Understanding mRNA Vaccine Development Process
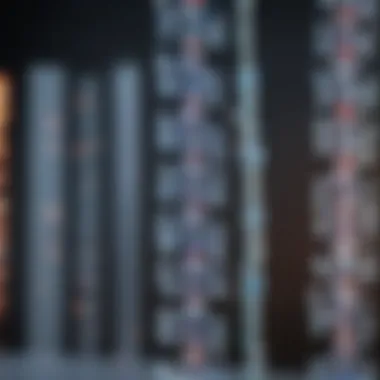
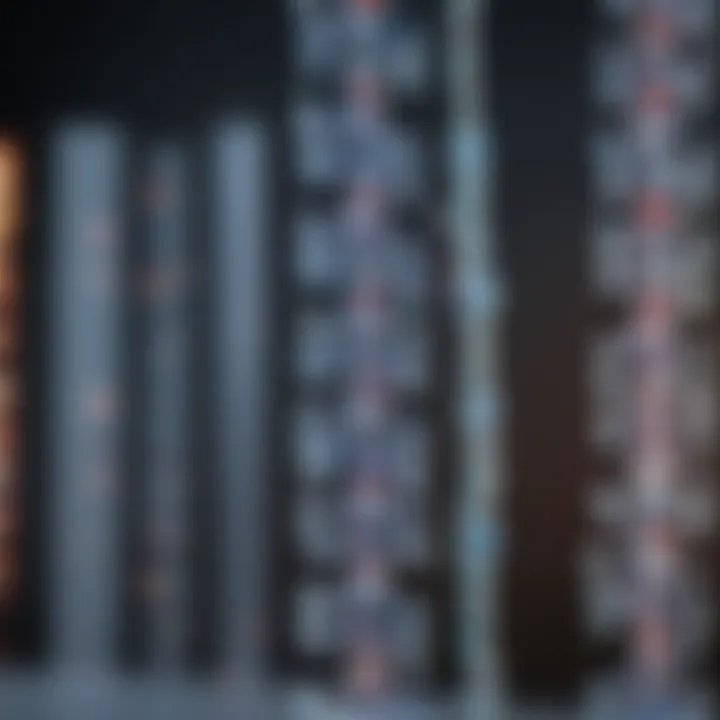
Intro
The emergence of mRNA vaccine technology has fundamentally reshaped the landscape of immunization strategies in recent years. This innovative approach not only aimed at addressing immediate public health crises but also paved the way for new horizons in vaccine development and therapy. In this section, we will delve into the key elements of mRNA vaccine development, discussing the intricate processes and methodologies involved in bringing these vaccines from concept to clinical practice.
Research Overview
Key Findings
Recent research indicates that mRNA vaccines can elicit strong immune responses. For example, studies have shown that the Pfizer-BioNTech and Moderna COVID-19 vaccines utilize mRNA encoding the SARS-CoV-2 spike protein, which led to a robust antibody response in a majority of recipients. Notably, the ability of mRNA vaccines to be developed rapidly is a game-changer, allowing scientists to respond to emerging infectious diseases with unprecedented speed.
Study Methodology
The development of mRNA vaccines typically follows a series of structured phases, utilizing both laboratory-based research and clinical trials:
- Preclinical Phase: Initial studies are conducted in vitro (test tubes) and in vivo (animal models) to assess the efficacy of the mRNA sequence.
- Clinical Trials: The trials proceed in three main stages: Phase 1: Focuses on safety profiles with a small group of volunteers. Phase 2: Expands the sample size, testing dosage and immune response further. Phase 3: Involves thousands of participants to evaluate overall efficacy and side effects compared to a placebo.
Throughout these stages, ethical standards and regulatory guidelines govern the operations to ensure participant safety and data integrity.
Background and Context
Historical Background
The concept of using mRNA for vaccines is not new, but only gained considerable traction in recent years. In the late 20th century, researchers explored the potential of mRNA as a therapeutic approach, though it faced challenges such as instability and immune recognition. The advent of lipid nanoparticles has facilitated the stabilization of mRNA, thus enabling its effective delivery into human cells.
Current Trends in the Field
Presently, mRNA technology extends beyond infectious diseases. Researchers are investigating its applications in cancer therapy where mRNA can be designed to encode tumor-specific antigens, fostering an immune response against malignant cells. Developments in personalized mRNA vaccines are igniting a new domain in treatment, where vaccines can be tailored to individual genetic profiles.
Overall, mRNA vaccine development represents a convergence of knowledge from multiple disciplines, encapsulating biology, immunology, and advanced delivery techniques.
Important Insight: "The rapid evolution of mRNA technology signifies not merely a response to health challenges but a transformational shift in how we perceive vaccine development and its possibilities."
Prologue to mRNA Vaccines
The development of mRNA vaccines marks a significant turning point in how we approach health and disease prevention. The importance of mRNA technology extends beyond its role in combating infectious diseases; it opens wide avenues for future therapeutic interventions. This section will delve into the historical context of vaccination and provide an overview of mRNA technology, two critical elements that frame the current landscape of mRNA vaccine development.
