Understanding Cellular Architecture: Key Components and Functions
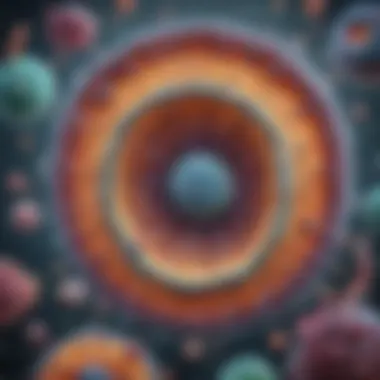
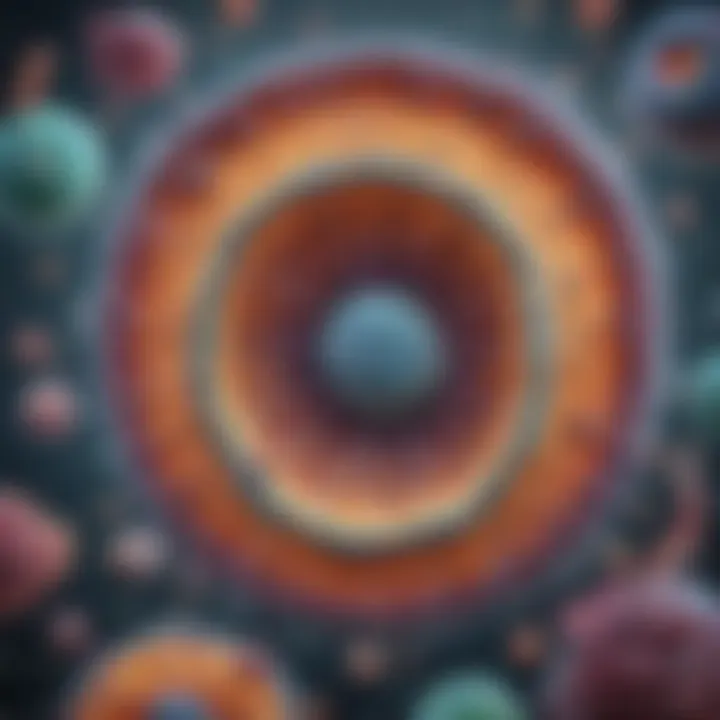
Intro
The study of cells is like opening a window into the very fabric of life itself. Every organism, from the tiniest bacterium to towering redwoods, if viewed through a microscope, reveals a remarkable and complex architecture that operates tirelessly. Cells, once thought to be simple units of life, are, in fact, highly organized structures with distinct parts that carry out essential functions. Understanding this cellular architecture not only sheds light on biological processes but also lays the groundwork for advances in medicine, biotechnology, and environmental science.
In this article, we will delve into the various components of cells, both prokaryotic and eukaryotic. Each section seeks to unravel the intricate interactions and roles that these structures perform, aiming to provide readers with a profound insight into cellular biology. By systematically examining components such as the plasma membrane, nucleus, organelles, and cytoplasm, we hope to promote a deeper appreciation of how life is built and sustained at the cellular level.
Prelude to Cellular Biology
Cellular biology stands as a fundamental pillar in the realm of life sciences. Understanding how cells, the basic units of life, function, interact, and adapt is paramount to deciphering the complexities of biological systems. At its core, cellular biology helps illuminate the intricate workings of organisms, from the meticulously ordered structure of prokaryotic cells, which lack a defined nucleus, to the complex orchestration found in eukaryotic cells, where a multitude of organelles work symbiotically.
In this article, we will shed light on the various components within cells and their respective functions. This exploration is not just academic; it encompasses real-world applications, from medical advancements to environmental science, where knowledge of cell biology can lead to breakthroughs.
By examining the subtleties of cellular architecture, we pave the way for deeper insights into topics like genetics, biochemistry, and evolutionary biology. Moreover, an appreciation for the differences in cell types can aid in comprehending how organisms adapt to their environments, providing a clarity that transcends mere memorization. As we dive into the specifics of cellular structures and the roles they play within both prokaryotic and eukaryotic contexts, we take significant steps toward a holistic understanding of life's fundamental unit.
The Significance of Cells in Life
Cells are often dubbed the "building blocks of life." This description bears its weight—without cells, there would be no tissues, no organs, and ultimately, no organisms. Each cell can function independently yet also coordinate with its neighbors, contributing to the synergistic functions necessary for survival. Whether it is the ability to proliferate, respond to stimuli, or assimilate nutrients, cells encapsulate the essence of life itself.
Cells carry out a plethora of functions that are essential for both individual organisms and entire ecosystems. They communicate using chemical signals, defend against pathogens, and recycle waste materials, ensuring that the vital processes of life continue unabated. In terms of evolutionary significance, understanding cellular mechanisms has shaped our approaches in fields such as medicine, biotechnology, and conservation.
"The cell is the basic unit of life, and understanding its architecture is crucial for advancing our knowledge in numerous biological disciplines."
Overview of Prokaryotic and Eukaryotic Cells
Contrasting the two primary classifications of cells—prokaryotic and eukaryotic—offers profound insights into biological diversity. Prokaryotic cells, those that make up bacteria and archaea, are characterized by their simplicity. They typically possess no membrane-bound organelles, with the genetic material residing freely in the cell's cytoplasm. Their rapid reproduction capabilities and adaptability to harsh environments make them intriguing subjects for study.
In stark contrast, eukaryotic cells are the more complex entities found in animals, plants, fungi, and protists. They feature distinct organelles such as the nucleus and mitochondria, each playing specialized roles. The compartmentalization of eukaryotic cells allows for more sophisticated regulation of cellular functions, contributing to the complexity seen in multicellular organisms.
This comparison between cell types does not merely reflect anatomical differences; it underscores functional ramifications that influence growth patterns, metabolic processes, and even the evolution of life as we know it. By grasping the unique features of prokaryotic and eukaryotic cells, we lay the groundwork for further exploration into how these cells interact with each other and their environments, thereby enriching our comprehension of cellular biology.
Cell Membrane: The Protective Barrier
The cell membrane, often referred to as the plasma membrane, serves as the crucial protective barrier that encapsulates the cell's contents. Its importance in cellular biology can’t be overstated. This membrane not only defines the boundaries of the cell but also plays a pivotal role in maintaining homeostasis, allowing essential materials to enter and exit while keeping damaging substances at bay. Considering it a mere barrier would be an understatement; it is a dynamic structure that is constantly engaged in various interactions with its environment.
Structure of the Cell Membrane
The structure of the cell membrane can be primarily described using the fluid mosaic model. This model conceptualizes the membrane as a mosaic of diverse protein molecules embedded in a phospholipid bilayer. The phospholipids have hydrophilic (water-attracting) heads and hydrophobic (water-repelling) tails. This duality enables the formation of a semi-permeable barrier, where the aligned tails meet and the heads face the watery environments both inside and outside the cell.
