids of these particles, making it less susceptible to environmental noise and errors.
Though still largely experimental, research led by institutions such as Microsoft demonstrates promising progress in developing topological qubits. The unique nature of this technology could lead to significant advancements in fault-tolerant quantum computing.
In summary, these three advancements in quantum hardware demonstrate a spectrum of capabilities and potential in quantum computing. Their ongoing development and integration stand to enhance the efficiency and reliability of quantum systems, signaling a promising future for the technology.
Key Algorithms Driving Quantum Computing
The advancements in quantum computing heavily rely on specific algorithms designed to leverage quantum mechanics. These algorithms are crucial because they enable quantum computers to perform calculations that are practically impossible for classical computers. Understanding the functions and applications of these algorithms is essential to grasp the full potential of quantum computing. This section examines three key algorithms: Shor's Algorithm, Grover's Algorithm, and Quantum Simulation Algorithms, which are instrumental in various fields such as cryptography, database search, and scientific modeling.
Shor's Algorithm
Shor's Algorithm is notable for its ability to factor large integers efficiently. This capability poses a significant challenge to traditional encryption methods, particularly RSA (Rivest–Shamir–Adleman) cryptography, which relies on the difficulty of factoring large numbers. The algorithm demonstrates an exponential speedup compared to classical factoring methods, making it a central topic in discussions about quantum security.
To illustrate, the classical algorithms take polynomial time relative to the number of digits in the integer. However, Shor's Algorithm operates in polynomial time. The implications of this are profound, as it could render current cryptographic techniques obsolete, pushing the need for quantum-resistant encryption solutions.
"Shor's Algorithm revolutionizes the way we think about encryption and security. Its implications reach far beyond computing."
Grover's Algorithm
Grover's Algorithm addresses the problem of unstructured search. In classical computing, searching through an unsorted database of N elements requires, on average, N/2 queries. Conversely, Grover's Algorithm reduces this to about √N queries, showcasing a quadratic speedup. This is particularly relevant for applications where quick data retrieval is essential, such as in optimization tasks and cryptographic key searches.
The algorithm is effective and can provide superior solutions for numerous practical problems, offering a fast alternative to classical methods. As organizations increasingly depend on data-driven insights, Grover's Algorithm becomes a critical tool in the quest for efficiency in data search and retrieval.
Quantum Simulation Algorithms
Quantum Simulation Algorithms facilitate the modeling of quantum systems, a task that is often beyond the capabilities of classical computers. These algorithms can simulate physical phenomena accurately, leading to breakthroughs in fields like materials science and drug discovery. The capacity to model complex quantum interactions enables researchers to explore new materials and chemical reactions that were previously unfeasible to study.
For instance, simulating the behavior of electrons within a new material can help scientists discover potentially groundbreaking substances for energy storage or electronics. Quantum simulations pave the way for innovations across numerous domains, ultimately driving scientific inquiry and industrial progress.
In summary, the algorithms detailed here exhibit the transformative nature of quantum computing. Shor's and Grover's algorithms not only highlight potential threats and opportunities in security and data processing but also showcase the vast capabilities of quantum simulations in expanding our understanding of the natural world.
Applications of Quantum Computing
The significance of applications of quantum computing lies in their potential to radically change various industries. As advancements in the field emerge, the implications extend across medicine, security, and optimization. Researchers and businesses can leverage quantum computing's unique capabilities to solve complex problems that classical computers struggle to address. This section explores how quantum computing is not just a theoretical pursuit but is carving a path to practical, impactful applications.
Pharmaceuticals and Drug Discovery
Quantum computing shows promise in speeding up the drug discovery process. Traditional methods in pharmaceuticals often involve lengthy simulations and trials. Quantum algorithms can model molecular interactions at unprecedented speed and accuracy. This capability allows for more efficient screening of potential drug candidates.
- Enhanced Molecular Simulations:
- Reducing Development Time:
- Quantum simulations can explore different molecular configurations.
- They can calculate the energy states of molecules more precisely.

