Understanding Luminescence Measurement Techniques
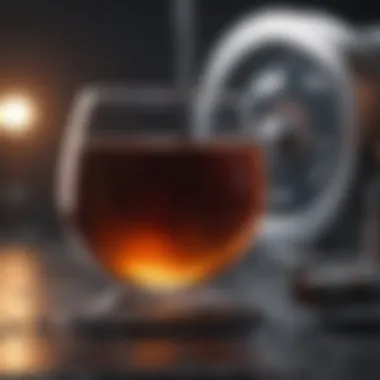
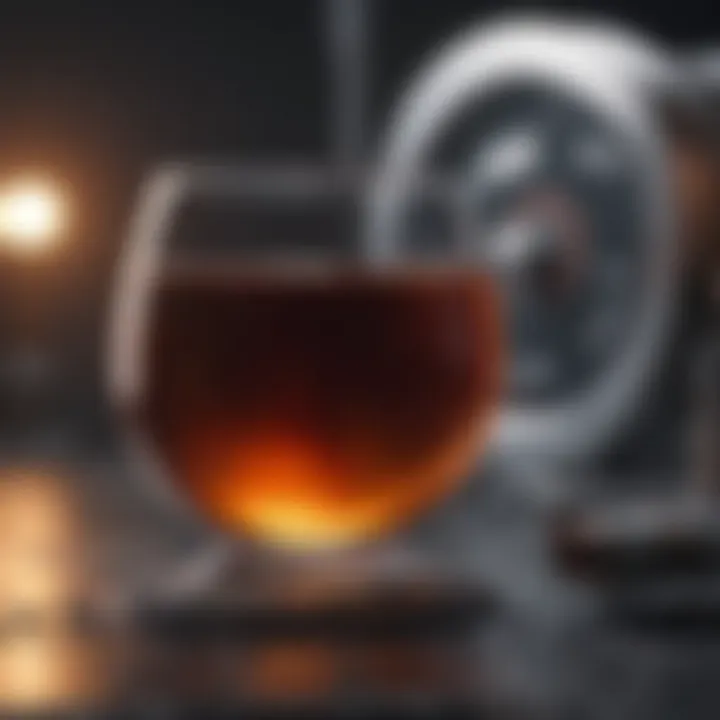
Intro
Luminescence measurement offers a fascinating avenue through which one can investigate various phenomena across many scientific disciplines. From chemistry to environmental science, the ability to detect and measure luminescence allows researchers, educators, and professionals to gain deeper insights into the materials and reactions they study. In essence, luminescence refers to the emission of light by a substance not resulting from heat; this can occur from processes such as fluorescence, phosphorescence, and chemiluminescence.
Understanding luminescence measurement is paramount not only for fundamental research but also for practical applications in industries such as healthcare, material science, and environmental monitoring. Through precise measurement techniques, researchers can glean vital information about chemical reactions, biological processes, and environmental conditions, thereby enhancing our ability to manipulate and interact with the world around us. As we delve into this intricate field, it is crucial to explore its principles, methodologies, and the pivotal roles it plays in advancing scientific knowledge.
Research Overview
Key Findings
Recent studies in luminescence measurement highlight that:
- The sensitivity of luminescence techniques allows for the detection of low concentrations of analytes.
- Innovative methodologies, such as hybrid systems that combine photonic and electronic detection, are on the rise.
- Applications in environmental science, particularly water quality assessment, are gaining traction, whereby luminescence indicates the presence of pollutants.
Study Methodology
The research in luminescence measurement often employs both empirical and theoretical approaches. Common methodologies include:
- Spectroscopic techniques which involve analyzing the emitted light's spectrum to identify compounds and measure concentrations.
- Time-resolved luminescence, providing insights into energy transfer processes and lifetimes of excited states.
- Imaging methods for spatial distribution analysis of luminescent materials.
Background and Context
Historical Background
The study of luminescence has roots going back centuries, with early observations noted as far back as ancient civilizations. The development of modern techniques began in the late 19th century when scientists like Henri Becquerel and Marie Curie explored radioactivity's luminescent properties. Their pioneering work laid the groundwork for understanding how various forms of light emission could be harnessed for measurement purposes.
Current Trends in the Field
In recent years, researchers are setting their sights on several trends within luminescence measurement:
- Miniaturization of detection devices: Smaller, portable equipment is making real-time monitoring feasible in various settings.
- Integration with nanotechnology: Nanoparticles are being utilized to enhance luminescent properties, significantly improving sensitivity and selectivity.
- The impact of machine learning: Advanced algorithms are revolutionizing data analysis in luminescence measurements, enhancing both speed and accuracy.
Luminescence measurement is a versatile tool applicable across multiple domains, presenting new horizons in scientific research and commercial applications.