Key features of this structure include:
- Phospholipid Bilayer: The fundamental framework that creates a barrier between the cell's internal and external environments.
- Proteins: Integral and peripheral proteins that facilitate communication, transport, and enzymatic activity.
- Cholesterol: Interspersed within the bilayer, cholesterol molecules help to stabilize the membrane’s fluidity, making it less permeable to very small water-soluble molecules.
Functions of the Cell Membrane
The cell membrane has several key functions that ensure cellular integrity and communication.
Selective Permeability
Selective permeability is one of the cell membrane’s standout characteristics. It refers to the ability of the membrane to regulate what substances can pass through. This ensures that essential nutrients such as glucose and ions can enter the cell, while waste products and harmful substances are kept out. The efficiency of this process is paramount for maintaining the cell's internal environment.
Advantages of selective permeability include:
- Nutrient uptake: The ability to allow necessary molecules through plays a direct role in metabolism.
- Waste management: It prevents toxic substances from accumulating, thus safeguarding cell function.
However, there are some disadvantages as well:
- Limitations on Transport: Not all molecules can pass freely; larger or charged molecules often require specific transport proteins to assist their movement.
Cell Communication
Cell communication through the cell membrane is vital for tissues and organ systems to function cohesively. Receptors on the cell membrane are crucial for detecting signals from hormones and neurotransmitters. When these signals bind to receptors, a cascade of cellular responses can be triggered, allowing the cell to react to its environment.
This function is indispensable because:
- Response Generation: It enables the cell to respond appropriately to various stimuli, which is fundamental for survival.
- Activity Regulation: Communication pathways help regulate cellular processes, ensuring everything functions in harmony within the organism.
Nonetheless, one potential drawback is:
- Miscommunication: Errors in signaling pathways can lead to diseases, such as cancer or metabolic disorders, indicating how critical accurate communication is.
Protection and Support
The cell membrane serves as a frontline defense, protecting the internal components of the cell from external forces. It also contributes to providing structural support by anchoring the cytoskeleton, a network that maintains the cell's shape and organization.
Notable characteristics include:
- Barrier Function: It acts as a shield against physical damage and pathogens, thus promoting cellular integrity.
- Structural Role: By connecting with the cytoskeleton, it helps sustain overall cell architecture.
The weaknesses of this role could be:
- Vulnerability: Certain pathogens can manipulate the membrane structure, compromising its protective capabilities. For instance, some viruses hijack the membrane to enter and infect the cell.
The cell membrane is more than just a boundary; it is an active, dynamic entity that plays critical roles in cellular function, communication, and protection. Understanding its complexities paves the way for deeper explorations into cellular health and disease mechanisms.
Cytoplasm: The Inner Workings
Cytoplasm serves as a fundamental part of a cell's structure. It is often overlooked, yet it plays a crucial role in maintaining the intracellular environment and supporting the organelles. Understanding the cytoplasm is key not just for students but also for professionals and educators in the field of cellular biology. This gel-like substance houses various cellular components, acting as a medium for biochemical reactions essential for life.
Definition and Composition of Cytoplasm
Cytoplasm can be defined as the material within a cell, excluding the nucleus but encompassing everything else. It is primarily composed of water, salts, and organic molecules. The presence of water is significant due to its role as a solvent and medium for transporting nutrients and waste.
While making up about 80% of cell volume, water also facilitates many biochemical reactions, making it a vital component for maintaining the cell's metabolic processes.
- Cytosol: This is the liquid portion of the cytoplasm, where many metabolic reactions occur.
- Organelles: These are specialized structures within the cytoplasm that perform distinct functions, which we'll discuss in the next section.
- Cytoplasmic inclusions: These are non-living substances such as lipids, glycogen, and pigments that can be found suspended within the cytoplasm.
Cytoplasmic Organelles
The cytoplasm harbors many organelles, each performing specific tasks that ensure the cell operates smoothly. Organelles can be likened to different departments in a company, where each has a unique function that contributes to the overall efficiency of operations. Here’s a brief look at some of the key organelles found in the cytoplasm:
- Mitochondria: Known as the powerhouses of the cell, mitochondria are responsible for producing ATP, the main energy currency of the cell, via aerobic respiration.
- Endoplasmic Reticulum (ER): This organelle comes in two varieties—smooth and rough. The rough ER aids in synthesizing proteins, while the smooth ER focuses on lipid synthesis and detoxification.
- Golgi Apparatus: Often referred to as the cell’s packing and shipping center, the Golgi apparatus modifies proteins and lipids before they are sent to their destination.
- Lysosomes: These are the waste disposal units of the cell, breaking down unwanted materials and cellular debris through enzymes.
- Ribosomes: Tiny but vital, ribosomes are the sites of protein synthesis and can be found either floating in the cytosol or attached to the rough ER.
To summarize, the cytoplasm not only contains these organelles but also supports their interactions, facilitating a complex network of activities that keep the cell functional. The cytoplasm provides the necessary environment for all these organelles to work together, emphasizing its critical role in cellular biology.
"Cytoplasm is more than just a filler; it is a bustling hub of activity forming the heartbeat of the cell."
In understanding the cytoplasm, one gains insight into the intricate processes that enable life. This exploration of cytoplasm sets the stage for further discussions on the more specialized organelles and their impacts on cellular functionality.
Nucleus: The Control Center
The nucleus, often referred to as the heart of the cell, plays a critical role in maintaining cellular integrity and function. Within its confines lies the genetic blueprint that governs everything from metabolism to reproduction. This section will explore the intricacies of the nucleus, emphasizing its structure and functions, which are indispensable to our understanding of cellular behavior.
Structure of the Nucleus
The nucleus is enveloped by a double membrane known as the nuclear envelope. This membrane serves as a protective barrier, differentiating the contents of the nucleus from the cytoplasm. Embedded in this envelope are nuclear pores that function like gateways, allowing selective transport of molecules between the nucleus and the cytoplasm.
Inside, the nucleus houses chromatin, a complex of DNA and proteins that packages genetic material. When a cell prepares to divide, chromatin condenses into visible chromosomes, ensuring accurate genetic replication. The nucleolus, a dense region within the nucleus, is involved in the synthesis of ribosomal RNA, which is vital for protein production.