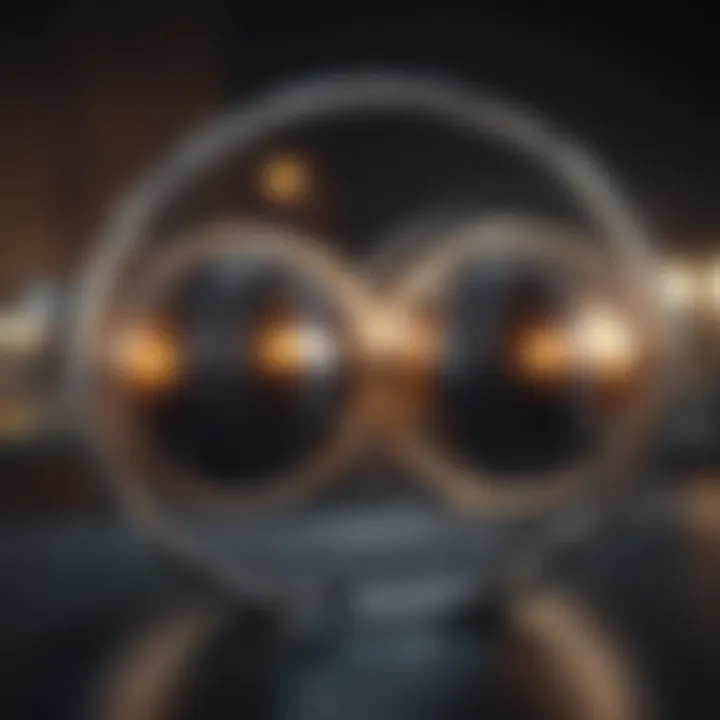
- With better modeling, researchers can focus on promising candidates earlier.
- This can significantly reduce the time taken from discovery to market.
"Quantum computing has the potential to revolutionize how we discover new drugs, enabling us to understand complex biological processes at a quantum level."
Availing benefits from quantum computing can lead to breakthroughs in treatments for diseases that are currently difficult to target. Thus, this technology holds immense promise for the future of pharmaceuticals.
Cryptography and Information Security
Another critical application of quantum computing is in the realm of cryptography. As data security is becoming increasingly vital, quantum technology can address some of the limitations of classical cryptographic methods. Quantum Key Distribution (QKD) is a notable example of how quantum systems enhance security protocols.
- Quantum Key Distribution:
- Quantum Resistance Algorithms:
- It ensures secure transmission of keys using quantum mechanics.
- Eavesdropping can be detected in real-time, raising security standards.
- As quantum computers advance, they could threaten traditional encryption algorithms.
- Developing quantum-resistant encryption is crucial for future-proofing data security.
The implications for national security, finance, and personal data protection are profound. The interplay between quantum computing and cryptography is an evolving landscape that necessitates ongoing research and development.
Optimization Problems in Logistics
Logistics and supply chain management face numerous challenges, including route optimization, resource allocation, and inventory management. Quantum computing provides sophisticated mechanisms to navigate these issues more effectively than existing classical methods.
- Route Optimization:
- Resource Allocation:
- Inventory Management:
- Quantum algorithms, such as the Quantum Approximate Optimization Algorithm (QAOA), can efficiently calculate the best routes for delivery services.
- Improved routing can reduce costs and delivery times significantly.
- Businesses can effectively allocate resources based on dynamic market conditions and demand forecasts.
- This adaptability can lead to optimized production schedules.
- Quantum computing can enhance demand prediction models, making inventory management more precise.
- Reducing excess stock lowers waste and increases profitability.
The incorporation of quantum computing into logistics can create substantial efficiencies and lead to more responsive and agile supply chain operations.
Interdisciplinary Collaborations in Quantum Research
Interdisciplinary collaborations are essential in quantum research. They merge diverse fields, bringing fresh perspectives and solutions to complex issues. Researchers from physics, computer science, neuroscience, and material science can work together, facilitating the exchange of ideas. This collaboration enhances the scientific community’s understanding of quantum systems and accelerates advancements in technology.
The benefits of such partnerships are numerous. They allow for the combination of theoretical knowledge with practical applications. By working together, scientists can develop new quantum algorithms, enhance system stability, and improve error correction methods. The shared goal within interdisciplinary teams is often to push the boundaries of what quantum computing can achieve.
Furthermore, interdisciplinary research encourages innovation. It allows researchers to explore unknown territories. For instance, combining quantum theories with insights from neuroscience can lead to the creation of more efficient algorithms, mimicking how the human brain processes information.
Additionally, these collaborations often lead to breakthroughs that would be difficult for a single field to achieve alone. They allow researchers to ask better questions and pursue ambitious projects. By pooling expertise, teams can tackle challenges that include scalability and error rates more effectively than if they were to work in isolation.
The collaborative atmosphere fosters creativity. It invites thinkers to question standard methods and approach problems with novel strategies, essential in a field marked by rapid change and complex challenges.
Physics and Computational Neuroscience
The relationship between physics and computational neuroscience highlights a particularly fruitful area of interdisciplinary research. Physics, with its focus on the fundamental laws of nature, provides the groundwork necessary for advancements in quantum computation. Computational neuroscience, on the other hand, offers insights into how the brain processes information.
Researchers are exploring how principles found in physics can explain neural activities. This understanding might inform the design of quantum computers. For instance, neural models inspired by brain functions can lead to improved quantum algorithms that enhance speed and efficiency. The potential to mimic biological processes within quantum frameworks paves the way for revolutionary developments.