Historical Context of Vaccination
Vaccination has a storied history, tracing back over two centuries with Edward Jenner’s pioneering work on smallpox in the late 18th century. His method of inoculating individuals with material from cowpox lesions laid the groundwork for modern immunization practices. This early form of vaccination relied heavily on the principle of exposing the immune system to a less virulent pathogen to build immunity against a more dangerous one. Over the years, vaccines for diseases such as polio, measles, and influenza have been developed, utilizing various techniques, including live attenuated, inactivated, and subunit vaccines.
The imperative for innovative solutions in vaccination gained momentum with the emergence of burgeoning infectious threats. The advent of technologies, such as recombinant DNA and, subsequently, mRNA, represents a natural evolution in the quest for more effective and rapidly deployable vaccines. This historical introspection reveals how scientific advancements have been instrumental in overcoming public health challenges.
Overview of mRNA Technology
mRNA technology is a modern marvel that harnesses the power of genetic material to induce an immune response. Unlike traditional vaccines that often use inactivated or weakened forms of pathogens, mRNA vaccines utilize synthetically produced messenger RNA to instruct cells to create a harmless piece of the virus—specifically, a protein that triggers an immune response.
This method offers numerous benefits. For one, the speed of development is remarkable. Once the genetic sequence of a virus is known, mRNA vaccines can be designed and synthesized in a matter of weeks, almost on the fly. This agility has been particularly evident during the COVID-19 pandemic, where mRNA vaccines were administered to the public in record time.
Additionally, mRNA vaccines can be tailored for a broad range of infectious diseases and even have potential applications in treating cancer. This flexibility suggests a new horizon where mRNA technology may redefine how we approach both vaccination and broader therapeutic applications.
"mRNA vaccines represent a paradigm shift in how we understand immunization, creating a path for quicker responses to emergent health crises".
The rapid evolution of this technology underscores its significance as a cornerstone in modern medicine, offering hope and resilience in the face of both historical and emerging challenges in public health.
Mechanism of Action
Understanding the mechanism of action of mRNA vaccines is crucial for grasping how these innovative vaccines work. This section sheds light on the underlying principles that govern the efficacy of mRNA vaccines, something that has taken on newfound significance in recent years due to the rapid development and deployment of such vaccines. Essentially, mRNA vaccines leverage the body's own cellular machinery to create an immune response, a concept that not only alters the landscape of immunization but opens the door for a myriad of other potential applications.
How mRNA Vaccines Function
At the heart of mRNA vaccines lies the messenger RNA itself. When mRNA is injected into the body, it travels into host cells. Once inside, the cells' ribosomes take center stage. They read the sequence of nucleotides on the mRNA and translate it into a specific viral protein. For COVID-19 vaccines like the Pfizer-BioNTech and Moderna, this protein is the spike protein found on the surface of the virus. This is not just any old protein; it's a key player that enables the virus to enter human cells.
Now, here comes the clever part. As soon as these spike proteins are produced, they are displayed on the cell surface. This triggers an immune reaction. The immune system identifies the spike protein as foreign and begins to ramp up its defenses. This includes the activation of T-cells and the production of antibodies, laying the groundwork for immunity. So, when an actual virus later enters the body, the immune system has already seen it, allowing it to jump into action without delay.
"The most fascinating aspect of mRNA technology is its ability to prompt the body's own cells to produce the antigens necessary for a targeted immune response, effectively turning each injected individual into a miniature vaccine factory."
Immune Response Activation
Activation of the immune response occurs through a series of well-coordinated steps. The initial step is the recognition of the viral protein by antigen-presenting cells (APCs), such as dendritic cells. These APCs engulf the spike protein and process it, breaking it down into smaller peptide fragments.
Consequently, the processed peptides are transported to the cell surface, where they are presented to T-cells, specifically the helper T-cells. This interaction is pivotal; upon recognition, helper T-cells produce signaling molecules known as cytokines, which in turn activate B-cells capable of generating antibodies specific to the viral protein.
This multi-faceted immune response transforms the way the body reacts to pathogens. In many other vaccines, whole viruses or inactivated parts are introduced, but mRNA vaccines are a game-changer, using just the instructions needed to make the target protein. This enhances safety by removing the risk associated with live or attenuated pathogens.