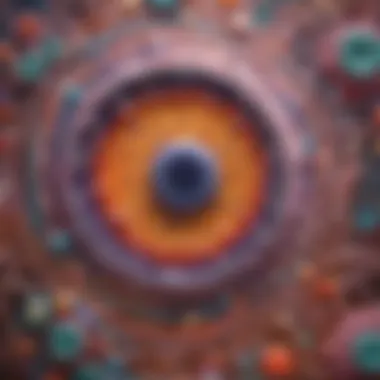
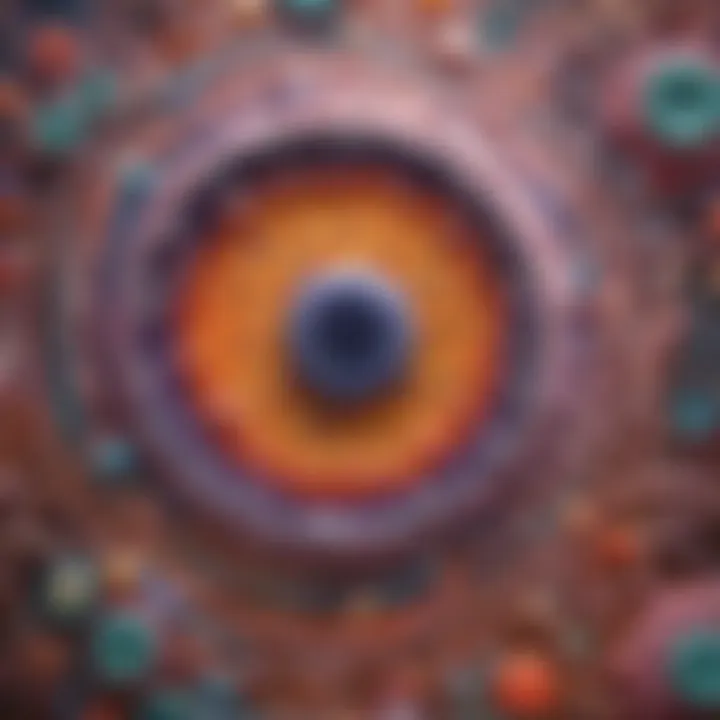
Overall, the nuclear structure is finely tuned to protect genetic information while facilitating the necessary interactions with other cellular components.
Function of the Nucleus
The nucleus serves two principal functions that are pivotal to cellular life: Storage of Genetic Material and Regulation of Cellular Activities.
Storage of Genetic Material
The storage of genetic material within the nucleus is a cornerstone of cellular life. This segment of the nucleus holds the DNA, which contains the instructions for synthesizing proteins essential for various cellular tasks. A key characteristic of this genetic storage is its remarkable organization. The DNA is wrapped around histone proteins, creating a structure that is not only compact but also dynamic. This means the genetic material can be accessed quickly when needed, allowing cells to respond adaptively to their environment.
This option is beneficial for the article as it highlights the nucleus not only as a storage area but as an active part of cellular operations. One unique feature related to storage is the presence of epigenetic modifications that can influence gene expression without altering the DNA sequence. Such modifications can play a crucial role in determining a cell's function, potentially providing advantages in acclimatizing to varying internal and external conditions.
Regulation of Cellular Activities
Regulating cellular activities is where the nucleus truly earns its title as the control center. It plays an instrumental role in signaling pathways that dictate how cells respond to environmental cues. For instance, when a cell senses a growth signal, the nucleus can initiate specific gene expressions to promote growth and division.
A fundamental characteristic of this function is its complex interplay with various other organelles. The nucleus does not operate in isolation; instead, it communicates with the endoplasmic reticulum and ribosomes to coordinate protein synthesis according to the cellular needs. This coordination is an effective choice for the article, as it emphasizes the importance of cellular communication and the intricate web of interactions that uphold cell functionality.
The ability to modulate activities based on cues can be seen as an advantage, keeping the cell in a state of homeostasis while allowing for growth, differentiation, or even apoptosis when necessary. This dynamic nature of cellular regulation underscores the importance of the nucleus in maintaining the delicate balance within cells and, subsequently, within entire organisms.
"The nucleus stands as a testament to the complexity of cellular organization and the profound significance of how life itself is orchestrated at a microscopic level."
In summary, the nucleus is not merely a repository for genetic material but a dynamic control center that oversees and regulates cellular activities. This understanding provides a deeper appreciation of how cells function and adapt, highlighting the nucleus's vital role in the grand tapestry of life.
Mitochondria: The Powerhouses
Mitochondria, often dubbed as the cell's powerhouses, play a pivotal role in the energy production processes of both prokaryotic and eukaryotic cells. Their significance stretches far beyond mere energy suppliers; they are also involved in cellular signaling, metabolism, and apoptosis. In the greater narrative of cellular biology, understanding the nuances of mitochondria enriches our knowledge of how cells function and adapt. Without these organelles, life as we know it, would be considerably different, making their exploration essential for anyone interested in cellular architecture.
Mitochondrial Structure
Mitochondria exhibit a unique structure that facilitates their numerous functions. They are enclosed by two membranes: the outer membrane is smooth and permeable to small molecules, while the inner membrane contains numerous folds known as cristae.
These cristae dramatically increase the surface area available for chemical reactions, which is crucial for energy production. Between these membranes lies the intermembrane space, and inside the inner membrane, there's liquid known as the mitochondrial matrix. This matrix holds enzymes, mitochondrial DNA, and ribosomes, allowing mitochondria to possess some autonomy from the cell. The distinct double-membrane structure not only serves functional purposes but also points to an evolutionary past, hinting at a symbiotic relationship with ancestral prokaryotic cells.
Role in Energy Production
Energy production is the core function of mitochondria, primarily achieved through aerobic respiration. This process is crucial for generating adenosine triphosphate (ATP), the cell's main energy currency.
Aerobic Respiration
Aerobic respiration is essential for most eukaryotic cells, allowing them to produce energy efficiently. It occurs in the mitochondria, where glucose and oxygen combine to generate ATP. The main characteristic of aerobic respiration is its efficiency; it generates a significantly higher yield of ATP compared to anaerobic methods.
One unique feature of aerobic respiration is its reliance on oxygen, which serves as the final electron acceptor in the electron transport chain. This characteristic is not just beneficial; it highlights the adaptability of organisms to oxygen-rich environments, enhancing their survival and functionality. The downside, however, is that cells not equipped for aerobic processes must depend on less efficient pathways, which can lead to the production of lactic acid or ethanol.
ATP Synthesis
ATP synthesis within mitochondria involves a series of complex enzymatic processes, particularly through oxidative phosphorylation. This process is unique as it directly links the energy released from electron transport to ATP production by ATP synthase.
The primary advantage of ATP synthesis in mitochondria is the huge amount of ATP produced: up to 36 ATP molecules can be generated from a single glucose molecule during aerobic respiration. This makes it a popular method in research and discussions regarding cellular energy. One challenge lies in the control of ATP production rates, which must meet the varying energy demands of the cell. If energy demands exceed production, cells can face metabolic stress.
Mitochondria not only produce ATP but also modulate signals involved in cell death and growth, reinforcing their role in maintaining cellular health.
Ribosomes: The Protein Factories
Ribosomes play a pivotal role in the broader narrative of cellular functionality. Often dubbed the "protein factories" of the cell, they are essential for the synthesis of proteins that are crucial to cellular structure and function. Without ribosomes, cells would lack the machinery to create the proteins that perform a variety of tasks, from catalyzing biochemical reactions to providing structural support.
As one delves into the details of ribosomes, one can appreciate their intricate designs and diverse functions, which are indispensable to life itself. Understanding ribosomes offers insight into not just protein synthesis but also broader cellular dynamics, including how cells respond and adapt to their environments.
