Understanding Light Scattering: Mechanisms and Applications
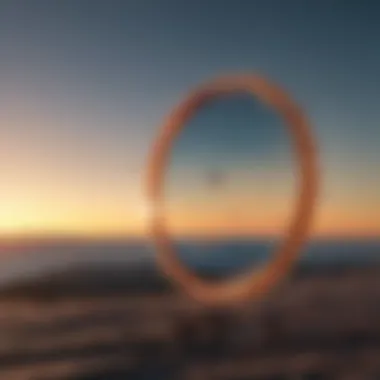
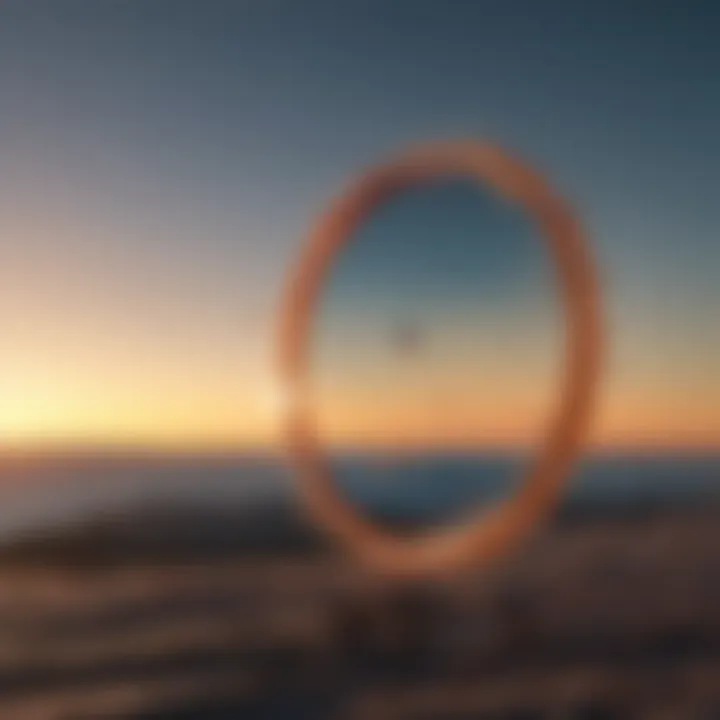
Intro
The scattering of light is a phenomenon that has intrigued scientists for centuries. It encompasses a diverse range of interactions between light and matter, leading to insights that permeate various fields. Understanding how light scatters is not merely an academic pursuit; it is crucial for applications spanning meteorology, astronomy, and material science.
By examining different types of scattering, such as Rayleigh and Mie, we can appreciate their diverse implications. Beyond theoretical concepts, the practical applications of light scattering also play a vital role in everyday life. This article offers a comprehensive exploration of light scattering principles, relevant mechanisms, key findings in research, and the broader context surrounding its study.
Scattering phenomena reveal critical information about the universe. As we dive deeper, the complexities of light-matter interactions become clearer, highlighting their significance in both scholarly exploration and technological innovation.
Research Overview
Key Findings
Research on light scattering reveals several pivotal insights:
- Rayleigh Scattering: This fundamental type of scattering occurs when light interacts with particles much smaller than its wavelength. It accounts for the blue color of the sky and the red hues during sunrise and sunset.
- Mie Scattering: Unlike Rayleigh, Mie scattering involves larger particles. It explains the white appearance of clouds and other atmospheric phenomena.
- Applications in Precision Measurement: Techniques leveraging light scattering are critical in fields such as optical sensor technology, providing precise measurements in various scientific applications.
Study Methodology
The studies on light scattering employ diverse methodologies, combining theoretical modeling with experimental approaches. Research often integrates:
- Mathematical Frameworks: Employing Maxwell's equations and other principles to develop predictive models.
- Experimental Data Collection: Utilizing lasers and photodetectors to obtain real-time measurements in controlled environments.
Background and Context
Historical Background
The study of light scattering dates back to the early 19th century. John William Strutt, Lord Rayleigh, significantly advanced this field through his exploration of Rayleigh scattering. This laid the foundation for understanding how light interacts with various particles.
Current Trends in the Field
Today, the exploration of light scattering has expanded, focusing on:
- Nanotechnology: Research is increasingly directed toward how light scatters in nanostructured materials, aiding advancements in material science.
- Astrophysics: The role of light scattering in understanding cosmic phenomena continues to be a vibrant field of investigation.
By illuminating both the history and current trends of light scattering, we can appreciate its significance and how ongoing research contributes to the scientific landscape.
Understanding the nuances of light scattering provides essential knowledge that drives innovation in multiple disciplines. As the inquiry into this complex topic continues, the implications of scattered light will undoubtedly grow in relevance.