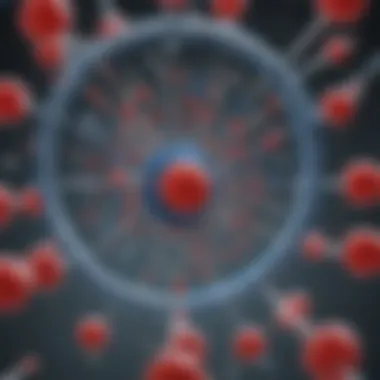
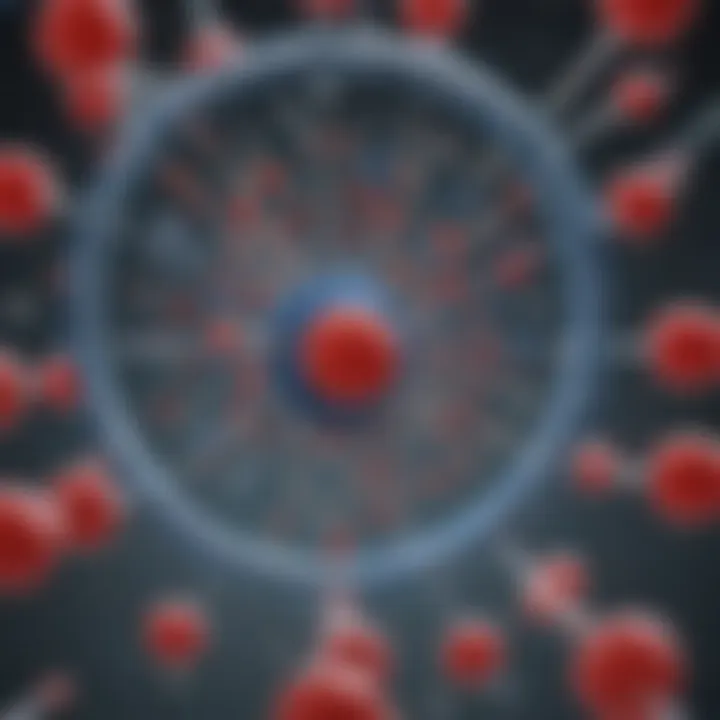
In summary, the mechanism of action for mRNA vaccines is a marvel of modern science, turning the human body into a powerful ally in the fight against infectious diseases. By understanding these intricate processes, researchers, educators, and students can better appreciate the potential future applications of mRNA technology beyond infectious diseases, perhaps even extending into areas like cancer and other therapeutic realms.
Selection of Target Antigens
Selecting the right target antigens is like picking the best apples from a tree; it might seem straightforward, but when done wrong, it can lead to unsatisfactory results, especially in vaccine development. This stage is critical in the mRNA vaccine process because the effectiveness of the vaccine ultimately hinges on the chosen viral proteins they aim to provoke an immune response against. As scientists venture into this selection, they must consider several vital elements that can determine the overall success of the vaccine produced.
Identifying Viral Proteins
Identifying specific viral proteins as targets is the first step in the process. This choice is crucial because these proteins must elicit a robust immune response without causing disease. For instance, when developing a vaccine for the SARS-CoV-2 virus, the spike protein was prioritized due to its key role in the virus's ability to infect human cells. By focusing on these biomarkers, researchers can maximize the likelihood of generating effective immunity.
When on the hunt for potential antigens, many factors come into play:
- Immunogenicity: The target protein must be capable of triggering a strong and lasting immune response.
- Conservation: Ideally, the protein should be conserved among different strains of the virus, ensuring that the vaccine remains effective against potential variants.
- Accessibility: Proteins that are easily accessible on the surface of the virus are preferable since they can be readily recognized by the immune system.
Once the target proteins are selected, further analyses and testing follow to affirm their effectiveness before proceeding to the next phases of vaccine development.
Bioinformatics Tools for Antigen Selection
In today's fast-paced scientific environment, bioinformatics tools have become invaluable assets. These tools allow researchers to sift through vast amounts of data to identify antigens efficiently. Through computational models, scientists can predict which proteins might serve as optimal targets. The years gone by have seen them honing these tools to ensure reliable results.
Some prominent bioinformatics resources and techniques include:
- Protein databases: Databases like UniProt and NCBI provide detailed information about known proteins, allowing researchers to evaluate potential targets based on their properties.
- Epitope mapping: This technique is used to identify the specific parts of the protein that the immune system recognizes, enabling targeted vaccine design.
- Predictive algorithms: Algorithms such as those used in machine learning can forecast how a given protein might behave in terms of immunogenicity, streamlining the selection process.
The integration of these bioinformatics tools into the antigen selection process not only accelerates the development timeline but also enhances the accuracy of the targeting strategy.
"The strength of the selection process will dictate the efficacy of the entire mRNA vaccine approach. It’s not just science; it's an art grounded in meticulous precision and informed decisions."
In summary, the selection of target antigens is a foundational step in mRNA vaccine development that combines the art of science and the prowess of technology. By understanding which viral proteins to target and leveraging innovative bioinformatics tools, researchers pave the way for developing safe and effective vaccines that hold the promise for a healthier future.
Synthesis of mRNA
The synthesis of mRNA is a cornerstone of vaccine development, particularly its role in creating effective mRNA vaccines. This process revolves around the need for precision and efficiency, ensuring that the generated mRNA accurately mirrors the sequence of the target antigen necessary for instigating an immune response. Without a high-quality mRNA synthesis process, the overall efficacy of the vaccine can be compromised, leading to less effective immunogenicity and potentially diminishing public confidence in vaccination efforts.