Closure
As we continue to unlock the mysteries of luminescence, it becomes increasingly important to disseminate knowledge about its measurement methods and applications. This understanding not only enhances academic research but also drives innovation and efficacy across various industries. Therefore, delving into the core principles and newly developed techniques will pave the way for future advancements in this intriguing area of science.
Understanding Luminescence
Luminescence measurement is a fascinating area that combines science and technology to decipher the beauty and complexity of light emission. Understanding luminescence is pivotal, as it provides a foundation for grasping how materials emit light through various mechanisms. This section explains the significance of luminescence and lays the groundwork for subsequent discussions on its principles, techniques, and applications.
This understanding can drive advancements across scientific fields—be it chemistry, biology, or environmental science—where examining light emission can lead to groundbreaking discoveries. Luminescence can illuminate the hidden features of materials and biological systems, making it invaluable for researchers and practitioners. It is the linchpin that links fundamental concepts with real-world applications, thereby revealing new paths for exploration in science and industry.
Definition and Types
Fluorescence
Fluorescence refers to the phenomenon where a substance absorbs light or another form of electromagnetic radiation and then re-emits light at a longer wavelength. A key characteristic of fluorescence is its rapid response time; the emitted light ceases almost immediately after the excitation source is removed, typically within nanoseconds. This quality makes fluorescence a popular choice for a myriad of applications, particularly in molecular biology for techniques such as fluorescence microscopy or flow cytometry.
One unique feature of fluorescence is that it can be analyzed in real-time, making it highly advantageous for studying dynamic processes. However, a disadvantage is that fluorescence may be sensitive to environmental factors, such as pH or temperature, which can interfere with accurate measurements.
Phosphorescence
Phosphorescence is similar to fluorescence but stands apart due to its prolonged emission of light. In this case, excited electrons take a detour through a triplet state before returning to the ground state, which extends the duration of emitted light from microseconds to hours, and sometimes even longer. This characteristic makes phosphorescence highly beneficial for applications like glow-in-the-dark materials or safety signage.
A unique feature of phosphorescence includes its persistence in emitting light, which can continue for an extended time after the excitation source is gone. On the flip side, this delayed emission means that phosphorescent materials can be less efficient in high-intensity applications where immediate light return is critical.
Chemiluminescence
Chemiluminescence stands out as it refers to the production of light as a result of a chemical reaction, without the need for external electromagnetic radiation. This uniqueness in its source of light emission makes chemiluminescence particularly interesting for applications in biochemistry, such as in luciferase assays for detecting biomolecules.
The key characteristic of chemiluminescence is its inherent efficiency, as it can produce bright light with minimal energy input. However, chemiluminescent reactions can be more complex to control and may not provide as stable or reproducible results, particularly when compared to fluorescence or phosphorescence.
The Science Behind Luminescence
Excitation and Emission Processes
At the heart of luminescence are excitation and emission processes. When a molecule absorbs energy from an external source, electrons are excited to higher energy levels. Upon returning to their ground state, these electrons emit light. This process of excitation and emission is fundamental in distinguishing different types of luminescence. Many consider this duality a critical aspect of the luminescence phenomenon, as it provides insights into the energy distribution within materials and influences the technique selection based on the desired application.
A unique aspect is its dependence on the type of material and the wavelength of light used for excitation. Fluorescence depends on lower energy transitions, whereas phosphorescence involves more complicated processes. The challenge lies in optimizing the excitation wavelengths to maximize efficiency depending on the application, such as in clinical diagnostics or environmental monitoring.
Energy Levels and Transitions
The concept of energy levels and transitions is essential for understanding luminescence. At its core, this involves the quantized states of electrons within an atom or molecule. When electrons transition between these energy levels, they emit or absorb light, thus giving rise to various luminescent phenomena.
The unique feature here is that transitions may vary in their nature- whether they are radiative (resulting in light emission) or non-radiative (where energy is dissipated as heat). This aspect is crucial as it determines the efficiency of luminescence in different systems and can guide researchers in selecting materials for applications such as LED development or biological assays.
Decay Time
Decay time is a fundamental parameter in luminescence measurements, referring to the time it takes for emitted light to decrease to a certain fraction of its initial intensity after excitation. This characteristic provides pivotal information about the nature of the luminescent material. For instance, longer decay times in phosphorescent materials can signify stronger intermolecular interactions or a more complex energy transfer pathway.
The unique feature about decay time is that it allows differentiation between fluorescence, phosphorescence, and chemiluminescence. Each type has distinct decay time profiles, and this can be an advantage in applications such as time-resolved spectroscopy, which helps in studying dynamic processes in materials or biological systems.
In summary, understanding luminescence not only encompasses the various forms it can take but also the principles underlying these processes. Each type of luminescence offers unique advantages and challenges that must be considered in increasing the precision and application scope of measurement techniques.