Structure of Ribosomes
Ribosomes can be described as complex molecular machines composed of ribonucleic acid (RNA) and proteins. Their structure is key to their function.
Characteristics of Ribosomes:
- Two Subunits: Ribosomes are made up of two distinct subunits: the large subunit and the small subunit. The large subunit typically houses the peptidyl transferase center, which facilitates the formation of peptide bonds between amino acids. The small subunit is responsible for decoding the messenger RNA (mRNA).
- Size Variability: In eukaryotic cells, ribosomes are larger compared to those found in prokaryotic cells, generally measuring around 80S in eukaryotes versus 70S in prokaryotes. The 'S' refers to Svedberg units, which correlate to the rate of sedimentation during centrifugation and indirectly indicate size and shape.
- Ribosomal RNA (rRNA) Composition: Each subunit contains ribosomal RNA, which is crucial for the ribosomal functions. The rRNA has various structural and catalytic roles in protein synthesis.
- Binding Sites: Ribosomes feature multiple binding sites that facilitate interactions with mRNA and transfer RNA (tRNA), which are critical for the translation process.
The structural intricacy of ribosomes underscores their critical role in protein synthesis, as it allows for seamless interaction between RNA and amino acids.
Function in Protein Synthesis
The primary role of ribosomes is to translate mRNA into polypeptide chains, which fold into functional proteins. This process, known as translation, is multifaceted and involves several steps:
- Initiation: This segment begins when the small ribosomal subunit binds to the start codon on the mRNA strand. The initiator tRNA, carrying methionine, pairs with the start codon, positioning itself correctly for the upcoming assembly.
- Elongation: During this phase, the ribosome moves along the mRNA, adding new amino acids to the growing polypeptide chain. Each tRNA molecule presents an amino acid corresponding to the next codon in the mRNA sequence, allowing for the sequential addition of amino acids.
- Termination: The elongation process continues until a stop codon is reached on the mRNA. At this point, release factors bind to the ribosome, prompting the disassembly of the ribosomal units and the release of the newly formed polypeptide.
Ribosomes do more than just translate genetic information; they influence cellular regulation and response. For instance, under stress conditions, ribosomes can alter their activity, selectively translating proteins that help the cell cope with challenging environments. This adaptability highlights how ribosomes stand at the intersection of genetics and cellular function, making them integral to life's processes.
Ribosomes are not merely machines but are dynamic entities that respond to the cellular context, shaping how genes are expressed.
Ribosomes, as the protein synthesis hubs, embody the interplay between structure and function, signifying their vital contribution to the overarching cell biology narrative.
Endoplasmic Reticulum: The Manufacturing Hub
The Endoplasmic Reticulum, commonly known as the ER, is an essential organelle within the cell, often described as its manufacturing and packaging center. The ER plays a critical role in the synthesis of proteins and lipids, thereby directly impacting overall cellular function and health. Without it, cells would struggle to maintain their supplies and perform efficiently. The two distinct regions of the ER, the smooth and rough, serve varied but complementary functions. Understanding these aspects helps paint a broader picture of cellular processes and their intricate dynamics.
Structure of the Endoplasmic Reticulum
Smooth Endoplasmic Reticulum
Smooth Endoplasmic Reticulum (SER) is quite the noteworthy section of the ER. Its structure lacks ribosomes, which gives it a smooth appearance. This absence allows SER to engage in several important activities. One key feature of SER is its primary role in lipid synthesis, which is vital because fats serve various roles including cellular membrane formulation and energy storage. In addition to lipid production, the SER is also noted for its involvement in carbohydrate metabolism and the detoxification of harmful substances, such as drugs and alcohol.
Why is the Smooth Endoplasmic Reticulum often regarded as a valuable part of the cell? It’s mainly due to its capacity to modify and manage lipid levels, effectively assisting the cell in adapting to varying demands. However, while the smooth ER is often beneficial in its activities, a busy SER can indicate high metabolic activity, which may result in stress on the cell.
Rough Endoplasmic Reticulum
When discussing the Rough Endoplasmic Reticulum (RER), the most identifiable feature is its studded surface of ribosomes, which gives it a rough texture. This structure plays a central role in the process of protein synthesis. Ribosomes on the RER translate mRNA into polypeptide chains that will ultimately fold into functional proteins. The RER's involvement doesn't stop there; it also assists in the initial folding and modification of these polypeptide chains as they enter its lumen.
The RER emerges as a popular topic in cellular biology because proteins synthesized here are often destined for export from the cell or for use in the plasma membrane. Notably, any disruption in the functions of the RER can lead to misfolded proteins, causing detrimental cellular stress. This unique role of the RER showcases its importance in a healthy cellular environment, reinforcing the idea that a well-functioning RER is essential for cellular success.
Functions of the Endoplasmic Reticulum
Protein and Lipid Synthesis
The role of the ER in protein and lipid synthesis can’t be overstated. The synthesis of proteins originates in the ribosomes attached to the RER. These proteins may serve numerous functions – from acting as enzymes to serving structural roles or facilitating cell communication. On the other hand, lipid synthesis occurs primarily in the SER. The ER has adapted this dual functionality, allowing it to balance the needs of the cell seamlessly.
What makes the synthesis processes more notable is the complexity involved: it is not just about producing these macromolecules, but also ensuring they are correctly folded and modified to function effectively in the larger cellular context. This characteristic of the ER stands as a bedrock concept of cellular biology.
Detoxification Processes
Detoxification is another crucial function of the Endoplasmic Reticulum, mainly undertaken by the SER. This aspect involves chemically modifying and neutralizing drugs, toxins, or other harmful substances that might enter the cell from the environment. The SER can contribute to this detoxification process by adding various functional groups to these harmful compounds, making them more water-soluble and easier to expel from the body.
The significance of detoxification cannot be ignored, especially in the context of human health where exposure to pollutants or excessive alcohol consumption is common. A robust detoxification process within the cell enables it to maintain its balance and fend off potential damage, adding another layer to the impressive capabilities of the Endoplasmic Reticulum.
A well-functioning Endoplasmic Reticulum is essential for maintaining overall cell health, highlighting the intricate relationship between cellular machinery and environmental factors.
This discussion encapsulates the fascinating world of the Endoplasmic Reticulum, serving as a reminder of how interconnected cellular structures are in the grand scheme of life.
Golgi Apparatus: The Sorting Center
The Golgi apparatus holds a pivotal role in a cell's operations, serving as its sorting center and distribution hub. Without this component, cellular processes could easily fall into disarray. Think of it like a post office that not only receives packages but also organizes and sends them to the right destinations. This analogy highlights its fundamental importance in managing the flow of proteins and lipids within the cell. The uniqueness of the Golgi apparatus stems from its hierarchical structure and multifaceted functions, which are crucial for maintaining cellular homeostasis.