Preamble to Light Scattering
Light scattering is an essential phenomenon that crosses various fields of science and technology. Understanding light scattering can provide insights into how light interacts with matter, which is critical for both theoretical studies and practical applications. The study of light scattering encompasses different mechanisms, including Rayleigh and Mie scattering, which have unique implications in various domains such as meteorology, astronomy, and material science.
This phenomenon plays significant roles in environmental assessment, medical diagnostics, and even daily experiences like the blue sky or red sunsets. By learning about the principles of light scattering, researchers and practitioners can better interpret environmental data and innovate within their fields.
Each mechanism of scattering offers distinct characteristics that scientists utilize for specific applications. For instance, Rayleigh scattering explains why the sky appears blue, primarily influenced by the tiny particles in the atmosphere. On the other hand, Mie scattering, which occurs with larger particles, has critical implications in atmospheric optics.
Given its breadth, the study of light scattering encompasses analysis, measurement techniques, and inquiry into the nature of light itself. This article provides a detailed exploration of these aspects, helping readers appreciate the complexity and significance of light scattering in both scientific and everyday contexts.
Definition and Importance
Light scattering refers to the redirection of light rays when they encounter particles or molecules, leading to various optical phenomena. The importance of light scattering extends beyond mere definitions; it underpins numerous applications in environmental science, healthcare, and industrial processes.
Light scattering can help assess air and water quality, aiding in environmental monitoring. Additionally, it plays a vital role in biomedical imaging and therapies, highlighting its relevance for health sciences. Understanding this process maximizes the benefits across these domains, leading to advancements in technology and improved public awareness of environmental concerns.
Through a comprehensive grasp of light scattering, individuals can harness its principles for innovative solutions. Thus, the study is essential not just as a theoretical endeavor but also as a practical one.
Historical Context
The exploration of light scattering has deep historical roots, evolving through centuries of scientific inquiry. From early theories by Isaac Newton to modern advancements in optics, the journey has been transformative.
- In the 19th century, Lord Rayleigh conducted pivotal work explaining the scattering of light in the atmosphere, establishing foundations for understanding how light interacts with air. His work elucidated why the sky is blue, providing a clear example of Rayleigh scattering.
- Subsequently, Mie theory, developed by Gustav Mie in the early 20th century, further expanded knowledge on light scattering by larger particles.
This historical progression highlights the ongoing nature of scientific research, where foundational ideas lead to advancements in understanding phenomena. The insights gained from past studies remain crucial today, influencing current applications and guiding future research.
Theoretical Foundations
The theoretical foundations of light scattering are critical to understanding its principles and applications. These fundamentals guide the comprehension of how light interacts with matter, providing insights into various phenomena observed across different scientific fields. By dissecting these theories, we can evaluate the impacts on meteorology, optical technologies, and environmental science, among others. This section explores the nature of light and its wave properties, emphasizing their role in scattering phenomena.
Nature of Light
Light embodies both wave and particle characteristics, a duality that underpins its interaction with matter. Classical physics describes light as an electromagnetic wave, consisting of oscillating electric and magnetic fields. This wave nature is evident through phenomena such as interference and diffraction.
On the other hand, the particle aspect of light is articulated through quantum mechanics, where light is composed of photons. These photons carry energy that is dependent on their frequency. Thus, light behaves differently depending on the context of its interaction with particles, influencing how scattering occurs.
Understanding this duality helps scientists predict and quantify the effects of light scattering in various environments, making it essential for accurate modeling and application in real-world scenarios.
Wavelength and Frequency
Wavelength and frequency are fundamental attributes of light that significantly influence scattering processes. Wavelength refers to the distance between consecutive peaks of a light wave, while frequency denotes the number of cycles that pass a point in a unit of time, usually measured in Hertz.
The relationship between these two properties of light can be captured by the equation:
[ c = \lambda \cdot f ]
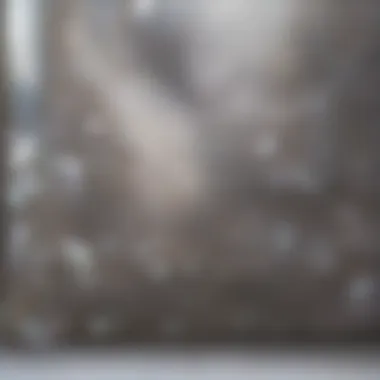
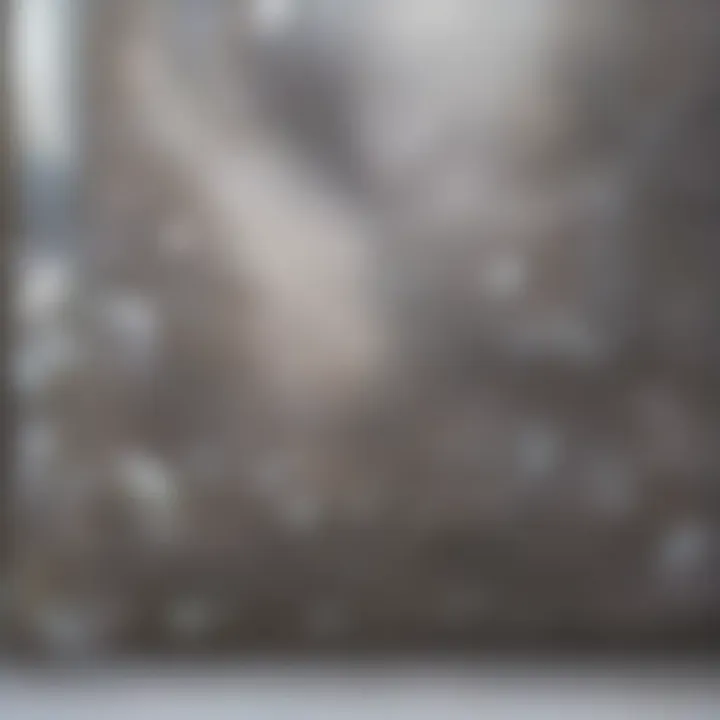
where (c) represents the speed of light in a vacuum, (\lambda) is the wavelength, and (f) is the frequency. This equation encapsulates the interdependence of wavelength and frequency, highlighting that as one increases, the other decreases.