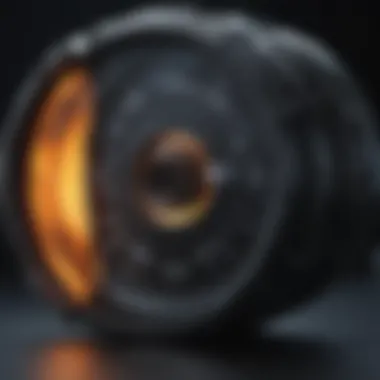
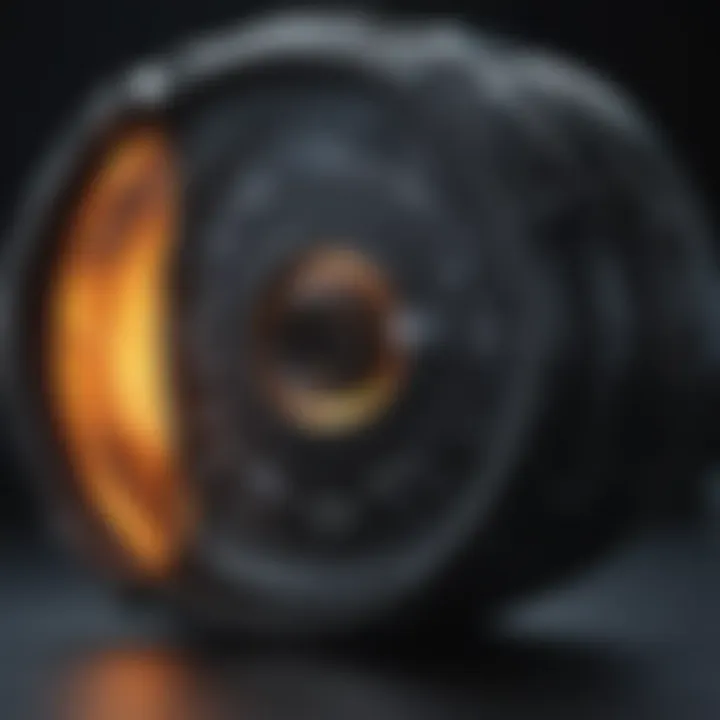
Historical Context
The field of luminescence measurement has evolved remarkably over the centuries. Understanding the historical context not only sheds light on how we’ve arrived at our current methodologies, but it also emphasizes the milestones that shaped the practices we now take for granted. This section aims to unpack the pivotal moments and contributions that have stamped the importance of luminescence on the scientific map.
Early Discoveries
Initial Observations
In the late 16th century, scientists began to take notice of luminescent materials, particularly the unique behaviors displayed by certain minerals under various light sources. These initial observations laid the groundwork for rigorous scientific inquiry into luminescence. For instance, the peculiar property of strontium aluminate, which glows in the dark after exposure to light, garnered attention much before the scientific explanations were formulated. The key characteristic here is that these early practitioners relied heavily on visible phenomena without advanced technology.
This intrinsic curiosity not only advanced initial theories but also highlighted the fundamental connection between chemistry and light, which proves to be a vital aspect for this article. What truly stands out about these observations is their character: a blend of inquiry and excitement. While these investigations were often tentative and rudimentary, they opened up a treasure trove of possibilities.
The unique feature of these observations lies in their qualitative nature; they sparked deeper explorations into light-matter interactions. Though challenges were aplenty, such as limited tools and resources, this period was, without doubt, a springboard for future advancements, making it a worthy mention in this article.
Key Contributors
Notable figures such as Sir Isaac Newton and Michael Faraday made significant contributions during the initial phase of luminescence measurement. Newton's color spectrum experiments illustrated the interaction of light with matter, laying foundational principles for understanding luminescence. Faraday further expanded on this by exploring electrochemistry’s effects on luminescent materials, highlighting unique behaviors observed under different conditions.
Key characteristic of these contributors is their interdisciplinary approach. By combining physics and chemistry, they provided comprehensive frameworks that informed future research. Their work made it easier for others to step into this field and build upon their findings, thereby enriching the scientific community's understanding of luminescence.
One unique aspect of these early contributors was their ability to merge observation with theory. They weren’t just seeing glowing substances; they were trying to explain the why and how. Although their concepts were in nascent stages, the frameworks they established paved the way for many modern applications.
Evolution of Measurement Techniques
From Simple Methods to Advanced Technologies
The progress from rudimentary measurements to sophisticated techniques marks a significant trajectory in luminescence measurement. At first, methods mostly relied on simple visual assessments—after all, how bright a material appeared was often all the information researchers had. Early practitioners used basic glass prisms and filters to analyze light emitted by luminescent substances. Their findings were often confined to qualitative assessments.
However, as the quest for precision intensified, so did the invention of more refined methodologies. The emergence of spectrometers transformed this landscape. Spectroscopy allowed researchers to not just observe but quantify luminescence, making it easier to analyze differences in intensity, wavelength, and decay rates. This transition is a beneficial shift for the narrative of luminescence measurement.