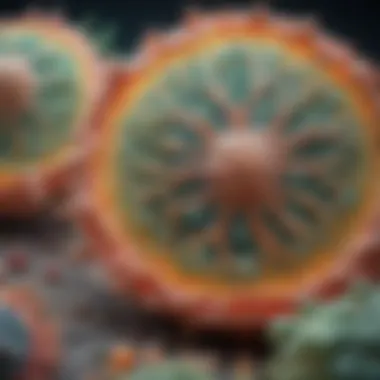
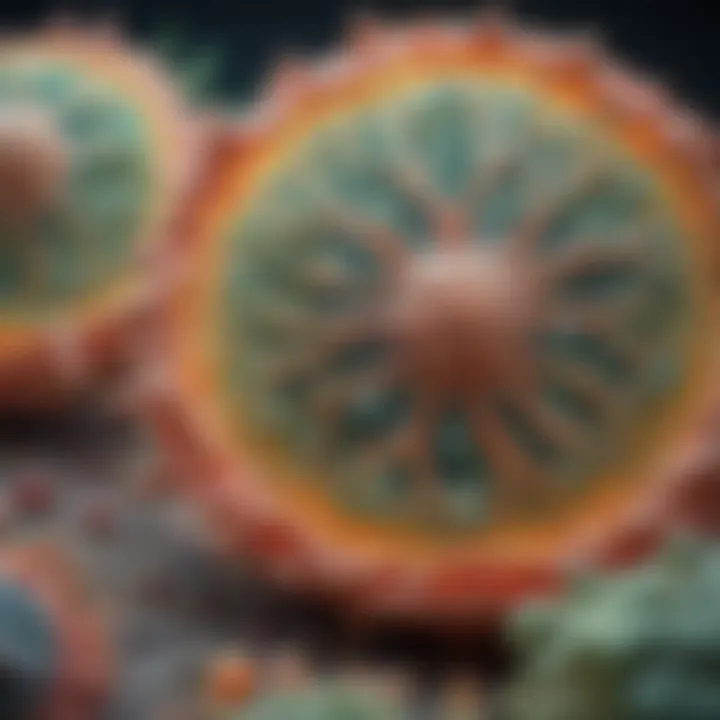
Structure of the Golgi Apparatus
The Golgi apparatus consists of a series of flattened, stacked pouches known as cisternae. These structures work together seamlessly to process various cellular substances. Here is an overview of its intricate anatomy:
- Cisternae: These are the disc-like, membrane-bound structures that form the bulk of the Golgi. They resemble a stack of pancakes, emphasizing the Golgi's role in packaging materials.
- Incoming Transport Vesicles: Vesicles contain proteins and lipids that travel to the Golgi from the endoplasmic reticulum (ER). They fuse with the cis face of the Golgi apparatus, which is the receiving side.
- Outgoing Transport Vesicles: Once processed, vesicles bud off from the trans face, delivering their contents to specific locations in or outside the cell.
This layered configuration allows the Golgi to compartmentalize its functions, ensuring that modifications and sorting can proceed effectively.
Function in Cellular Logistics
At the heart of the Golgi's function is its role in cellular logistics. It manages both the modification and transport of critical biomolecules, ensuring they are in the right form and reach their desired location. A couple of key aspects stand out in this process:
Modification of Proteins
In this section, the Golgi apparatus plays a significant role in the post-translational modification of proteins. This process involves chemical alterations that can include glycosylation, phosphorylation, or sulfation. These modifications are crucial for the following reasons:
- Key Characteristic: Protein modification enhances functionality and stability. For instance, glycosylated proteins can better interact with other molecules, thus participating in a number of processes including cell signaling and immune responses.
- Contribution: The appropriateness of these modifications ensures proteins are functional once dispatched to their destinations. Without these adjustments, proteins may fail to perform their duties effectively or could even cause cellular dysfunction.
A unique feature of protein modification in the Golgi is its specificity. Each protein types undergoes its own set of tailoring, underscoring the meticulous attention to detail within cellular processes. However, misfolding or incomplete modifications can lead to disorders, highlighting the Golgi's importance in health.
Transport and Packaging
The transport and packaging function of the Golgi apparatus facilitates safe and efficient dispatch of cellular products. Once proteins have been properly modified, they are sorted and packed into vesicles for delivery. Let’s delve deeper:
- Key Characteristic: The Golgi apparatus ensures that proteins are sent to the correct destination. This organized dispatch minimizes potential errors that could disrupt cellular processes.
- Contribution: The packaging process is not merely about transport; it also involves encapsulating molecules in lipid membranes, adding an additional layer of protection.
The unique aspect of the transport mechanism is its ability to adapt to differing cellular needs. For example, certain signaling proteins may need to be delivered quickly, while others can be delayed, showing the Golgi's flexibility in meeting dynamic cellular demands.
In short, the Golgi apparatus serves as the nexus for managing the lifecycle of proteins and lipids within a cell. Its structure and logistics optimize cellular function, making it a focal point in our exploration of cellular architecture.
"The Golgi apparatus is not just a sorting center; it's a complex network that underscores the intricate ballet of cellular organization."
Through a careful examination of the Golgi apparatus, its structure and functions become vastly more illuminating, emphasizing just how vital it is to cellular integrity and functionality.
Lysosomes: The Digestive System
Lysosomes hold a vital role in cellular biology, functioning essentially as the waste disposal system of the cell. These small organelles contain a cocktail of enzymes, capable of breaking down various biomolecules. Without them, cells would quickly become cluttered with damaged or unneeded components, leading to dysfunction. Their importance is underscored by their ability to recycle cellular debris, thus ensuring that cellular processes run smoothly. Moreover, lysosomes contribute to the cell's ability to adapt to changes in the environment, making their study fundamental to understanding cellular functionality.
Lysosomal Structure
Lysosomes are membrane-bound organelles characterized by their roughly spherical shape. Their membrane is crucial for maintaining an acidic internal environment, which is essential for the optimal activity of the digestive enzymes within.
- Key components of lysosomes include:
- Lipid bilayer: This protects the enzymes from the rest of the cell.
- Hydrolytic enzymes: These enzymes can break down proteins, lipids, nucleic acids, and carbohydrates.
- Proton pumps: These help maintain the internal acidic pH, usually around 4.5 to 5.0.
The successful encapsulation of enzymes within the lysosomal membrane is what prevents potential harm to other cellular components. If these enzymes were free-floating within the cytoplasm, they would be able to degrade essential cell parts, leading to cellular chaos.
Roles in Digestion and Waste Removal
Lysosomes play a multifaceted role in digestion and waste management within cells. They break down excess or worn-out cellular components, facilitating an important recycling process. When a cell engulfs debris or macromolecules through endocytosis, lysosomes fuse with the resulting vesicles and unleash their digestive enzymes to dismantle the material.
- Primary functions of lysosomes include:
- Autophagy: This is the process by which lysosomes digest damaged organelles, thus sustaining cellular health. It's like a spring cleaning for cells.