The significance of these properties in light scattering is profound. For example, shorter wavelengths, such as blue light, scatter more than longer wavelengths like red light. This principle is vividly observable in Rayleigh scattering, which explains why the sky appears blue during the day. The understanding of wavelength and frequency plays a crucial role in various scientific applications, ranging from astrophysics to industrial technologies.
"The intricate dance of light, as it traverses different media, reveals important truths about nature and our understanding of the universe around us."
Through these theoretical lenses, we can begin to appreciate the complexities of light scattering, elucidating how it shapes our interaction with the world.
Mechanisms of Light Scattering
Understanding the mechanisms of light scattering is essential for a comprehensive grasp of how light interacts with various materials. This section highlights key scattering phenomena, specifically focusing on Rayleigh and Mie scattering. These mechanisms not only provide insights into optical behaviors but also underscore the significance of light scattering across multiple domains, from environmental science to industrial applications. Exploring these details illuminates the complexities involved in analyzing light interactions and their practical implications.
Rayleigh Scattering
Basic Principles
Rayleigh scattering is a physical phenomenon where light is scattered by particles much smaller than its wavelength. The intensity of the scattered light is inversely proportional to the fourth power of the wavelength, meaning shorter wavelengths scatter more than longer ones. This aspect is paramount in explaining why the sky appears blue. The key characteristic of Rayleigh scattering lies in its dependence on particle size and light wavelength, making it a beneficial choice for demonstrating light's interaction with gas molecules.
Unique Feature: One distinctive feature is the strong wavelength dependency, which allows scientists to infer information about the atmosphere’s composition. However, this principle also presents limitations, as it is ineffective for larger particles where Mie scattering becomes relevant. Therefore, while it aids in understanding atmospheric effects and visual phenomena, it may not suffice in situations with larger scatterers.
Applications in Meteorology
Rayleigh scattering holds significant importance in meteorology. By analyzing scattered light, meteorologists can deduce cloud formations, pollution levels, and atmospheric conditions. The key characteristic lies in its ability to enhance visibility predictions and weather forecasts. Utilizing this principle enables the modeling of light interaction in various atmospheric scenarios, which is beneficial for predicting weather patterns.
Unique Feature: Rayleigh scattering also allows for identifying different gases within the atmosphere, providing data essential for climate studies. A limitation arises in scenarios where dense clouds obscure light, complicating assessments. Despite this, its contributions to atmospheric science remain substantial, providing foundational knowledge for more complex models.
Examples in Everyday Life
Rayleigh scattering manifests in daily experiences, such as the vivid colors observed during sunrise and sunset. The light from the sun travels through more atmosphere at these times, scattering shorter wavelengths and allowing longer wavelengths to dominate. This phenomenon's key characteristic is its availability to any observer, making it a relatable example of light scattering.
Unique Feature: It illustrates the broader implications of light scattering beyond scientific contexts. However, understanding this process may require deeper comprehension of light physics, potentially limiting casual observers. Regardless, the connection to everyday life enhances engagement with the science behind such occurrences, making it a popular choice for teaching light behavior.
Mie Scattering
Comparison with Rayleigh
Mie scattering differs from Rayleigh scattering primarily due to the size of scattering particles being comparable to the wavelength of light. This aspect allows it to describe interactions more effectively in many real-world scenarios, especially in atmospheric science. The key characteristic of Mie scattering can address irregularities in scattering caused by larger particles, which Rayleigh cannot adequately explain. As such, it is a valuable addition to the discourse on light scattering in diverse scientific areas.
Unique Feature: The primary advantage of Mie scattering is its application to various fields, including optics, remote sensing, and meteorology. However, its complexity can challenge those not familiar with advanced mathematical models. Overall, Mie scattering complements Rayleigh scattering, providing a more rounded understanding of light-matter interactions.