A standout trait of this evolution is its adaptability: as scientific needs grew complex, measurement techniques evolved to meet those demands. This development also underscores the importance of collaboration among various scientific fields. Yet, this shift wasn't without its drawbacks; increased complexity often meant higher costs and the need for specialized training.
Influence of Technological Advances
The technological revolution drastically influenced luminescence measurement, nudging the field into realms previously unimagined. The transition from analog to digital techniques is a telling example. The SPCM (Single Photon Counting Module) and modern CCD (Charge-Coupled Device) sensors are prime contenders that have drastically improved sensitivity and resolution.
The key characteristic of this influence is how it democratized luminescence measurement. As devices became smaller, cheaper, and user-friendly, even smaller labs and universities could measure luminescence accurately, thus broadening its applications. Not only did this surge of technology benefit established industries—like biomedical research and in materials science—but it also drew in budding scientists and innovators who previously lacked access to such sophisticated methods.
One notable upside is that technological advances have allowed for high-throughput screening of luminescent compounds. However, with advancement comes a bumpy road. Relatively higher learning curves and the potential for technology-induced errors signify challenges that accompany new tools.
"Understanding the history of luminescence measurement not only elevates our comprehension but cements its relevance across scientific fields."
Measurement Techniques
Understanding measurement techniques in luminescence is crucial for both accuracy and application in various scientific fields. These techniques not only enhance our understanding of light emission properties but also serve as the backbone for many research endeavors. Choosing the right measurement method can greatly influence the results and interpretations derived from luminescence phenomena.
Spectroscopy Methods
Spectroscopy methods are pivotal in examining luminescence, providing detailed insights into the energy levels and transitions involved. Each technique within spectroscopy offers unique advantages and nuanced perspectives on luminescent behaviors.
UV-Vis Spectroscopy
UV-Vis spectroscopy deals with the ultraviolet and visible light regions. This method allows researchers to explore absorbance and emission properties effectively. One of its key characteristics is its ability to offer broad spectral information which often leads to insights about molecular structures.
- Advantages: It is widely used owing to its simplicity and cost-effectiveness. Researchers can quickly gather quantitative data on sample concentrations.
- Disadvantages: However, it might not be as sensitive to some luminescent compounds compared with other methods.
The unique feature of UV-Vis spectroscopy lies in its accessibility; many laboratories are equipped with UV-Vis spectrophotometers, making it a popular choice among chemists and biologists alike.
Fluorescence Spectroscopy
Fluorescence spectroscopy stands out for its sensitivity to low concentrations of analytes. It plays a vital role in tracking dynamic processes within cells or other mediums. This technique is characterized by its high signal-to-noise ratio, enabling detection of even minuscule amounts of luminescent material.
- Advantages: This method is highly beneficial due to its rapid response times and non-destructive nature that preserves sample integrity.
- Disadvantages: However, it can be susceptible to quenching effects that can distort results.
The unique aspect of fluorescence spectroscopy is its versatility. It finds application in studying biochemical interactions, making it invaluable when probing deeper into biological systems.
Photomultiplier Tube Detectors
Photomultiplier tube detectors have been a cornerstone in luminescence measurement. They convert light into an electrial signal, which is useful in low-light environments. The operational principle behind these detectors is fascinating and showcases their effectiveness in a variety of settings.
Operational Principles
The way photomultiplier tubes work is by employing the photoelectric effect. When photons hit a photocathode, they release electrons, which are then multiplied by a series of dynodes, resulting in an amplified current that corresponds to light intensity. This operational detail is crucial because it underpins how we can measure subatomic levels of light emission.
- Advantages: Their high sensitivity allows for detecting extremely dim light sources, which is essential in many experimental settings.
- Disadvantages: The main drawback is their vulnerability to background light and noise, which can skew results.
Applications in Research
In the domain of research, photomultiplier tubes are deployed in various fields including nuclear physics, biochemistry, and environmental monitoring. Their capacity to provide accurate luminescence measurements is indispensable for researchers trying to detect faint light signals.
- Advantages: With their high temporal resolution, they can capture fast luminescent events.
- Disadvantages: They require careful calibration to ensure correct readings, adding complexity to their operation.
Charge-Coupled Device Sensors
Charge-coupled devices (CCDs) have revolutionized luminescence measurement, becoming integral in modern devices. Their design allows for converting light into electronic signals, making them a preferred choice for imaging applications.