- Heterophagy: Involves the degradation of external materials brought into the cell, enabling nutrient acquisition and waste reduction.
- Pathogen elimination: Lysosomes can digest pathogens engulfed by immune cells, playing an essential role in the immune response.
In essence, if lysosomes are the digestive system of the cell, then they are crucial not merely for cleanup but for overall cellular maintenance and health.
Overall, lysosomes ensure that cellular waste doesn’t pile up and that all the components of a cell can come together seamlessly to sustain life. Understanding these organelles sheds light on broader aspects of cellular functioning and highlights their significance in maintaining health at the cellular level.
Cytoskeleton: The Structural Framework
The cytoskeleton is a critical component of cellular architecture, serving as the scaffolding that maintains cell shape, organizes cellular components, and facilitates movement. It plays a vital role in various cellular functions, offering stability while remaining flexible enough to allow changes in cell form. By understanding the intricacies of the cytoskeleton, we gain deeper insight into the cellular mechanics that underpin life itself.
Components of the Cytoskeleton
Microfilaments
Microfilaments, made primarily of actin, are the thinnest components of the cytoskeleton. They offer structural support and are key players in various cellular processes including motility. One key characteristic of microfilaments is their ability to rapidly grow and shrink, which plays a crucial role in cell shape changes and movement.
Microfilaments enable cells to crawl and extend in different directions, important for processes like wound healing or immune responses.
The unique feature of microfilaments lies in their dynamic nature. They can rapidly polymerize or depolymerize, providing the cell with the versatility it needs to adapt to its environment. While being advantageous, the rapid turnover of microfilaments can also lead to instability if not properly regulated. Their beneficial properties, however, make them essential for processes such as cytokinesis, where cell division occurs, and amoeboid movement seen in some white blood cells, to name a few.
Intermediate Filaments
Intermediate filaments serve to stabilize the structure of the cell and provide tensile strength. Unlike microfilaments, they are more permanent components, offering mechanical stability. One notable characteristic of intermediate filaments is their resistance to tension, helping cells withstand stress and strain during various activities.
Their unique feature is their composition, which varies depending on cell type, e.g., keratin in epithelial cells and neurofilaments in neurons. This specificity allows intermediate filaments to contribute to the unique structural characteristics of different tissues. However, since they do not easily disassemble and remodel, they lack the flexibility that microfilaments possess, possibly limiting certain cellular responses. They play a critical role in maintaining cell integrity and protecting against mechanical disruption.
Microtubules
Microtubules are the thickest component of the cytoskeleton and are essential for maintaining cell shape, facilitating intracellular transport, and enabling cell division. Comprised of tubulin dimers, a key characteristic of microtubules is their ability to form a network throughout the cell that acts as tracks for the movement of organelles, vesicles, and chromosomes.
The unique feature of microtubules is their structural polarity, which allows for the directional transport of cargo within the cell via motor proteins like kinesin and dynein. While microtubules are beneficial for transport and for providing rigidity, they can become unstable if their assembly or disassembly is not properly regulated, which could lead to compromised cellular function. Their role in forming the mitotic spindle during cell division underscores their importance in cellular replication and organization.
Function of the Cytoskeleton
Cell Shape Maintenance
The cytoskeleton plays an indispensable role in maintaining the overall shape of a cell. It acts like a framework that provides support, helping to prevent deformation under various external pressures. One important characteristic of this function is adaptability; the cytoskeleton can adjust to changes in cell shape, enabling phenomena such as cell migration and growth.
Its unique features include the ability to rapidly reorganize its components to accommodate the shape changes, such as during cell division or response to environmental stimuli. This adaptability presents advantages for cells that require significant alterations in shape, such as muscle cells during contraction or white blood cells during immune responses. Failure to maintain proper shape can lead to stiffness or malformations, affecting the cell’s functionality.
Cell Motility
Cell motility is a fundamental aspect facilitated by the cytoskeleton, involving the muscle cells' ability to contract or the movement of cells across surfaces, such as during wound healing. The dynamic nature of microfilaments, for instance, allows for the extension of cellular protrusions like lamellipodia and filopodia, essential in cell movement.
The key characteristic of motility is the cooperative interaction between different components of the cytoskeleton, working together to allow cells to propel themselves. A notable unique feature is the way motor proteins interact with microtubules and microfilaments to generate movements, an essential process in both single-celled organisms and multicellular entities. While this is advantageous for immune response and organismal development, excessive motility can sometimes lead to detrimental conditions, such as in cancer metastasis when cells migrate uncontrollably.
Through understanding the cytoskeleton and its components, we not only appreciate its complexity but also grasp the importance of cellular architecture in maintaining life and functionality at a micro level.
Cell Division: A Key Process
Cell division stands as one of the most vital events that occurs within living organisms. It’s like a dance of sorts, replete with intricate steps, ensuring that life continues and evolves. This process is fundamental not only for growth but also for the repair of tissues, the replacement of worn-out cells, and reproduction in various forms.
Dividing is how cells maintain their number and functional integrity, navigating through a cycle of life that allows organisms to thrive. When we take a closer look at cellular processes, it becomes clear that each phase of cell division bears significance for the holistic understanding of cellular architecture and function.
"Without cell division, life as we know it would be impossible; it’s the linchpin that holds the fabric of life together."
Phases of Cell Division
Cell division is generally categorized into two primary processes: mitosis and meiosis. Each pathway consists of distinct phases, facilitating various types of cellular outcomes.
Mitosis
Mitosis is the process responsible for cell replication, essential for growth and maintenance. It is divided into several stages:

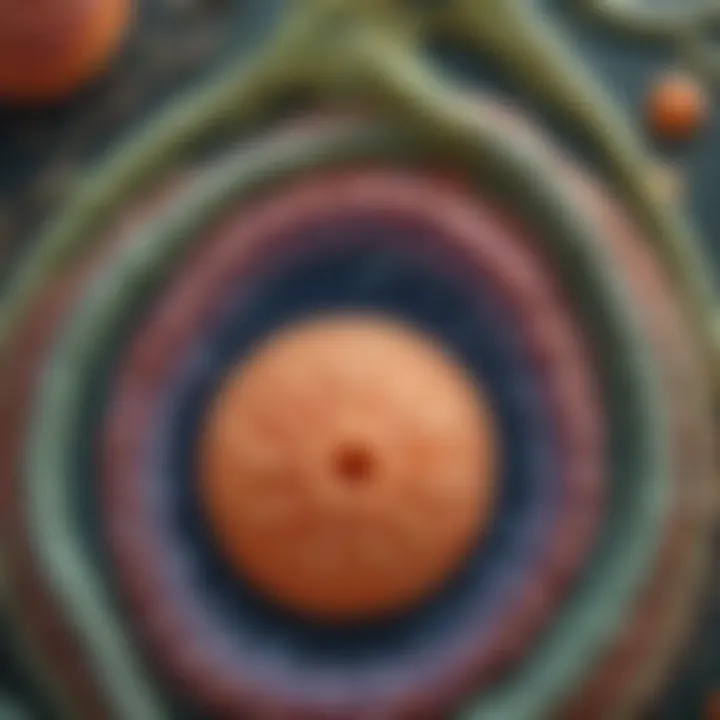
- Prophase: Chromatin condenses into visible chromosomes, and the nuclear membrane starts to break down.