Role in Atmospheric Science
In atmospheric science, Mie scattering plays a critical role in interpreting data about aerosols and particulates in the air. This scattering mechanism allows scientists to analyze how light interacts with clouds and other atmospheric particles. Its key characteristic leans on its ability to integrate data from various particle sizes, facilitating comprehensive atmospheric models.
Unique Feature: Furthermore, Mie scattering supports technologies like LIDAR and remote sensing, vital for studying climate change. Though the modeling can be complex, the resulting data is essential for environmental monitoring and research. As such, it reinforces the importance of understanding diverse scattering mechanisms in tackling atmospheric challenges.
Types of Scattering
The topic of types of scattering is fundamental in understanding the various phenomena associated with light interaction with matter. Scattering is not merely a simple event, but a complex interplay that influences our perception of the world. Recognizing the different types of scattering—elastic and inelastic, specular and diffuse—can significantly enhance our comprehension of light behavior in various environments. Their implications stretch across numerous scientific disciplines, making this topic essential for students, researchers, and professionals alike.
Elastic vs. Inelastic Scattering
In the realm of light scattering, distinguishing between elastic and inelastic scattering is crucial. Elastic scattering occurs when light interacts with particles, and its energy and wavelength remain unchanged. This means that the frequency of the scattered light remains the same as the incoming light. One of the most common examples of elastic scattering is Rayleigh scattering, where smaller particles cause the scattering of light without altering its energy. This phenomenon explains why the sky appears blue during the day.
On the other hand, inelastic scattering involves a change in the wavelength of the light as it interacts with matter. This change can occur because energy is absorbed from the light by the particles, leading to a longer or shorter wavelength in the scattered light. The well-known phenomenon of Raman scattering falls under this category, which has applications in chemical analysis and material characterization. Both types of scattering play pivotal roles in diverse fields, influencing everything from atmospheric studies to analytical medicine.
Specular and Diffuse Scattering
Another key distinction in scattering is between specular and diffuse scattering. Specular scattering happens when light reflects off a smooth surface in a single, well-defined direction. This is similar to how light behaves when reflecting from a mirror. The smoothness of the surface is crucial; the more polished the surface, the more pronounced the specular reflection. This type of scattering is vital in fields such as optical design and engineering where precise light control is necessary.
In contrast, diffuse scattering lacks a specific direction and occurs when light interacts with rough surfaces or irregular particles. The irregularities in the surface scatter light in multiple directions, making it less predictable compared to specular scattering. This kind of scattering is often observed in materials like paper or concrete, where the surface does not reflect light uniformly. The understanding of diffuse scattering is essential in areas like photography and color science, where surface texture significantly alters visual perception.
"Understanding the types of scattering is key to harnessing light's properties across multiple domains, from environmental science to advanced imaging techniques."
Applications of Light Scattering
The field of light scattering extends beyond mere theoretical understandings; it carries significant weight in practical applications across various domains. Its relevance can be seen explicitly in environmental sciences, industrial processes, and biomedical fields. Each application showcases how the principles of light scattering not only enhance research but also contribute to advancing technologies that benefit society. Understanding these applications helps unravel the various benefits and challenges that light scattering can present, providing insights for future advancements and improvements.
Environmental Monitoring
Air Quality Assessment
Air quality assessment is critical for evaluting the health of the environment and the safety of human activities. Through light scattering techniques, particles and pollutants within the air can be precisely measured, providing essential data on air quality. This technique is particularly important as it allows for real-time monitoring of harmful substances such as PM2.5 and ozone levels.
The key characteristic of air quality assessment through light scattering lies in its accuracy and efficiency. The use of instruments that capture scattered light enables scientists to analyze particulates swiftly and accurately. Therefore, it is a favored choice for environmental studies and governmental regulations.
A unique feature of air quality assessment using this method is its non-invasive nature. Unlike traditional sampling methods, light scattering provides immediate insights without the need for extensive preparation. However, one disadvantage is that varying humidity and temperature can affect scattering results, complicating data interpretation in certain contexts.
Water Quality Analysis
Water quality analysis plays a vital role in environmental management, ensuring safe drinking water and healthy ecosystems. Light scattering techniques are invaluable in detecting and evaluating suspended particles and microorganisms in water. This method can identify contaminants quickly, which is essential for timely responses to water pollution incidents.
The key characteristic of water quality analysis through light scattering is its ability to measure a wide range of substances in real-time. This adaptability makes it a popular choice in laboratories and field studies. The unique feature here is the ability to provide fast feedback loops, which aids in managing water treatment processes effectively.