In Vitro Transcription Process
In vitro transcription is one of the key methods used to produce mRNA for vaccines. This approach entails generating RNA from a DNA template in a carefully controlled laboratory environment. The process involves several stages, beginning with the isolation of a DNA sequence that corresponds to the desired protein – often, this represents the viral antigen that the immune system needs to recognize. Then, enzymes known as RNA polymerases are employed to transcribe the DNA into mRNA. The specifics of this technique can greatly influence the yield and quality of the mRNA produced. Factors such as enzyme choice, reaction conditions, and the purity of reagents play critical roles in determining the success of the transcription process.
Additionally, the use of modified nucleotides can enhance the mRNA’s stability while also influencing its translation efficiency into proteins. Such modifications can help the mRNA to evade degradation inside the host cells, allowing for prolonged expression of the encoded antigen. Ultimately, the in vitro transcription process represents a vital intersection between biochemistry and vaccine technology, where meticulous attention to detail can have profound implications for the vaccine's overall success.
Quality Control in mRNA Production
Once mRNA synthesis is complete, ensuring the integrity and functionality of the product is paramount. Quality control measures are typically implemented throughout the entire production process, focusing on specific characteristics of the synthesized mRNA. These can include assessments of transcription efficiency, RNA purity, and the presence of any contaminants that may affect the vaccine’s effectiveness.
Common quality control techniques include:
- Spectrophotometry: This method helps in quantifying the amount of mRNA while also giving insights about its purity.
- Gel Electrophoresis: This technique separates the mRNA based on size, allowing researchers to confirm the integrity of the transcript.
- Functional Assays: These assess whether the mRNA can be effectively translated by cellular machinery into the intended protein.
"Quality control ensures that only mRNA that meets stringent criteria is considered for vaccine formulation, reinforcing the safety and efficacy standards essential in vaccine development."
Through rigorous quality control, developers can guarantee that the mRNA used in vaccines not only meets design specifications but also translates into a robust immune response, providing essential protection against diseases.
Thus, the synthesis of mRNA is a multifaceted process that weaves together elements of molecular biology, chemistry, and regulatory standards. It stands as a beacon of innovation within the realm of vaccine development, paving the way for future therapeutic endeavors.
Formulation and Delivery Methods
The formulation and delivery methods of mRNA vaccines are critical in ensuring that these innovative vaccines are effective, safe, and capable of eliciting a robust immune response. This stage involves not just the technical aspects of vaccine composition but also meticulous planning around how the vaccine will be stored, transported, and ultimately administered to patients. A well-thought-out formulation can greatly affect the stability of mRNA, its delivery efficiency, and the overall efficacy of the vaccine.
Lipid Nanoparticles in Vaccine Delivery
One of the standout features of mRNA vaccines is their use of lipid nanoparticles (LNPs) for delivering the mRNA into human cells. These nanoparticles serve as tiny vehicles, encapsulating the fragile mRNA molecules and protecting them from degradation before they reach their target cells. This is essential since mRNA is inherently unstable and could be broken down quickly in the biological environment.
Key Benefits of Lipid Nanoparticles:
- Enhanced Delivery: LNPs facilitate the mRNA’s entry into cells by fusing with cell membranes. This boosts the chances that the mRNA will reach its intended site and begin producing the desired protein, which in turn activates the immune response.
- Controlled Release: LNPs can be designed to release their mRNA payload at a specific rate, ensuring a sustained immune response. This controlled release can be advantageous in maintaining effective levels of antigen presentation over time.
- Compatibility with Various Routes of Administration: Whether injected intramuscularly or delivered via other routes, LNPs can stabilize mRNA and ensure its delivery across various biological barriers.
However, it’s important to select the right lipid formulation as not all lipids yield the same performance. This requires careful experimentation and optimisation to maximize the vaccine's effectiveness.
Stability and Storage Considerations
The stability and storage of mRNA vaccines pose significant challenges but are crucial for widespread distribution. Stability refers to the vaccine's ability to maintain its intended function over time and under varying environmental conditions.
- Cold Chain Requirements: Most mRNA vaccines require refrigeration or even deep freezing to maintain stability. For example, the Pfizer-BioNTech vaccine requires storage at -70°C, while the Moderna vaccine is stable at -20°C. This cold chain requirement can complicate logistics, especially in regions with limited healthcare infrastructure.
- Degradation Factors: Various factors can lead to mRNA degradation, including exposure to heat, light, and humidity. Therefore, packaging needs to be designed to minimize these exposures while maintaining accessibility to healthcare providers.
- Innovative Storage Solutions: Researchers are exploring alternative formulations that might allow for room temperature storage, which would greatly enhance the distribution capabilities and accessibility of mRNA vaccines globally.