- Metaphase: Chromosomes align at the cell's equator, ensuring that each daughter cell will receive an identical set.
- Anaphase: Sister chromatids are pulled apart, moving towards opposite poles of the cell.
- Telophase: Chromatids reach the poles, and the nuclear membrane reforms, resulting in two nuclei within a single cell.
- Cytokinesis: Finally, the cytoplasm divides, yielding two separate daughter cells.
Meiosis
Meiosis is specialized for the formation of gametes in sexual reproduction. It undergoes two sequential divisions:
- Meiosis I includes prophase I, metaphase I, anaphase I, and telophase I, reducing chromosome numbers by half.
- Meiosis II resembles mitosis, resulting in four non-identical daughter cells.
Importance of Cell Division
The importance of cell division cannot be overstated—it is the backbone of biological systems. Here are several key benefits and considerations associated with this process:
- Growth and Development: Cell division enables organisms to grow and develop from single cells into complex multicellular entities.
- Repair and Regeneration: Whether it's a skin scratch or damaged tissues, cell division facilitates healing, replacing lost or damaged cells, and maintaining homeostasis.
- Genetic Continuity: During mitotic division, genetic materials are equally divided, producing clone-like daughter cells. Meiosis, on the other hand, introduces genetic variations through recombination and independent assortment. This variation is critical for evolutionary processes.
- Reproduction: In unicellular organisms, division is a means of reproduction. In multicellular organisms, it primes the formation of gametes for reproduction.
- Cellular Functionality: Division also influences cellular differentiation, whereby unspecialized cells can become specialized to perform distinct functions.
The implications of cell division extend beyond the laboratory and into everyday life. It is integral to fields from healthcare to agriculture, influencing how we understand growth patterns in plants, the effects of cancer, and even regenerative medicine approaches.
In summary, comprehending the mechanisms and importance of cell division enriches our collective knowledge of cellular structures and processes, paving the way for advancements in biological sciences.
Cellular Communication: The Signaling Systems
Cellular communication is at the heart of how cells interact, respond, and adapt to their surroundings. This crucial aspect of cellular biology encompasses various mechanisms that allow cells to send and receive signals, facilitating coordination and functionality within tissues and organisms. Understanding cellular signaling systems is not just about delving into molecular interactions; it's about grasping how these processes influence everything from basic survival to complex behaviors in multicellular organisms.
Chemical Signals and Receptors
At the core of cellular communication are chemical signals, which can be hormones, neurotransmitters, or other types of signaling molecules. When these chemical signals are released, they traverse the intercellular space to bind to specific receptors on target cells. This interaction is akin to a key fitting into a lock, where the correct chemical signal (the key) must match the receptor (the lock) to elicit a response.
There are two primary classes of receptors:
- Membrane-bound receptors: These receptors sit on the cell surface and typically interact with larger or polar signaling molecules. Examples include insulin receptors that facilitate glucose uptake in response to insulin binding.
- Intracellular receptors: These receptors, located within the cell, usually bind to smaller and nonpolar molecules, such as steroid hormones, allowing them to directly affect gene expression.
The specificity of these receptors ensures that cells respond appropriately to a myriad of stimuli, maintaining homeostasis and reacting to any changes in the environment. The interplay of chemical signals and receptors is essential for regulating various cellular functions such as growth, metabolism, and immune responses.
Cell Signaling Pathways
Once a chemical signal has attached to a receptor, a cascade of events commonly known as a cell signaling pathway is initiated. These pathways are intricate networks of proteins that transmit the signal from the cell surface to its interior, resulting in specific cellular responses.
In broad terms, cell signaling pathways can be divided into a few key categories:
- Receptor Tyrosine Kinases (RTKs): When activated by a signal, RTKs undergo a conformational change, leading to rapid phosphorylation of tyrosine residues on their cytoplasmic domain. This serves as a docking site for signaling proteins, propagating the signal within the cell.
- G-Protein Coupled Receptors (GPCRs): GPCRs activate G-proteins which can trigger diverse signaling pathways, influencing functions like cell division, movement, and even neurotransmitter release.
- Intracellular Receptors and Gene Expression: Some signaling pathways lead to changes in gene expression, altering the production of proteins that ultimately shape the fate of a cell.
These pathways ensure responsiveness to external cues and play pivotal roles in various physiological processes including:
- Development: Ensuring proper growth and differentiation of cells.
- Homeostasis: Maintaining internal stability despite changes in the external environment.
- Response to Stress: Guiding how cells react to damage, infection, or other stressors.
The complexity and precision of signaling pathways illustrate just how sophisticated cellular communication is, allowing for a coordinated approach to biological function and adaptation.
In sum, cellular communication through chemical signals and signaling pathways is integral to the functioning of life itself. By understanding these systems, researchers can gain insights that can lead to advancements in medicine, biotechnology, and our overall grasp of biology.
Cellular Adaptations: Response to Environment
Cellular adaptations are fundamental responses that allow cells to endure environmental fluctuations and persist in a state conducive to life. This section elucidates why understanding these adaptations is crucial for comprehending cellular functionality within organisms. Through adaptations, cells demonstrate an incredible capacity to modify their structure and behavior in reaction to external stimuli. This is particularly significant in fields like medicine, environmental biology, and evolutionary studies where the adaptability of cells can be the key to survival and success.
Cellular adaptations show the resilience of life in the face of adversity, reflecting the profound connection between structure and function.
Mechanisms of Cellular Adaptation
The mechanisms through which cells adapt to their environments can be diverse and intricate. Here are a few specific ways cells may adjust their processes:
- Genetic Regulation: Cells often alter gene expression in response to changes in their environment. This might involve turning specific genes on or off to synthesize proteins that aid in adaptation. For instance, when exposed to a high-salinity environment, some cells ramp up the production of salt-exclusion proteins.
- Metabolic Alterations: Depending on available nutrients, a cell might shift its metabolic pathways. For example, under low oxygen conditions, cells can transition from aerobic respiration to anaerobic pathways, allowing them to continue producing energy.
- Structural Changes: Cells can also undergo physical changes in their structure. For instance, when a cell experiences tension or stretching, it may enhance its cytoskeletal elements, providing greater mechanical support and resilience against stretching forces.
These mechanisms not only facilitate survival but also optimize the efficiency of cellular processes, maximizing the chance for longevity and reproduction in a competitive environment.
Examples of Adaptation in Cells
Adaptation manifests in numerous scenarios across various cell types:
- Thermophilic Bacteria: Found in hot springs, these bacteria possess unique proteins that maintain their stability and functionality at high temperatures. The heat shock proteins they produce help avoid denaturation, ensuring their cellular machinery remains intact.