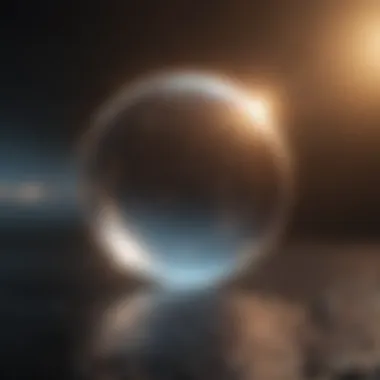
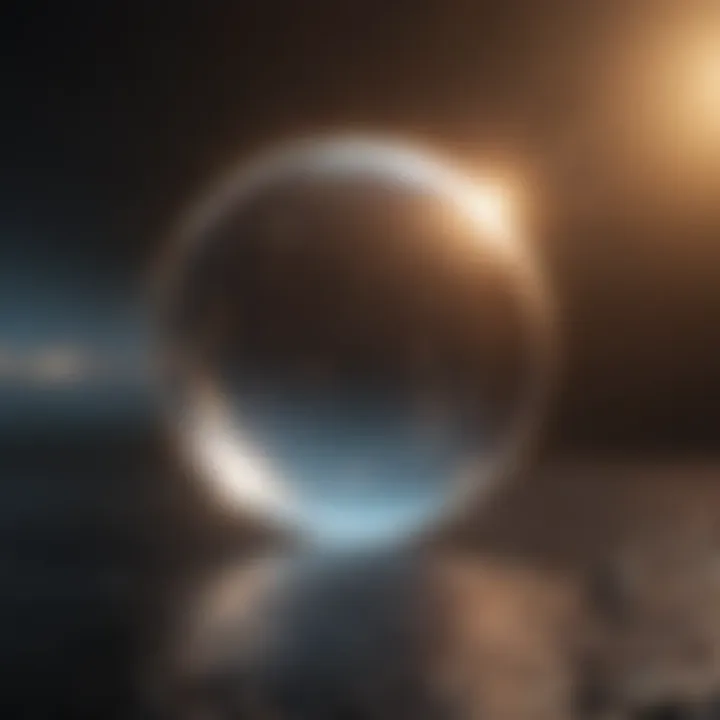
However, one concern with water quality analysis through light scattering is sensitivity to changes in water chemistry, which may yield false positives or negatives. Careful calibration and validation against established methods are essential to mitigate this challenge.
Industrial Applications
Material Characterization
Material characterization involves determining the properties of materials based on their interaction with light, including how they scatter it. This technique is widely adopted, particularly in the field of nanotechnology, where understanding particle size and distribution is crucial.
The primary importance of material characterization is its precision in identifying material properties. Using light scattering, researchers can analyze nanomaterials with exceptional sensitivity and resolution. This benefit makes it an attractive option in research and development, especially for innovative materials.
A unique attribute of this characterization technique is its capacity for online monitoring in industrial settings. This allows for immediate adjustments in manufacturing processes based on real-time data. However, the complexity of interpreting scattering patterns can be challenging, necessitating advanced modeling and data analysis techniques.
Quality Control in Manufacturing
Quality control in manufacturing is critical to ensure products meet specified standards and regulations. Light scattering methods are employed to monitor the uniformity and quality of products in production lines, providing a continuous assessment of material properties.
A key characteristic of using light scattering for quality control is its ability to function as a non-destructive testing method. This aspect ensures that products remain intact while undergoing testing, preserving their usability. It has become a favored choice among industries seeking efficiency without compromising product integrity.
The unique advantage of quality control through this method is the ability to analyze products in real time, allowing manufacturers to react swiftly to any inconsistencies. Nonetheless, potential variability in measurements can arise due to environmental factors, making regular calibration a necessity to ensure reliability.
Biomedical Applications
Diagnostic Imaging
Diagnostic imaging is a crucial aspect of modern healthcare, enabling non-invasive observation of biological tissues. Light scattering techniques, particularly optical coherence tomography, allow for detailed imaging of internal structures with high resolution.
The essence of diagnostic imaging using light scattering lies in its ability to provide real-time feedback during medical examinations. This approach enhances visualization in a clinical environment, making it a highly effective choice for diagnostics.
A significant feature of this method is the capacity for early disease detection. The non-invasive characteristics facilitate patient comfort while allowing physicians to gather essential information efficiently. However, a limitation is that specialized equipment is often costly, creating barriers for wider adoption in some healthcare settings.
Therapeutic Applications
Therapeutic applications of light scattering are emerging as innovative techniques in treatment modalities, such as phototherapy and targeted drug delivery systems. These applications exploit the scattering of light to deliver energy or enhance the absorption of therapeutic agents within specific tissues.
The primary importance of therapeutic applications lies in their specificity and effectiveness in targeting affected areas. This enables higher treatment efficacy while minimizing side effects, making these methods particularly appealing.
A unique characteristic of therapeutic applications is the potential for personalization. Treatments can be tailored based on individual patient characteristics, thus promoting better outcomes. However, challenges remain in consistently delivering precise dosages in complex biological environments, requiring ongoing research to optimize protocols.