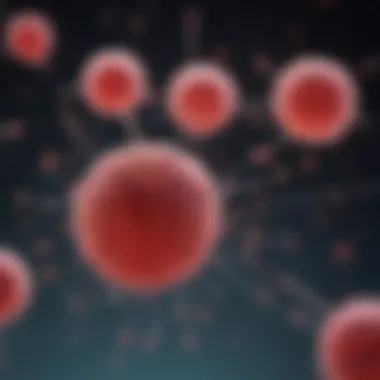
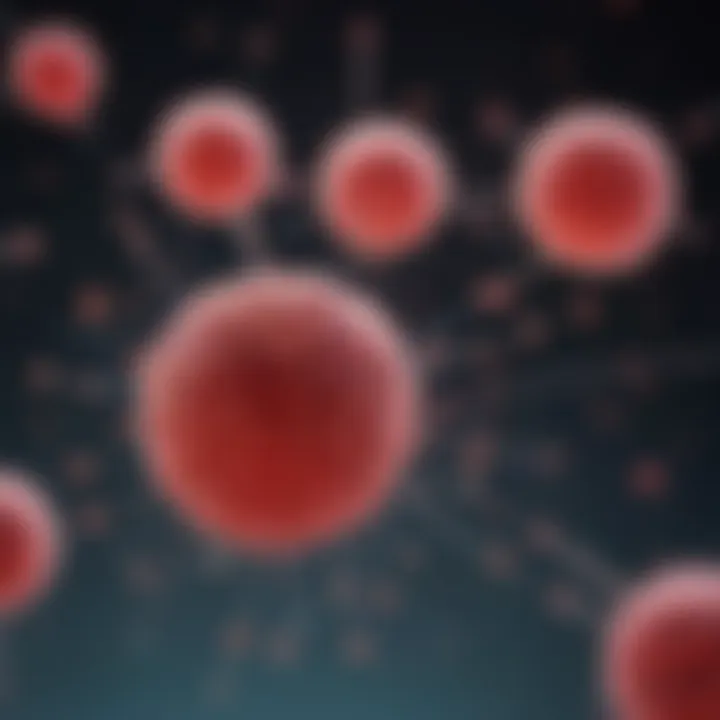
"The formulation and delivery method of mRNA vaccines are not merely technical challenges; they present a unique intersection of science and logistics that can influence vaccination success on a global scale."
Clinical Development Phases
The journey of mRNA vaccine development is not just a technical marvel; it is laden with stringent assessments and evaluations that ensure every product is both safe and effective before reaching the public. The Clinical Development Phases stand as a cornerstone in this process, separating the wheat from the chaff. These phases encompass rigorous testing scenarios that are essential for validating the vaccine's attributes—be it its safety profile, dosage efficacy, or real-world applicability.
Navigating the complexities of vaccine development is not a task for the faint-hearted. Each phase serves a distinct purpose, ensuring advancements are backed by solid data. There’s a nugget of wisdom in knowing that each hurdle passed not only signifies progress but bolsters public trust in emerging medical technologies. As we delve deeper, let’s examine the two pivotal segments under Clinical Development Phases: the Preclinical Trials and the multilayered Phases of Clinical Trials.
Preclinical Trials
Before an mRNA vaccine ever meets its first human candidates, it undergoes thorough examination through Preclinical Trials. Typically carried out in laboratory settings and using animal models, these trials aim to reveal any glaring issues with the vaccine itself.
The objectives of Preclinical Trials can be broken down into several key areas:
- Safety Testing: Identifying any immediate, adverse reactions that may arise from the vaccine.
- Efficacy Assessment: Understanding whether the vaccine generates a robust immune response.
- Dosage Determination: Fine-tuning the amount of mRNA required to produce the desired outcome without crossing the threshold of safety.
These stages are pivotal; they act as a litmus test. Often, the data gathered can lead to modifications in the formulation itself, ensuring that the subsequent human trials are more precisely aligned with the desired outcomes.
It’s like laying the groundwork for a sturdy house; without a solid foundation, the whole edifice risks toppling.
Phases of Clinical Trials
Once the Preclinical Trials show favorable results, the focus shifts to Clinical Trials, which are comprised of several sequential phases, each intricately designed to peel back the layers of understanding regarding the vaccine’s performance in humans.
- Phase 1: This initial phase involves a small cohort of healthy volunteers. The main goal is to assess safety and gauge rendering of immune responses.
- Phase 2: A larger group is tested, often composed of hundreds, to further dive into efficacy while closely monitoring for side effects.
- Phase 3: This is where the stakes get higher. Thousands of participants are involved in trials that are carefully controlled and statistically analyzed. Such a scale helps in determining how well a vaccine performs in a real-world setting, addressing questions around various demographics and long-term safety.
- Phase 4: Often called post-marketing surveillance, this phase tracks the vaccine's effectiveness and any emerging side effects once it has been widely administered.
"Without rigorous clinical trials, we are like ships adrift at sea without a compass."