Moreover, simulations that replicate brain activities can allow scientists to not only forecast but also analyze quantum behaviors. By employing methods used in neuroscience, researchers can refine quantum error correction, ensuring more stable and reliable computations.
Quantum Computing in Artificial Intelligence
The intersection between quantum computing and artificial intelligence (AI) is another area of intense focus. Quantum computing holds the promise of transforming AI by significantly speeding up data processing. Its ability to handle vast data sets through quantum superposition and entanglement allows for more sophisticated machine learning algorithms.
AI can also benefit from enhanced optimization techniques offered by quantum algorithms. Tasks such as identifying patterns and making predictions can be completed faster and at a scale impossible for classical computers. This acceleration enables the development of more powerful AI systems capable of solving complex problems across various domains.
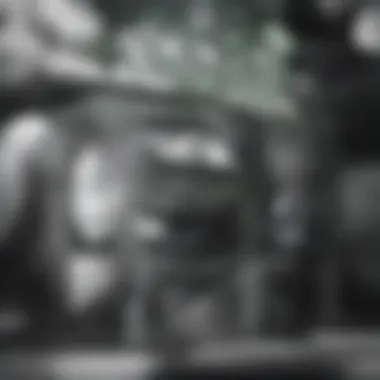
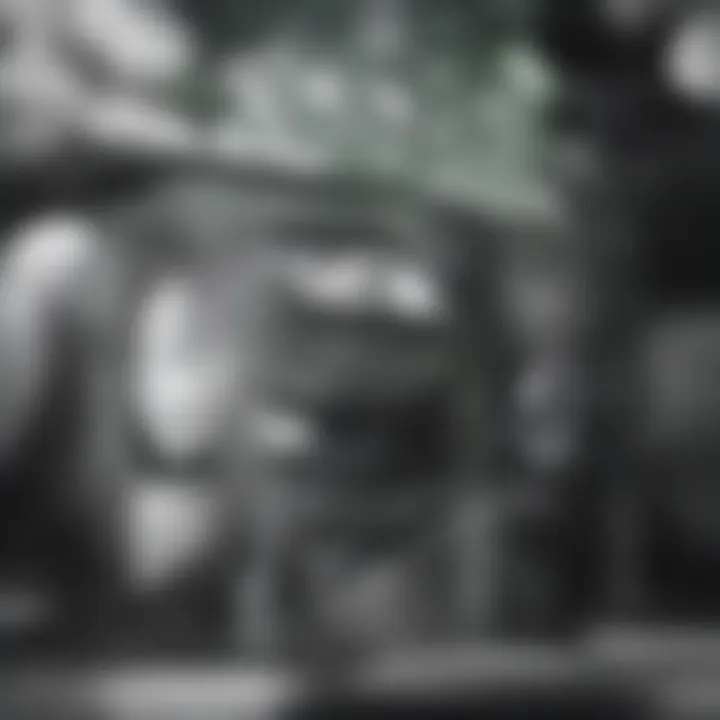
AI's capabilities, when combined with quantum advancements, can revolutionize industries. For example, in drug discovery, sophisticated models can analyze chemical compounds more effectively, leading to quicker innovations in pharmaceutical development.
"The fusion of quantum computing and artificial intelligence is set to redefine the boundaries of technology, offering unprecedented speed and efficiency in problem-solving."
As researchers explore these overlapping domains, they uncover new applications. Enhanced AI systems could lead to breakthroughs in natural language processing, data analysis, and autonomous systems, enriching both sectors.
Challenges in Quantum Computing
The realm of quantum computing, while promising, is fraught with significant challenges. These hurdles impede the realization of fully operational quantum systems. Understanding these challenges is crucial as they shape the development path of this technology. The primary focus of this section is to elaborate on decoherence, error correction, and scalability concerns. The insights gained will assist researchers and practitioners in navigating both the theoretical and practical implications of quantum computing.
Decoherence and Error Correction
Decoherence refers to the loss of quantum coherence, where qubits lose their quantum properties due to environmental interference. This effect represents a significant barrier to maintaining reliable quantum states. Environmental factors such as temperature fluctuations and electromagnetic radiation can lead to errors in computations, undermining the potential advantages of quantum systems.
Strategies for error correction in quantum computing are essential. These techniques are designed to identify and mitigate errors caused by decoherence. Quantum error correction codes, like the Shor code and the Surface code, are developed to protect quantum information even when errors occur. These codes rely on redundant encoding of qubits to ensure that information can be retrieved accurately despite the presence of errors.
"Decoherence is a challenge that necessitates robust error correction methods to ensure quantum states remain stable over time."
By employing error correction techniques, researchers aim to enhance the resilience of quantum computations. However, these methods introduce additional complexities. Implementing effective error correction requires extra resources, complicating the design and functioning of quantum systems. The delicate balance between maintaining coherence and managing error rates remains a focal point of research.