Light Scattering in Nature
The discussion of light scattering in nature is significant because it reveals the interaction between light and matter in various environments. Understanding these processes can enhance our comprehension of natural phenomena such as atmospheric conditions and biological functions. Through the mechanisms of light scattering, we can gain insights into how light influences perception, ecology, and communication among living organisms. This section explores the critical roles light scattering plays in color perception and biological significance, highlighting its benefits and implications.
Role in Color Perception
Scattering in the Atmosphere
Scattering in the atmosphere is a fundamental phenomenon that occurs when sunlight interacts with particles and molecules present in the air. This process contributes significantly to the colors we see in the sky, particularly during sunrise and sunset when the light path is longer. The dominant scattering mechanism here is called Rayleigh scattering, which predominates for shorter wavelengths of light.
A key characteristic of scattering in the atmosphere is that it produces blue skies and vibrant sunsets. This is a beneficial aspect for the article, as it introduces visual phenomena that directly affect human experience and artistic inspiration.
The unique feature of this scattering is its wavelength dependency. Blue light, which has a shorter wavelength, scatters more than red light when it passes through air molecules. This results in daytime skies appearing blue, while longer wavelengths dominate at sunset. However, factors such as pollution or weather conditions can vary these effects and change color perception. Therefore, the advantages and disadvantages of scattering in the atmosphere extend beyond simple aesthetic appeal to involve environmental considerations as well.
Effect of Particles
The effect of particles in light scattering is crucial in understanding various environmental and biological systems. Particles of different sizes and compositions can lead to different scattering effects, impacting how light interacts with the atmosphere, bodies of water, and biological organisms. Key characteristics of this effect include the varying impact of different particle types, such as aerosols or biological matter.
This aspect is beneficial because it aids scientists in tracking various elements like air pollution or marine biology. It provides insights into how these particles absorb and scatter light, thus affecting visibility and health standards.
A unique feature of this effect lies in its dual nature: while fine particles can lead to clearer skies, larger particles often lead to hazy or obscured conditions. Hence, understanding the effects of particles means striking a balance between their contributions to ecological processes and their potential detriments to human health.
Biological Significance
Photosynthesis
Photosynthesis is a critical biological process where light energy is captured and transformed into chemical energy by plants and certain microorganisms. This process utilizes visible light, primarily absorbed by chlorophyll, and is essential for sustaining life on Earth. The importance of light scattering comes into play here, as it affects light availability for photosynthesis, especially in aquatic environments.
The key characteristic of photosynthesis in relation to light scattering is its dependence on light intensity and quality. This makes it a beneficial process for the article, highlighting energy conversion's pivotal role in ecology and agriculture.
A unique feature of photosynthesis is that it can adapt to varying light conditions through mechanisms like photoprotection. However, excessive scattering of light can inhibit photosynthetic efficiency, especially in densely grown vegetation or polluted waters, thus revealing both advantageous and limiting factors.
Animal Communication
Animal communication refers to the various ways in which animals convey information to one another, often through visual signals that depend on light. Many species have adapted to use color and brightness variations for signaling, which are influenced significantly by light scattering.
A key characteristic here is the use of specific colors that result from light scattering, allowing animals to communicate effectively. This makes the study of animal communication a beneficial aspect for this article, demonstrating how light scattering influences behavior and social dynamics.
The unique feature of animal communication is its complexity. It often involves multiple layers of information conveyed through varied signals, such as brightness, color, and movement. While this enhances interaction among species, it can also lead to miscommunication in certain environments, particularly where light conditions are fluctuating.
In summary, the role of light scattering in nature is essential for understanding environmental and biological processes. From shaping color perceptions to facilitating vital ecological interactions, it remains an integral part of our understanding. The implications extend beyond mere observation, influencing scientific research in various domains.
Measurement Techniques
Measurement techniques are pivotal in the study of light scattering as they allow scientists to quantify and analyze the scattered light effectively. An accurate measurement can provide insights into the properties of scattering particles and their interactions with light. The choice of technique often hinges on the specific application and the nature of the samples involved. Some techniques can provide non-invasive means of analysis, while others may require more elaborate setups. Each method comes with advantages and disadvantages that must be carefully considered in the context of research objectives.
