Understanding PAMs in CRISPR: Enhancing Gene Editing
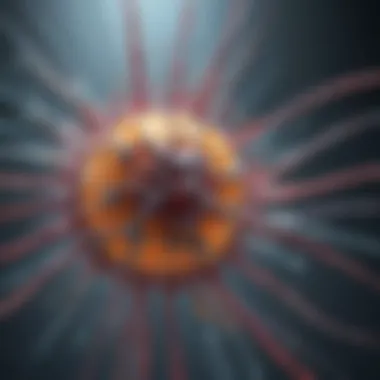
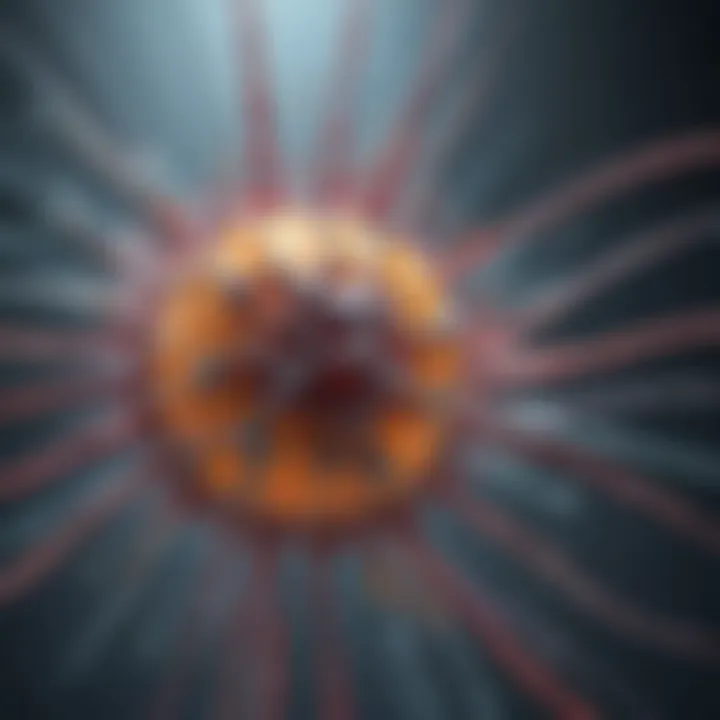
Intro
As genetic engineering surges forward, the CRISPR system continues to stand at the forefront. One crucial aspect of this technology is the notion of Protospacer Adjacent Motifs, commonly known as PAMs. These short DNA sequences play a pivotal role in determining where and how precisely CRISPR tools can operate. With the rise of gene editing's significance, understanding PAMs has never been more essential.
Let’s dive into the intricacies of PAMs, set a foundation for understanding their mechanics, and explore their relevance in driving future advancements in genetic technologies.
Research Overview
This section aims to outline the key findings and the methodologies that underlie various studies focusing on PAMs within the CRISPR landscape. It sheds light on how recent discoveries are reshaping our approach to gene editing.
Key Findings
- Specificity Enhancement: Research shows PAMs significantly enhance the specificity of CRISPR-Cas systems by acting as a prerequisite for target binding.
- Diversity in PAM Recognition: Different CRISPR systems exhibit varied PAM requirements, which affects the range of genomes that can be targeted.
- Efficiency Metrics: The presence of compatible PAM sequences influences the overall efficiency of the gene-editing process, making the choice of PAM a critical factor.
Study Methodology
The exploration of PAMs often involves a combination of experimental techniques and bioinformatics analyses. Many studies utilize:
- In vitro assays to test PAM-dependent target recognition.
- CRISPR library screens to identify effective PAM sequences across various species.
- Computational modeling to predict and understand PAM interactions within the broader context of CRISPR functionality.
Each of these methodologies plays a crucial role in painting a more detailed picture of how PAMs function within CRISPR systems.
Background and Context
To fully grasp the relevance of PAMs today, a bit of historical context can be enlightening. CRISPR technology’s roots can be traced back to bacterial immune systems, where PAMs serve as vital signals for recognizing foreign genetic material.
Historical Background
- Discovery in Nature: The initial identification of PAM sequences in bacterial CRISPR systems marked a revolutionary moment, revealing how these organisms defend themselves against phages and plasmids.
- Technological Evolution: Over the years, the application of CRISPR for gene editing in eukaryotic organisms has transformed our approach to genetics, facilitated by advancements in manipulation techniques.
Current Trends in the Field
As we stand at the present day, the research landscape shows exciting trends such as:
- Increasing interest in discovering new PAM sequences from various microbial communities, broadening the scope of CRISPR applications.