Integration in Modern Devices
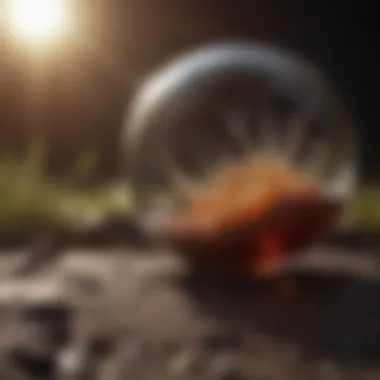
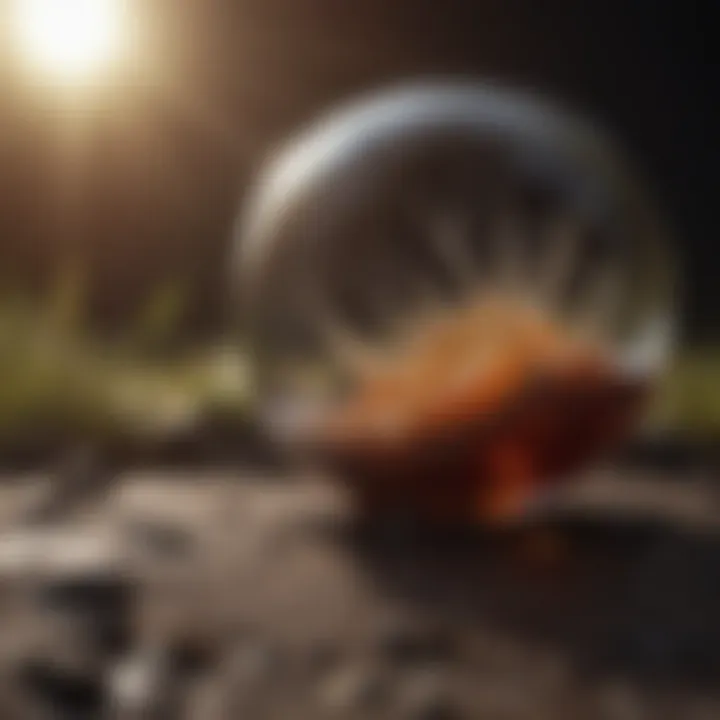
CCDs are often embedded in digital cameras and scientific equipment, showcasing their versatility. Their ability to capture images over a wide range of wavelengths underlines their importance.
- Advantages: The ability to gather a large amount of data quickly makes them very useful in various applications.
- Disadvantages: Noise management can be a concern, and they can be less responsive in low-light conditions compared to other detector types.
Data Acquisition and Analysis
The collection of data from CCDs leads to intricate analysis opportunities. Researchers can process digital images and identify precise luminescent features from different samples.
- Advantages: Their compatibility with complex software enables sophisticated analysis, providing detailed insights into the luminescent properties of samples.
- Disadvantages: The initial investment cost for advanced CCD systems may be higher, which can limit accessibility for some research teams.
Time-Resolved Techniques
Time-resolved techniques offer a window into the dynamics of luminescent processes. They allow investigators to gauge the timing of light emission and absorption, integral for deeper studies of molecular behavior.
Applications in Kinetics Studies
The ability to monitor how quickly luminescence occurs can yield rich information about reaction pathways and mechanisms. This is especially beneficial in examining rapid processes that traditional methods may overlook.
- Advantages: They provide crucial insights into the kinetics of luminescent species, leading to aided understanding of reaction mechanisms.
- Disadvantages: The requirement for advanced equipment and expertise can pose challenges for researchers.
Limitations and Challenges
Time-resolved techniques, while powerful, are not without pitfalls. One of the critical limitations is ensuring that the measured timings are accurate in dynamic systems. Furthermore, complex setups can deter routine adoption.
- Advantages: They provide a powerful tool for understanding transient states in luminescent materials.
- Disadvantages: The complexity of setup and analysis can lead to errors if not executed with precision.
Applications of Luminescence Measurement
The exploration of luminescence measurement reveals a rich tapestry of applications that significantly impact various scientific fields. Understanding how luminescence serves as a diagnostic tool is crucial for researchers striving to unveil complexities within chemistry, biology, and environmental sciences. These applications not only highlight the versatility of luminescence techniques but also underscore their indispensable role in fostering innovation and addressing contemporary challenges.
In Chemistry
Sensory Applications
Sensory applications of luminescence measurement stand out for their ability to detect and quantify various chemical substances at minimal concentrations. This aspect is critical in areas such as environmental monitoring, where detecting pollutants is paramount. The key characteristic of sensory applications is their specificity and sensitivity to different analytes. For instance, chemiluminescent sensors can indicate changes in pH, temperature, or the presence of certain ions in solutions.
One unique feature of sensory applications is the use of luminescent probes that change their emission characteristics upon interacting with target molecules. This allows for real-time monitoring, which is a significant advantage in dynamic systems. However, it's essential to be cognizant of the limitations, like the potential for interference from other substances, which might reduce the accuracy of measurements.
Quantitative Analysis
Quantitative analysis via luminescence measurement plays a pivotal role in determining the concentration of various compounds. This technique offers a non-destructive method for analysis, a beneficial aspect that appeals to many researchers. The key strength of quantitative analysis is its capacity to provide precise measurements over a wide concentration range. Utilizing fluorescence spectroscopy, chemists can accurately identify trace amounts of elements in complex mixtures.