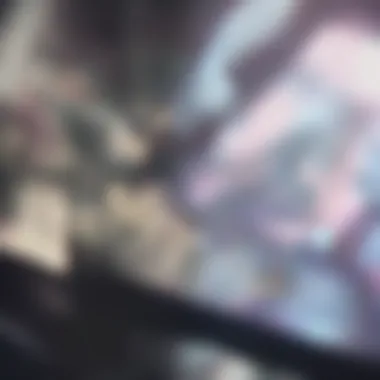
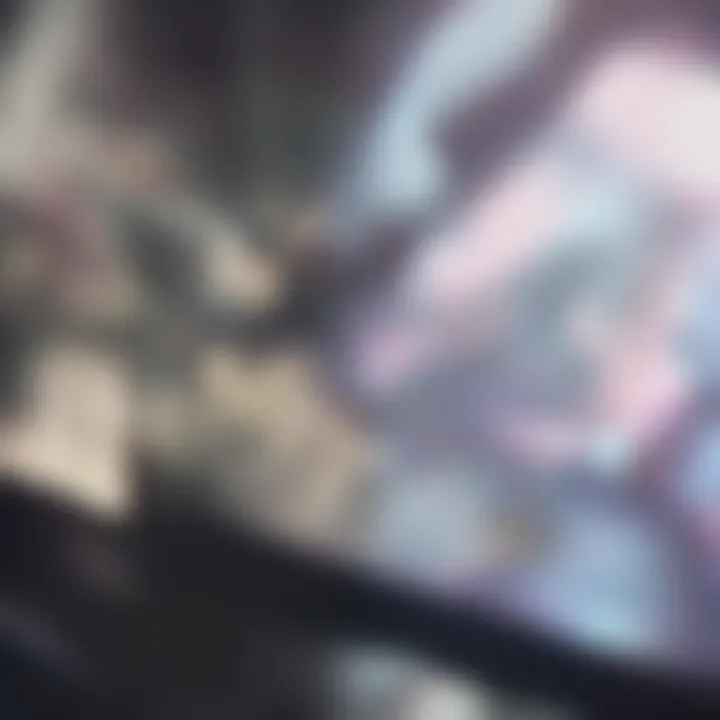
Instruments for Scattering Analysis
Spectrophotometers
Spectrophotometers are instrumental devices that measure the intensity of light at various wavelengths as it passes through a sample. They are very useful in analyzing the absorbance and transmission characteristics of a material, giving critical data about the sample’s composition based on how light interacts with it.
One key characteristic of spectrophotometers is their ability to operate in a wide range of wavelengths, from ultraviolet to infrared. This makes them a popular choice in studies of light scattering as they enable researchers to capture comprehensive spectral data.
A unique feature of these devices is their high sensitivity, which facilitates the detection of low-concentration samples. However, they can also have limitations. For example, they might struggle to accurately measure samples with high turbidity or those that scatter light too broadly. Nevertheless, in many applications related to light scattering, spectrophotometers offer a reliable and effective means of analysis.
DLS Techniques
Dynamic Light Scattering (DLS) techniques focus on measuring the fluctuations in intensity of scattered light from particles suspended in a fluid. This method is advantageous in determining the size distribution of small particles (typically in the nanometer to micron range) in suspension.
A distinguishing attribute of DLS is that it provides rapid analyses, often yielding results in just seconds. This is particularly beneficial when dealing with multiple samples in a short time frame.
However, DLS techniques also come with their own set of challenges. For instance, they may have difficulties with samples that contain polydisperse mixtures or particles that are aggregates, leading to inaccurate size calculations. Despite these limitations, DLS remains a widely used method for light scattering analysis in various fields, including material science and biology.
Data Interpretation Methods
Data interpretation methods are crucial for translating raw data collected from measurement techniques into meaningful insights. Successful interpretation hinges on a clear understanding of the scattering phenomena and the specific context of the analysis.
Researchers must consider various factors, such as the model of scattering employed and the underlying assumptions made during the analysis. Techniques such as regression analysis, statistical modeling, and machine learning methods are utilized to derive conclusions from scattered light data. These methods help in distinguishing between noise and significant signals, ultimately leading to a better understanding of light scattering in practical applications.
Challenges and Limitations
The study of light scattering presents several challenges and limitations that are crucial for researchers and professionals alike. Understanding these impediments is essential not only for the advancement of scientific knowledge but also for the development of practical applications across various fields. Here, we will examine two significant aspects: the complexity of scattering phenomena and the challenges associated with modeling and simulations.
Complexity of Scattering Phenomena
Light scattering is a multifaceted phenomenon influenced by a variety of factors, including the size, shape, and composition of particles, as well as the wavelength of light. The intricate nature of scattering leads to significant variability in results, complicating the interpretation of data.
- Diverse Particle Sizes: Scattering behavior can differ substantially between microscopic and macroscopic particles. For example, Rayleigh scattering predominates for smaller particles, while Mie scattering is important for larger ones. This variance must be taken into account when analyzing experimental results and applying theoretical models.
- Environmental Factors: The surrounding medium can also affect scattering. Temperature, pressure, and humidity may influence the interactions between light and particles. Such environmental variability can lead to inconsistencies in measurements, making it difficult to obtain accurate and reliable data.
- Scattering Mechanisms: Different mechanisms of scattering may dominate under different conditions. Understanding which mechanism is relevant at a given time requires detailed knowledge and understanding, which is not always feasible.
"The complexity of scattering phenomena fundamentally shapes the way scientists approach data collection and analysis. An appreciation of this intricacy is vital for drawing valid conclusions."