- Development of engineered CRISPR systems that can utilize atypical PAMs, leading to refined target specificity.
"The role of PAMs in CRISPR is akin to the key in a lock; without the right match, the door to genetic editing remains firmly shut."
Understanding the interplay of PAMs within CRISPR tools is not just vital for researchers and engineers working in the field, but also for educators and policy-makers engaged in the broader conversations around genetic technologies.
Intro to CRISPR Technology
CRISPR technology has transformed the landscape of genetic engineering and biotechnology, making it one of the most groundbreaking innovations in modern science. The importance of understanding CRISPR, particularly in relation to Protospacer Adjacent Motifs (PAMs), cannot be overstated. PAMs are integral to the function of CRISPR-Cas systems, serving as the keys that unlock gene-editing potential.
Overview of Gene Editing
Gene editing encompasses a range of techniques that allow scientists to modify an organism's DNA. Among these methods, CRISPR-Cas9 has emerged as a preferred choice due to its precision, efficiency, and comparatively low cost. By utilizing RNA-guided endonucleases, CRISPR systems can target specific sequences of DNA for modifications, whether that’s to knockout genes, insert sequences, or even correct mutations.
The practical implications of gene editing applications stretch across diverse fields such as medicine, agriculture, and synthetic biology. From developing disease-resistant crops to potential cures for genetic disorders, the utility and promise of gene editing create an excitement among researchers and practitioners alike.
However, with this power to alter life comes significant responsibility. Gene editing raises questions regarding ethical boundaries, unintended consequences, and the long-term ramifications on biodiversity and human health. Navigating these waters requires a strong handle on the core principles underlying CRISPR technology, particularly the mechanics of PAMs.
Historical Context of CRISPR Development
The journey of CRISPR technology traces back to the natural immune systems of bacteria, which have evolved over millions of years to defend against viral invasions. The first insights into CRISPR sequences occurred in the 1980s, but it wasn’t until the early 2000s that their function was elucidated. Researchers like Jennifer Doudna and Emmanuelle Charpentier significantly advanced the understanding of this system, ultimately leading to the formation of the CRISPR-Cas9 editing platform in 2012.
As the academic and practical applications of CRISPR flourished, the concept of PAMs became increasingly relevant. PAMs are short, specific DNA sequences that are crucial for the CRISPR machinery to recognize and bind to its target. This recognition is the first pivotal step in the editing process, determining whether the system can successfully make desired changes. The implications of PAMs on specificity and efficiency not only shape research but also guide future advancements in CRISPR technology.
In summary, as this article delves deeper into PAMs in CRISPR, clarity on the foundational aspects of gene editing will reinforce the significance of this critical motif in achieving precision in genetic manipulation.
Understanding PAMs
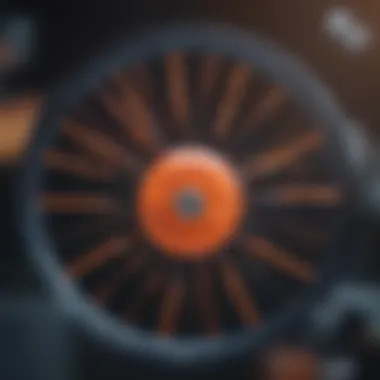
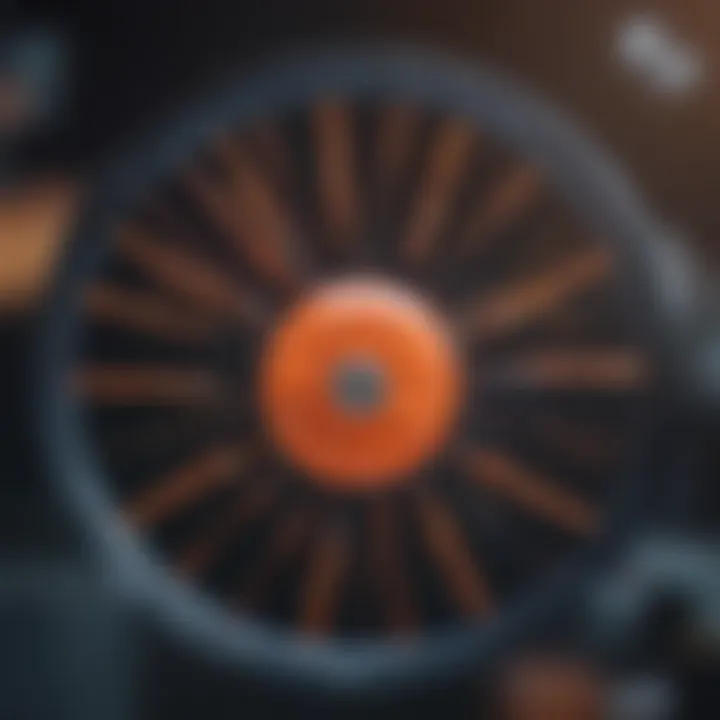
Understanding Protospacer Adjacent Motifs (PAMs) is essential in the realm of CRISPR technology as they serve as gatekeepers in the gene-editing process. These short, specific sequences of nucleotides are critical for the Cas proteins to recognize and bind to target DNA. Without PAMs, the effectiveness and precision of CRISPR interventions would be significantly hindered. Their importance can't be emphasized enough; they dictate not only where edits can happen on a DNA strand but also influence the overall success of gene editing endeavors.