Scalability Concerns of Quantum Systems
Scalability is another critical challenge in the field of quantum computing. As researchers work toward building larger and more complex quantum systems, they encounter multiple issues. One concern is the interconnectivity of qubits. For quantum systems to perform advanced calculations, qubits must interact efficiently with one another. This process is not straightforward due to limitations in current technology and design.
Moreover, scaling up quantum devices often leads to increased error rates and decoherence. The larger the system, the greater the number of qubits, which means more potential points of failure. Additionally, significant resources are needed to maintain the required low temperatures and other conditions for optimal performance.
The scalability dilemma also involves economic feasibility. Developing and maintaining large-scale quantum computers demands significant financial investment. Partnerships between academic institutions and industry leaders can address some of these issues by pooling resources and knowledge.
In summary, while the prospects of quantum computing are enticing, the challenges of decoherence, error correction, and scalability pose significant tests for researchers. A systematic approach to overcoming these barriers will require innovation, collaboration, and persistence in efforts to realize the full potential of quantum technology.
Future Prospects of Quantum Computing
Understanding the future prospects of quantum computing is crucial for several reasons. As we stand on the brink of a technological revolution, the implications of quantum advancements could redefine various fields of study and industries. The promise of quantum computing extends beyond mere speed. It offers the potential to solve problems considered intractable for classical computers. Therefore, this section explores the fundamental aspects of quantum supremacy and market impact, shedding light on the transformative power of this emerging technology.
The Role of Quantum Supremacy
Quantum supremacy refers to the point at which quantum computers can perform calculations that are infeasible for classical computers. This milestone is significant because it marks the transition from theory to practical applications. In 2019, Google claimed to have achieved this feat with its quantum processor, Sycamore. Research has shown that quantum systems excel in specific tasks such as optimization, simulation, and cryptography.
The importance of quantum supremacy extends to various fields. For instance, in pharmaceuticals, researchers can simulate molecular interactions faster, potentially shortening drug discovery timelines. Similarly, industries focusing on optimization, like logistics and supply chain management, stand to benefit immensely. As quantum computers become more reliable, their ability to influence decision-making processes will likely enhance operational efficiencies and drive innovation.
"Quantum supremacy represents a pivotal moment in the advancement of computational capabilities, reshaping our approach to complex problem-solving."
Potential Market Impact and Economic Implications
The market impact of quantum computing is multi-faceted. The technology promises to disrupt numerous sectors, leading to substantial economic implications. According to various estimates, the quantum computing market could be worth billions by the end of this decade. This potential growth is driven by both advancements in hardware and an increasing understanding of how to leverage quantum algorithms.
In financial services, quantum computing could transform risk analysis and fraud detection. Its ability to process vast amounts of data in real-time offers significant advantages over classical methods. Moreover, sectors such as cybersecurity will need to adapt to quantum-resistant algorithms, as current encryption methods may become obsolete.
In summary, the future of quantum computing is promising. As research continues and market dynamics shift, both existing and new industries must prepare for fundamental changes in their operational frameworks. This readiness will determine their competitive edge in an increasingly quantum-driven world.
Finale
The exploration of quantum computing signifies a pivotal moment in technological advancement. This article aimed to encapsulate the current state of quantum technologies, emphasizing their multifaceted impact across various disciplines. Understanding quantum computing is not just about grasping the underlying principles but recognizing how these developments challenge and redefine existing paradigms in computation and data processing.
Summarizing the Current Landscape
In summary, the landscape of quantum computing is rapidly evolving. Recent advancements in superconducting qubits, trapped ions, and topological systems contribute to a broader understanding of quantum mechanics and its applicability in real-world contexts. The emergence of algorithms like Shor's and Grover's offers novel solutions to previously intractable problems, highlighting the transformative potential of quantum technology.
Additionally, applications ranging from pharmaceuticals and logistics optimization to cryptography reflect the diverse fields poised to benefit from these innovations. The interplay between research disciplines fosters a collaborative environment, where physicists, computer scientists, and engineers converge. This cross-pollination of ideas is essential as researchers tackle the challenges of decoherence and error correction, ensuring the scalability of quantum systems for practical use.
Implications for Future Scientific Research
The implications of quantum computing extend beyond immediate technological applications. As researchers continue to unravel the complexities of quantum systems, the potential for breakthroughs in scientific research increases significantly. This could lead to enhanced computational models in fields like AI, materials science, and complex systems analysis.
Moreover, by navigating the challenges and exploring the frontiers of quantum computing, researchers are not only advancing their fields but also addressing critical global issues. The realization of quantum advantage could result in solutions to pressing challenges in climate modeling, supply chain logistics, and healthcare optimization.