The unique feature of this approach lies in its ability to achieve high levels of sensitivity and selectivity, which is crucial for ensuring reliable results. That said, quantifying substances can sometimes present challenges, particularly when numerous luminophores are present, as overlapping emission spectra can complicate the analysis.
In Biology
Bioluminescent Probes
The use of bioluminescent probes has revolutionized the way scientists explore biological processes. These probes, derived from natural luminescent organisms, serve as powerful tools for tracking molecular interactions within living cells. The key attribute here is the ability of bioluminescence to provide high signal-to-noise ratios, which enhances detection capabilities.
A unique feature of bioluminescent probes is their capability to offer real-time imaging, permitting the observation of dynamic biological processes in vivo without having to resort to invasive methods. This has opened doors for innovative studies in physiology and pathology, although one must be cautious about their stability and potential toxicity, which can influence experimental outcomes.
Cellular Imaging Techniques
Cellular imaging techniques that utilize luminescent indicators represent a frontier in biological research. These techniques allow for the visualization of cellular events at an unprecedented resolution. The main characteristic of these imaging systems is their potential for non-invasive monitoring of cellular processes, making them vastly important in fields like cancer research and drug development.
Notably, the unique feature of cellular imaging is its capacity to combine multiple luminescent tags to visualize several biological markers simultaneously. However, the challenge lies in optimizing these techniques for consistency, as variations in cellular environment can affect luminescence signals and interpretation.
In Environmental Science
Monitoring Pollution Levels
Monitoring pollution levels through luminescence measurement offers an effective way to assess environmental health. The key characteristic of this approach is the ability to quantify contaminants rapidly and accurately, enabling timely interventions. Luminescent assays can identify specific pollutants, helping scientists pinpoint sources of pollution.
A unique feature of monitoring pollution levels with luminescence is the capability to employ portable instruments for field analysis. Nonetheless, this method has its drawbacks; for instance, variability in environmental conditions, such as temperature and light conditions, can affect the reliability of results.
Studying Ecological Impacts
The role of luminescence in studying ecological impacts is becoming increasingly important as we strive to understand the complex relationships within ecosystems. By examining the luminescence of organisms, scientists can gather data regarding environmental stressors, population dynamics, and food web interactions. The key advantage here is the capacity for holistic ecological assessments.
A unique feature is that the bioluminescence of certain species can indicate the health of their habitats. However, this method can be limited by the availability of luminescent organisms, as well as the complexities in extrapolating findings from a single species to broader ecological conclusions.
Industrial Applications
Quality Control Processes
Quality control processes heavily utilize luminescence measurement for ensuring product standards, particularly in pharmaceuticals and materials science. The key feature of these processes is their ability to provide rapid assessment of product quality without damaging the items being tested. Luminometric assays are often faster and more convenient compared to traditional methods, presenting a beneficial aspect that manufacturers find attractive.
One unique feature of using luminescence in quality control is the capability of real-time monitoring during production processes, enabling immediate adjustments. This, however, requires a robust calibration system to ensure accuracy, posing a challenge that industries must overcome.
Development of New Materials
The development of new materials leveraging luminescence measurement is at the cutting edge of material science today. The key characteristic of this approach is the focus on discovering materials with tailored luminescent properties for specific applications, such as phosphorescent pigments or novel LEDs. This ability to engineer materials uniquely positions researchers in advancing technology.
A unique feature of luminescent materials is their versatility in applications ranging from displays to sensors. However, the drawbacks include the complexity of manufacturing processes and the potential for unpredictable performance under different conditions, which can pose setbacks in commercial viability.
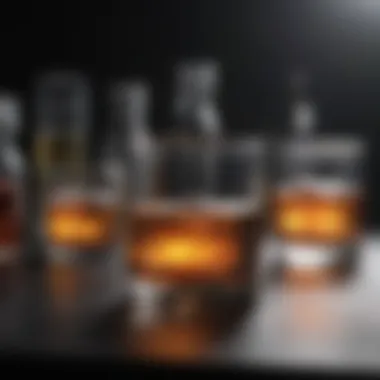
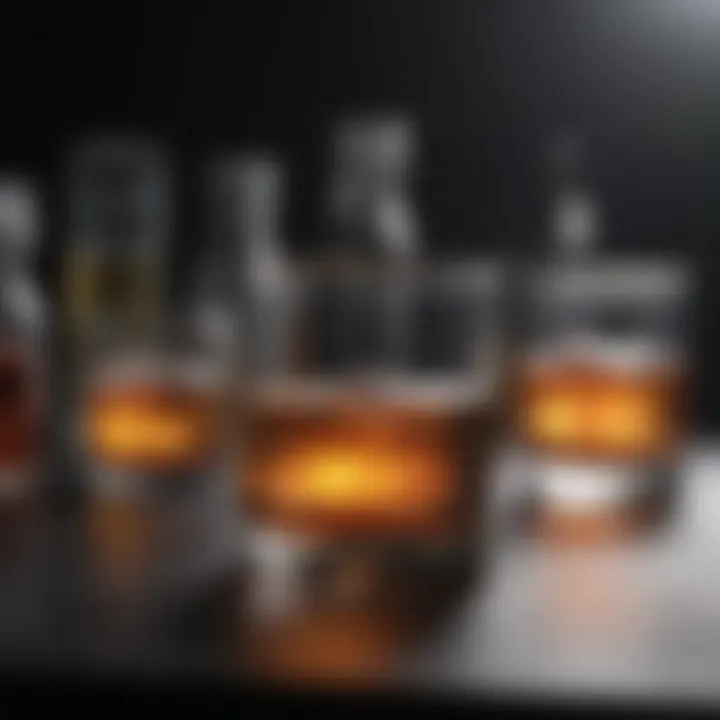
In summary, the applications of luminescence measurement span various fields, revealing profound impacts and offering extensive benefits. From chemistry to industrial use, the implications of these techniques continue to grow, promising further advancements and opportunities for scientific inquiry.