- Plant Responses to Drought: During periods of drought, plants close their stomata to reduce water loss. Cells in the roots might also initiate the production of aquaporins, enhancing water absorption from the soil thorough osmosis.
- Turtle Blood Cells: Some turtle species can survive periods of low oxygen under water by significantly slowing their metabolism. Turtle blood cells are specifically capable of functioning and producing energy in such low-oxygen environments, showcasing a remarkable adaptation mechanism.
These examples underline the vast reservoir of strategies cells employ to tackle environmental challenges. Much is still to be explored in the field, which presents exciting opportunities for researchers aiming to study evolution, biodiversity, and cellular resilience.
Understanding these adaptations not only enriches our comprehension of cellular biology but also inspires innovative approaches in biotechnology and medicine.
Comparative Analysis of Cell Types
In the study of cellular biology, understanding the differences and similarities among cell types serves as a critical foundation. The comparative analysis of cell types reveals insights into the evolution, functionality, and adaptability of organisms. Differentiating between prokaryotic and eukaryotic cells, as well as between diverse types like plant and animal cells, can deepen our understanding of how life operates at the most basic level. Grasping these distinctions allows researchers to better appreciate the complexity and diversity of life.
Differences Between Plant and Animal Cells
Recognizing the dissimilarities between plant and animal cells is not just academic—it's essential for understanding their respective functions in ecosystems. Here are some key differences:
- Cell Wall: Plant cells are surrounded by a rigid cell wall made of cellulose, offering structure and protection. In contrast, animal cells lack this layer, relying on a flexible plasma membrane for shape and containment.
- Chloroplasts: Only plant cells contain chloroplasts, necessary for photosynthesis. This allows plants to convert sunlight into energy, a process that animals rely on indirectly through the consumption of plant material.
- Vacuoles: While both cell types may have vacuoles, plant cells possess a large central vacuole that stores water and maintains turgor pressure, which is crucial for plant rigidity. Animal cells have smaller vacuoles, often involved in storage and transport rather than structural support.
These differences illustrate how both cell types are adapted to their environments and functions within the framework of life. Understanding these properties helps students and researchers grasp how life varies so widely yet remains interconnected.
Uni-Cellular vs Multi-Cellular Organisms
The distinction between uni-cellular and multi-cellular organisms is another fascinating area of cellular analysis. Uni-cellular organisms, like bacteria and some protists, consist of a single cell that performs all necessary life functions. This efficiency is crucial for survival in diverse environments and can lead to rapid adaptation.
On the other hand, multi-cellular organisms, including humans, animals, and plants, are built from numerous cells working in harmony. Key points of this distinction include:
- Specialization of Cells: In multi-cellular organisms, cells often differentiate into specialized types, such as muscle, nerve, and epithelial cells, each performing unique functions. This specialization allows for greater complexity and efficiency in processes like movement and communication.
- Dead Cells: Multi-cellular organisms often contain many dead cells, such as the outer layer of skin cells, which serve protective roles without requiring active maintenance. In uni-cellular organisms, the entire entity must remain functional.
- Cell Communication: Communication between cells is essential in multi-cellular organisms, facilitated through complex signaling pathways, while uni-cellular organisms rely more on their own internal processes to respond to environmental changes.
By exploring these differences, we gain insights into the intricacies of life and how cellular structures and functions adapt to meet the challenges of different environments. This analysis emphasizes the remarkable variability in cellular designs that allows life to thrive across various ecosystems.
"The comparative study of cell types offers a lens through which we can grasp the grand tapestry of life, showcasing both unity and diversity at the cellular level."
By appreciating these cellular dynamics, students, researchers, and educators can gain a refined understanding of biology, paving the way for future discoveries in the field.
Ending: Integrative Understanding of Cell Functionality
The realm of cellular biology is vast and layered, tying together the intricate mechanisms that dictate life as we know it. Understanding cellular functionality enables a comprehensive grasp of not just the components but their interplay and harmony within the multitude of life forms. It’s not enough to view these elements in isolation; their relationships and functions collectively contribute to the dynamism inherent within cells.
The various components of the cell, from the cell membrane protecting its integrity to the nucleus harboring genetic blueprints, serve specific roles that are vital for cellular health and organismal viability. In summary, each piece of the puzzle plays a significant part:
- Cell Membrane: Acts as a gatekeeper, maintaining the environment within.
- Nucleus: The brain of the operation, ensuring genetic information is stored and utilized effectively.
- Mitochondria: Power production units, converting nutrients into energy.
- Endoplasmic Reticulum: Factory floors where proteins and lipids are assembled.
- Golgi Apparatus: Transportation hub that prepares and routes proteins to their needed destinations.
Having a solid grasp of these interrelations empowers students, researchers, and educators to appreciate the complexity of life. It allows for enhanced communication across various scientific disciplines and lays the groundwork for further research.
Understanding how cells operate is like being given a front-row seat to the symphony of life, where every instrument has its melody and contributes to the overall harmony.
Summarizing Key Cellular Functions
In revisiting the significant functionalities of cellular components, attention must be given to their collaborative nature. Here’s a snapshot of the key functions explored throughout the article:
- Protective Roles: Cell membranes establish selective permeability, ensuring the necessary substances enter while keeping harmful ones at bay.
- Energy Production: Mitochondria and chloroplasts in plant cells work together to harness energy, with mitochondria focusing on aerobic respiration for ATP synthesis.
- Information Processing: The nucleus plays a crucial role in not only storing but also regulating gene expression, pivotal for cellular responses to environmental stimuli.
- Synthesis and Transport: The function of the endoplasmic reticulum and Golgi apparatus in producing and modifying proteins is indispensable for cellular systems to operate effectively.
- Waste Management: Lysosomes contribute to cellular cleanliness, ensuring that metabolic waste is digested and recycled when needed.
This summary anchors the understanding of cell functionality into practical, relatable concepts, making it easier to recognize how slight disturbances can lead to major consequences in biological systems.
Future Directions in Cellular Research
Looking ahead, the field of cellular biology is poised for transformative advancements. As technology evolves, several trajectories stand out:
- Single-Cell Sequencing: This technique allows researchers to examine the genomic data of individual cells, revealing variations and functions that bulk analyses may obscure.
- Synthetic Biology: The creation of biological systems through engineering offers possibilities for developing new therapies, enhancing cell functions, and creating biomaterials.
- CRISPR and Gene Editing: These tools will not only enhance our understanding of genetic functions but may also enable the correction of genetic disorders at their source.
- Cellular Imaging Techniques: Enhancements in imaging technologies will grant deeper insights into cellular processes in real-time, allowing researchers to visualize interactions that were once elusive.
As research continues to peel back the layers of cellular mechanics, the insights gleaned can lead to better approaches and solutions in medicine, environmental conservation, and biotechnology. It’s an exciting time to be engaged in cellular biology, as every discovery opens a new chapter in understanding life itself.