The clinical phases of testing are not merely a checkbox process—they are vital in capturing promising data points that inform health authorities when making approval decisions. The resolution found in these studies is paramount, as it conveys to the public that the vaccine is grounded in scientific diligence and is safe for mass distribution.
In essence, Clinical Development Phases not only streamline the path to a viable mRNA vaccine but also ensure that what ultimately receives the green light carries both the weight of assurance and the torch of hope for many.
Regulatory Considerations
In the context of mRNA vaccine development, regulatory considerations play a vital role in ensuring public safety and trust. The rigorous vetting processes put in place by various regulatory agencies help to validate the efficacy and safety of these innovative vaccines. This section will delve into the mechanisms by which regulatory bodies operate, how they contribute to the overall vaccine development process, and what this means for future advancements in mRNA technology.
Role of Regulatory Agencies
Regulatory agencies act as gatekeepers in the vaccine development process. This is especially pertinent for mRNA vaccines, as they introduce a novel technology that has not been widely used before in broad public health immunization. Agencies such as the Food and Drug Administration (FDA) in the United States or the European Medicines Agency (EMA) in Europe ensure that any vaccine meets stringent safety standards by requiring comprehensive data from preclinical and clinical trials.
These agencies engage in multiple activities, including:
- Review of Preclinical Data: Before moving to human trials, extensive laboratory and animal studies must demonstrate initial safety and immunogenicity.
- Evaluation of Clinical Trial Protocols: Proposals for human trials undergo rigorous review to ensure they will adequately assess safety and efficacy.
- Continuous Monitoring: Regulatory bodies do not stop their oversight after approval; they maintain vigilance through Phase IV monitoring to track real-world safety and effectiveness.
These actions not only safeguard public health but also instill confidence in the vaccine development process.
Approval and Monitoring Procedures
The approval process for vaccines, particularly mRNA ones, is multi-faceted and carefully structured. This starts from the submission of the Investigational New Drug (IND) application, which lays out the safety and efficacy findings from earlier research. Following this, a meticulous review occurs.
Once the vaccine moves into Phase I, II, and III clinical trials, the data collected forms the basis for an eventual Biological License Application (BLA). In essence, this is a plea to the regulatory agency to market the vaccine, signifying that the developers believe they have proved the vaccine's safety and efficacy.
After the approval, ongoing monitoring is crucial to identify any rare side effects or long-term health impacts. Typically, this involves:
- VAERS Database: The Vaccine Adverse Event Reporting System collects and analyzes reports of adverse effects.
- Post-Marketing Surveillance Studies: These are vital for assessing longer-term safety and effectiveness once the vaccine is distributed.
It's important to underscore that regulatory considerations not only pave the way for safe vaccines but also provide a framework for accountability and transparency in public health initiatives. As mRNA technology continues to evolve, a robust regulatory environment is essential, ensuring that innovations can be harnessed while prioritizing safety.
Safety and Efficacy Assessments
In the landscape of mRNA vaccine development, safety and efficacy assessments stand as paramount cornerstones. These components not only ensure that the vaccine operates effectively in immunity enhancement but also that it does so without inflicting harm on the recipient. The intricate processes involved in these assessments determine public confidence, guiding regulatory approvals and mass vaccination strategies.
One of the critical elements in evaluating safety revolves around monitoring adverse effects that may follow vaccination. Adverse effects can range from mild reactions, such as soreness at the injection site or temporary fevers, to more serious complications, which, although rare, warrant rigorous scrutiny. The evaluation begins in preclinical trials, where animal models are used initially to ascertain any immediate adverse reactions before human trials commence.
Once human trials begin, safety monitoring continues unabated through a robust framework. Regulatory agencies often mandate ongoing reporting of adverse events throughout the clinical trial phases, ensuring any troubling signs are quickly identified. The use of databases allows manufacturers and health authorities to track these incidents, providing a vast pool of data from which to draw conclusions regarding vaccine safety. This proactive stance in addressing potential adverse effects not only helps researchers mitigate risk but also plays a crucial role in fostering public trust.
"Ensuring vaccine safety is not just about immediate reaction, but also involves understanding long-term impacts and community perceptions."
Monitoring Adverse Effects
Monitoring adverse effects is a continuous and dynamic process that progresses through various phases of vaccine development. After the initial clinical trials, participants are followed up with regular evaluations and surveys designed to capture both common and uncommon reactions.