Challenges in Luminescence Measurement
The intricate domain of luminescence measurement is not without its fair share of hurdles. As researchers continue to explore the far-reaching applications of luminescence in various scientific disciplines, understanding the challenges involved becomes crucial. These challenges, particularly the interference from background noise and the calibration issues, can impact the accuracy and reliability of measurements. Addressing these elements is essential for ensuring that luminescence data not only meets the needed standards but also contributes meaningfully to research advancements.
Interference and Noise
Sources of Background Noise
In any measurement process, noise can rear its head like an uninvited guest at a party. The sources of background noise in luminescence measurement can stem from various phenomena, including stray light from the environment, thermal fluctuations, and even the electronic noise inherent in detectors. Each of these factors introduces variability that can compromise the integrity of the results. The key characteristic of these noise sources is their unpredictability, which can distort the readings and complicate data interpretation. This multifaceted nature of noise makes it a popular topic of concern in luminescence discussions, as minimizing its impact is a priority.
A unique feature is that certain background noises, like electromagnetic interference from electronic devices, can be controlled through thoughtful experimental design. However, this requires careful setup and awareness of the surrounding environment, which adds another layer of complexity.
Methods for Minimizing Interference
Tackling interference in luminescence measurement is akin to navigating a maze; it requires strategies that efficiently mitigate unwanted disturbances. Several methods exist for addressing these challenges, such as using optical filters to limit stray light entry, implementing noise-cancellation techniques, or even employing specific electronics to reduce electronic noise. The key characteristic of these methods is their proactive nature; they aim to create a controlled environment in which measurements can be taken with minimal external influences.
However, these methods come with their unique trade-offs. For instance, adding optical filters can sometimes block out desired signal wavelengths along with unwanted noise, potentially leading to a loss of information that could be critical for analysis. Thus, researchers must carefully balance the desire to reduce interference with the need to maintain data integrity.
Calibration Issues
Importance of Standardization
Calibration stands as a backbone for any measurement technique, and luminescence measurement is no different. The importance of standardization lies in its ability to ensure that measurements are reliable, reproducible, and comparable across different studies and instruments. By establishing a consistent framework for calibration, researchers can confidently interpret their data and draw meaningful conclusions.
In essence, a standardized approach helps in solidifying the credibility of luminescence measurement. Its unique feature is that it reinforces the notion of scientific rigor, laying the foundation for future advancements. If calibration techniques are unreliable, the entire body of work derived from those measurements can be called into question, resulting in potentially misguided interpretations.
Strategies for Effective Calibration
When navigating the complexities of calibration, employing effective strategies is key for achieving precise results. Various approaches, such as using certified reference materials or employing a calibration curve derived from known standards, can optimize the calibration process. The key characteristic here is the establishment of a direct correlation between measured values and known standards, which significantly enhances the credibility of the data.
Moreover, one must be cognizant of factors like instrument drift which can occur over time. Regular recalibration ensures that measurements remain accurate throughout experiments. However, this entails additional resources and time, as well as a commitment to continuous assessment of methodology. As researchers weigh their options, the cost-benefit analysis of calibration strategies becomes pivotal in their experimental design.
"Effective calibration is as crucial to reliable data as a sturdy foundation is to a skyscraper."
Future Directions
As research in luminescence measurement continues to expand, so too do the opportunities and challenges that lie ahead. Navigating these future directions is not just a matter of keeping abreast of innovations; it’s about recognizing the implications these innovations have across various fields. With advances in technology and broadened applications, the path forward holds the potential for transformative impacts on both scientific research and practical applications.
Innovations in Technology
Emerging Detection Methods
Emerging detection methods are paving the way for enhanced accuracy and sensitivity in luminescence measurement. For instance, novel approaches such as quantum dots and nanomaterials are becoming more popular due to their ability to yield high-resolution data under a range of conditions. This key characteristic allows researchers to observe processes that were previously hidden from view.