Modeling and Simulation Challenges
The ability to model and simulate light scattering accurately is paramount for predicting real-world behavior. However, several challenges require attention.
- Mathematical Complexity: The equations governing light scattering are often highly complex, requiring substantial computational resources to solve. This mathematical intricacy can hinder the progress of research, as scientists may be limited by available technology or expertise.
- Parameter Selection: Choosing the right parameters for modeling is another significant challenge. The selection process requires a deep understanding of the system being studied, and mistakes can lead to inaccurate predictions.
- Validation of Models: Models developed need rigorous validation against experimental data. However, due to the variability inherent in scattering phenomena, achieving reliable validation is not always possible. Discrepancies between models and experimental results can lead to uncertainty in conclusions drawn from research.
Due to these challenges, ongoing research is critical to improve our understanding and techniques related to light scattering. Addressing these limitations will enable advancements in fields such as material science, environmental monitoring, and biomedical applications.
Future Directions in Research
Research on light scattering continues to evolve, opening avenues for enhanced understanding and applications in multiple fields. Moving forward, it is imperative to address the emerging trends that can reshape existing knowledge and practices. New measurement technologies and interdisciplinary approaches will play a significant role in this progression. These elements not only enhance our comprehension but also provide practical benefits across various domains, from environmental science to biomedical technologies.
Advancements in Measurement Technologies
Recent advances in measurement technologies are transforming the way researchers analyze light scattering. Instruments that utilize advanced spectrophotometry and dynamic light scattering (DLS) provide greater accuracy and sensitivity. These improvements facilitate the detection of smaller particles and enhance our understanding of scattering phenomena under diverse conditions.
Innovative techniques utilize lasers, allowing for more precise measurements of scattered light. For example, the integration of nanotechnology with scattering methods has enabled researchers to explore light-matter interactions on a nanoscale level. This capability leads to significant enhancements in applications like drug delivery systems in biomedicine, as well as real-time monitoring of environmental pollutants.
Interdisciplinary Approaches
Interdisciplinary approaches are crucial for advancing research in light scattering. Collaboration among physicists, chemists, biologists, and environmental scientists fosters a more comprehensive understanding of scattering processes. By combining different scientific perspectives, researchers can develop more robust models to explain complex phenomena.
For instance, employing insights from physics can enhance our comprehension of particle behavior in scattered light, while biological insights can inform the medical applications of scattering techniques. Moreover, connecting materials science with scattering research can improve industrial applications, leading to innovations in quality control and product development.
The integration of diverse disciplines not only enhances theoretical frameworks but also addresses practical challenges in application. As research on light scattering continues to advance, these interdisciplinary collaborations will be vital in realizing the full potential of this significant scientific phenomenon.
"Innovations in measurement technologies and the blending of disciplines are pivotal for the future of light scattering research."
Culmination
The conclusion serves as a vital segment of this article, synthesizing the key insights presented throughout the discussion on light scattering. In essence, it encapsulates the major themes and findings regarding the principles, mechanisms, and applications of light scattering. Light scattering, involving both Rayleigh and Mie mechanisms, is not merely an abstract physical principle but a topic that intersects various fields, from environmental science to industrial applications.
Summary of Key Findings
In our exploration, we have identified several crucial points:
- Principles of Light Scattering: The article discussed the fundamental principles that govern light scattering phenomena, focusing on the differences and implications of Rayleigh and Mie scattering.
- Mechanisms: We delved into mechanisms that explain how light interacts with various particles and materials in different environments.
- Applications: The real-world applications underline the importance of light scattering in multiple domains:
- Meteorology: Understanding weather patterns and atmospheric conditions through scattering insights.
- Material Science: Quality control and characterization of materials based on scattering data.
- Biomedical Fields: Diagnostic and therapeutic techniques employing scatter-based methodologies.
These findings highlight the multifaceted nature of scattering, revealing its role not just as a scientific phenomenon but as an integral aspect of technological development and environmental assessments.
Impact on Scientific Understanding
The implications of our findings have far-reaching significance. By grasping how light scatters, we unlock deeper insights into the natural world. This knowledge informs scientific inquiry and fosters innovations across disciplines. For example:
- Environmental Monitoring: Enhanced detection of pollutants and assessment of air and water quality.
- Industrial Efficiency: Improved material properties and production processes leading to better performance and sustainability.
- Medical Advances: Innovations in imaging techniques providing clearer diagnoses and treatment pathways.
As research continues into the complexities of light scattering, we can expect more refined methodologies that will further advance our understanding in both existing applications and emerging fields. The appreciation of these principles fosters a comprehensive scientific literacy, enabling professionals and researchers to apply this knowledge efficiently in their fields.
"Understanding the scattering of light not only illuminates the path of scientific inquiry but also spans practical implications across various essential sectors."
As students, educators, and professionals engage with these concepts, the continuing exploration of light scattering will undoubtedly enhance our collective comprehension of the universe.