Some key considerations include:

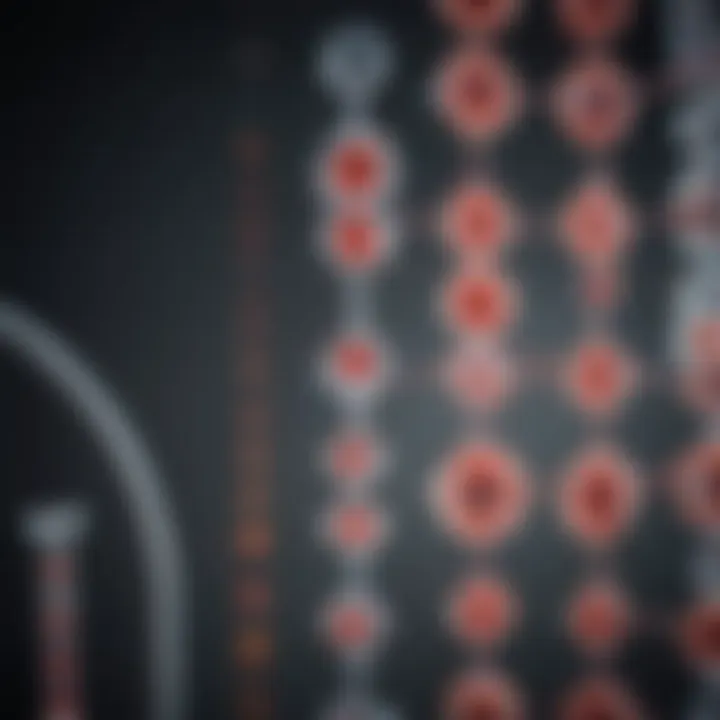
- Timeline: Tracking effects soon after vaccination and extending monitoring to weeks or months later.
- Data Collection: Utilizing digital platforms and mobile apps to facilitate easy reporting from participants.
- Risk-Benefit Analyses: Constantly weighing the benefits of immunization against potential risks.
The significance of real-time monitoring cannot be overstated. This phase enables not just the detection of safety issues but also allows public health officials to communicate findings effectively regarding the overall benefits of vaccination. The response to adverse events must be transparent, highlighting both individual and population-level safety considerations.
Long-Term Efficacy Studies
While safety is critically important, long-term efficacy studies play a fundamental role in ascertaining how well a vaccine performs over time. Initial trials often demonstrate short-term immune responses, but understanding how immunity wanes or strengthens over months—or even years—requires extensive longitudinal studies.
These studies shed light on several significant aspects:
- Duration of Immunity: Research delineates how long protective antibodies last, marking when subsequent booster shots might be required.
- Effectiveness Against Variants: It’s crucial to examine how efficacious the vaccine remains when faced with emerging viral variants.
- Population Variability: Efficacy can vary widely across different demographics, necessitating long-term data collection to ensure that community-wide applications of the vaccine remain grounded in robust evidence.
To sum up, both safety and efficacy assessments forge the backbone of mRNA vaccine development, ensuring that any rollout is not only scientifically sound but also ethically responsible. As we venture into a future where vaccines could be a primary tool for managing public health crises, a vigilant approach toward ongoing assessments will remain indispensable.
Challenges in mRNA Vaccine Development
The emergence of mRNA vaccines offers hope for rapid vaccine development, but this field is not without its pitfalls. The challenges in mRNA vaccine development affect not only the scientific community but also public health policies and vaccine adoption rates. Acknowledging these hurdles is pivotal for advancing research and ensuring effective preparations in future outbreaks.
Technological Hurdles
One of the most significant barriers in developing mRNA vaccines lies in the technological hurdles that scientists face. The mRNA technology is relatively new, and navigating various complexities can be daunting. For instance, the synthesis of high-quality mRNA is crucial. Impurities or degradation of mRNA can lead to ineffective vaccines.
Moreover, the production scale poses its own challenges. While embarking on smaller batches may seem straightforward, transitioning to large-scale production can be like trying to turn a ship in a narrow canal. Factors such as scalability, enzymatic efficiency, and purification processes must be optimized to prevent production bottlenecks.
Additionally, delivery systems present another layer of complexity. Lipid nanoparticles, the carriers for mRNA, require meticulous balancing of elements. The stability of these particles and their ability to efficiently deliver the mRNA into human cells are of utmost importance.
In light of these hurdles, continuous investment into research and development is imperative. Organizations involved in vaccine development must prioritize the innovation of technologies to streamline the manufacture and efficacy of mRNA vaccines.
Public Perception and Vaccine Hesitancy
Even when overcoming technological challenges, developers face the critical task of addressing public perception and vaccine hesitancy. In an age characterized by the rapid dissemination of information, misinformation can travel faster than truth. Many people harbor doubts regarding the safety and long-term effects of mRNA vaccines.
Engagement and transparency are crucial in fostering public trust. Educational initiatives should focus on clarifying how mRNA vaccines work, highlighting their rigorous testing processes, and debunking myths. It’s also essential to utilize platforms like reddit.com and facebook.com to enhance community outreach and discussion.
Understanding public concerns is not just beneficial—it's necessary. Listening to communities can help tailor messages that resonate with their fears and questions.
Furthermore, demographic variability plays a role. Vaccine acceptance can differ significantly across age groups, geographic locations, and cultural backgrounds. Tailored communication strategies aimed at specific subsets of the population can increase understanding and acceptance better than blanket policies.
In summary, while the potential of mRNA vaccines continues to unfold, addressing technological hurdles and public perceptions is vital. Acknowledging these challenges can facilitate a more successful vaccine rollout in the face of future health crises.