One of the notable aspects of these emerging methods is their adaptability to different experimental setups, which is a significant advantage for many disciplines. The unique feature of these techniques—high photostability—means they can operate without the degradation that affects traditional luminescent materials over time. This reliability is crucial for long-term studies which are often essential in fields like environmental monitoring or biological research. However, while the benefits are substantial, challenges such as the complexity of synthesis and the risk of toxicity in some nanomaterials need to be managed carefully.
Advancements in Photonic Devices
Advancements in photonic devices have emerged as another fulcrum of change in luminescence measurement. These devices, known for their high-efficiency light emission and precise management of photon interactions, can dramatically enhance measurement capabilities. Highly sensitive photodetectors are becoming instrumental in research settings, as they allow for the detection of even the faintest light signals.
The uniqueness of these devices lies in their capacity to operate over a broad spectrum of wavelengths, making them multidisciplinary tools that can be leveraged in varied research fields. The advantage of speed in data collection is particularly appealing for real-time monitoring applications. Nonetheless, the downside is often tied to cost and the specialized training necessary to operate and interpret data from these sophisticated instruments.
Broadening Applications
Integration with Other Scientific Fields
Integration with other scientific fields represents a key horizon for the future of luminescence measurement. Interdisciplinary collaboration fosters innovation, with luminescence tools being applied in realms such as physics, material science, and even social science. This collaborative trend helps build a more holistic understanding of complex phenomena.
The key characteristic of this integration is the cross-pollination of methodologies. By blending techniques from different disciplines, researchers can yield richer data and novel insights. One unique feature of this collaboration is the introduction of luminescence measurements in non-traditional fields, like archaeology, where they help analyze ancient artifacts. The advantage here is the ability to gather precise data that informs historical narratives, but it also raises questions regarding the interpretative paradigm of such applications.
Potential in Healthcare
The potential of luminescence measurement in healthcare is becoming increasingly clear, especially with its applications for diagnostics and therapeutics. By employing luminescent probes in biological systems, researchers can track disease progression in real-time, offering a significant advantage in personalized medicine.
What sets this application apart is the ability to conduct minimally invasive procedures that provide a wealth of information while ensuring patient comfort. The unique attribute of high specificity makes luminescent probes particularly valuable for targeting specific cells or biomarkers. However, balancing these advantages with considerations regarding safety, efficacy, and the socioeconomic implications of such technologies remains paramount.
"The future of luminescence measurement lies not just in its technologies, but in how those technologies will be woven into the very fabric of various scientific inquiries."
Epilogue
In sum, the journey through luminescence measurement lays bare its significance across various realms of science and industry. This article has highlighted the critical role that luminescence plays not just as a phenomenon, but as a tool for research and development. By organizing the subject into coherent sections, we’ve illuminated the layered complexities that define this field and underscored its profound implications for the future.
Synthesis of Key Insights
Importance of Luminescence Measurement
Luminescence measurement stands as a cornerstone in multiple scientific disciplines, combining elements of chemistry, physics, and biology. One of its defining characteristics is its ability to provide real-time data on chemical reactions, biological processes, and environmental changes. By measuring light emissions, scientists can gain insights that might otherwise be obscured. For instance, in the realm of biochemistry, luminescence serves as a convenient proxy to track cellular activity without the intrusive markers that traditional techniques often employ. The versatility of this method makes it a beneficial choice, allowing researchers to extend their capabilities in diagnostics and monitoring.
While this measurement technique offers many advantages, it does wrestle with certain limitations. Factors like background fluorescence or scattering can complicate readings. However, when properly calibrated and utilized, luminescence measurement remains a preferred method due to its precision and potential for miniaturization in sensors. With advancements on the horizon, such as integration with microfluidic technology, the importance of this measure will only amplify in upcoming research.
Impact on Future Research
The future of luminescence measurement promises exciting developments. Its impact on future research is significant, particularly in fields such as pharmacology and environmental science. With the increasing emphasis on non-invasive methods, researchers can harness luminescence techniques to explore biological mechanisms or environmental changes without damaging the sample or altering conditions.
A key characteristic that stands out is the adaptability of these measurement techniques. Whether applied in drug discovery to track interaction dynamics or in ecological studies to monitor ecosystem health, their application is becoming ever more versatile. This adaptability not only enhances research quality but also opens new avenues for collaboration across disciplines.
However, this also comes with challenges. As methodologies evolve and integrate with newer technologies, ensuring consistency in results becomes paramount. Thus the evolution of luminescence measurement will require ongoing refinement of techniques and thorough validation of results.
"Luminescence measurement is becoming a critical compass for guiding us through the complexities of both biological and environmental systems."
Through the evolving landscape of luminescence measurement, its potential to influence scientific understanding continues to grow. As we delve deeper into this subject, the integration of luminescent methodologies across various sectors will pave the way for innovative solutions to complex problems in the future.