Future of mRNA Technology
The exploration of mRNA technology is shaping up to be one of the most promising advancements in the field of medicine. This section focuses on how the future of mRNA technology may offer not just a lifeboat during pandemics but also an entire fleet of therapeutic options, spurred by its adaptability and precision. As we unlock new capabilities, it's crucial to consider both the benefits and potential challenges in its applications.
Potential Beyond Infectious Diseases
mRNA vaccines were best known for their role during the COVID-19 pandemic, but their capabilities go far beyond just infectious diseases. Researchers are investigating the use of mRNA technology for various conditions, including:
- Autoimmune Diseases: There’s a growing body of literature looking at how mRNA can teach the immune system to tolerate certain antigens instead of attacking them. For instance, targeting proteins associated with autoimmune issues could result in transformative therapies.
- Metabolic Disorders: Scientists are now looking at the potential for mRNA to correct genetic errors linked to conditions like diabetes and obesity. Imagine an mRNA treatment that could potentially guide the body's metabolic pathways directly.
- Viral Infections beyond COVID-19: mRNA technology might pave the way for vaccines targeting other viruses, such as HIV or influenza, providing a robust framework for broader infection control methods.
"The adaptability of mRNA technology may revolutionize how we approach vaccine design in the coming decades."
This encapsulation of multiple therapeutic areas demonstrates how mRNA isn't merely a reactive tool against pathogens, but a proactive approach toward broader health challenges.
mRNA in Cancer Therapeutics
The application of mRNA in oncology is gaining traction as researchers explore its potential to impel tailored cancer treatments. The following points illustrate how mRNA could play a significant role in cancer therapeutics:
- Customized Cancer Vaccines: Each tumor can exhibit unique mutations. mRNA vaccines can be designed to instruct the body's immune system to specifically target those mutations, creating personalized treatment that hones in on the cancerous cells.
- Therapeutic mRNA: Instead of merely generating a vaccine response, researchers are also engineering mRNA to produce therapeutic proteins that can inhibit tumor growth. By introducing mRNA that codes for certain checkpoints or inhibitors, we can potentially neutralize cancer cells more effectively.
- Neoantigen Targeting: The future may well witness the utilization of neoantigens—new antigens that arise from mutated proteins in cancer cells—as prime targets for mRNA vaccines. This specificity could enhance the efficacy and minimize side effects by directing the immune response precisely where it’s needed.
In a nutshell, the blending of mRNA technology through personalized approaches holds great promise in shifting paradigms in cancer treatment. As this field continues to evolve, many are optimistic about its potential to redefine how we understand and treat disease at the molecular level.
Finale
The journey from the conception of mRNA vaccines to their practical application is a remarkable tale of scientific advancement. The development of mRNA vaccines signifies not just a leap in technology but a new chapter in global health. This article captures the pivotal facets of mRNA vaccine creation, which may shape future medical landscapes.
Summary of Key Points
As we summarize the critical elements discussed, several noteworthy points emerge:
- Historical Context: The roots of vaccination stretch back centuries, serving as a foundation for understanding today's innovations.
- Mechanism of Action: mRNA vaccines operate through a sophisticated mechanism that prompts the body to generate an immune response, equipping it to combat infectious agents.
- Target Antigens: The selection process is meticulous; identifying viral proteins is crucial in ensuring that the immune system can recognize and respond effectively to a potential threat.
- Synthesis and Quality Control: Producing high-quality mRNA is non-negotiable to guarantee the efficacy and safety of the vaccine.
- Regulatory Oversight: Agencies play a vital role in the approval and monitoring of vaccines, ensuring that public safety is prioritized throughout the development process.
- Future Prospects: The scope of mRNA technology transcends infectious diseases, with potential applications in cancer treatment and beyond.
The Significance of mRNA Vaccines in Modern Medicine
In the landscape of modern medicine, mRNA vaccines hold tremendous significance. They are not merely a response to the immediate health crisis posed by viruses like SARS-CoV-2 but represent a new paradigm in vaccine technology.
- Speed of Development: Unlike traditional vaccines, mRNA vaccines can be rapidly developed, allowing for swift responses to emerging pathogens. This agility could potentially shorten the timeline for dealing with future epidemics.
- Adaptability: mRNA technology can be tailored for various diseases, offering a versatile option for researchers and pharmaceutical companies alike.
- Efficacy and Safety: The robust clinical testing phases that mRNA vaccines undergo ensure they're both effective and safe. The rigorous monitoring post-approval fosters public trust.
"mRNA vaccines are the fastest, most efficient way to protect against infectious diseases, posing a groundbreaking shift in our approach to public health."
Ultimately, the journey of mRNA vaccines highlights the interplay between innovative technology and public health strategy. With broader implications for future treatments, the meticulous process of mRNA vaccine development stands as a testament to human ingenuity and resilience. Through continuous research and refinement, we stand on the brink of a new era in healthcare.