Definition and Role of PAMs
PAMs are sequences adjacent to the target DNA that a Cas protein specifically recognizes. Their presence is vital for the CRISPR-Cas system to function correctly. Typically, a PAM includes a few base pairs that precede the target sequence of interest, and this structure varies across different types of Cas proteins. The most common PAM for Cas9, a widely used enzyme in CRISPR research, is "NGG", which means that after any nucleotide (N), there should be two guanines.
The role of PAMs extends beyond mere recognition. They can influence the DNA binding kinetics of the Cas protein, which means that the more favorable a PAM sequence is, the faster the Cas protein can locate its target. This efficiency is crucial in applications dealing with high-throughput gene editing requiring rapid targeting or in therapeutic contexts where timing is critical. In short, PAMs are not just a trivial detail; they fundamentally define how and where gene edits can be made.
Types of PAMs Across Species
PAMs exhibit significant variability depending on the species of the Cas proteins involved. This diversity is part and parcel of the adaptability and utility of CRISPR across a broad spectrum of applications.
- Cas9 from Streptococcus pyogenes: This is the most commonly studied CRISPR system. Its PAM sequence is generally "NGG," enabling it to recognize a wide variety of targets, thus offering ample flexibility.
- Cas9 from Neisseria meningitidis: A little different from its cousin, this system recognizes the PAM sequence "NNAGAAW". This slight nuance allows researchers to target distinct sequences in some cases where S. pyogenes Cas9 may fall short.
- Cas12 (formerly Cpf1): This enzyme offers a slightly different set of PAMs, such as "TTTV," which enables editing opportunities in more complex genomic contexts.
- Cas13: Unlike Cas9 and Cas12, Cas13 is unique since it targets RNA rather than DNA and has a different type of PAM sequence; it requires an A or U at its target site position, indicating its distinct mechanism of action.
The breadth of PAM variability across species underscores the adaptability of CRISPR technologies, allowing for targeted interventions and enhancing the overall precision of gene editing. As researchers continue to explore these sequences, they might stumble upon novel PAMs that could further revolutionize genetic engineering, making PAM research a pivotal area in CRISPR applications.
Mechanism of Action in CRISPR Systems
The mechanism of action within CRISPR systems is crucial for understanding how PAMs facilitate efficient gene editing. The recognition of PAMs by Cas proteins is the first step in the intricate dance of gene targeting. From this point forward, the sequence of events unfolds in a precise manner, leading to the desired edits in the genetic material of the target organism. This section delves into the two key phases: PAM recognition by Cas proteins and the subsequent actions that take place.
PAM Recognition by Cas Proteins
PAM, or Protospacer Adjacent Motif, serves as the gatekeeper in the CRISPR system. Without it, Cas proteins struggle to identify their intended targets. It is like trying to enter a concert without the right ticket – you just won’t get in. The Cas proteins, especially Cas9, rely heavily on the presence of these specific sequences.
In more straightforward terms, the PAM acts as a molecular beacon, signaling to the Cas proteins where to bind on the DNA strand. The presence of a PAM sequence often dictates which targets are viable for editing. Generally, PAMs are short, ranging from 2 to 5 nucleotides. If a PAM is absent, even the best-designed CRISPR construct will fail to engage with its target, wasting both time and resources.
"The specificity of gene editing hinges on PAMs; they are the unsung heroes of CRISPR technology."
Subsequent Steps Post-PAM Interaction
Once the Cas protein has successfully recognized a PAM, a series of events unfolds. The next step involves the actual binding of the CRISPR RNA (crRNA) to the complementary DNA target. This is the moment when the crRNA guides the Cas protein to its intended location, much like a GPS steering you to a hidden gem in a city.
After binding seals the deal, the Cas protein exerts its nuclease activity, cleaving the DNA at the specified location. This cutting action can lead to double-stranded breaks, initiating the cell’s repair mechanisms. The machinery within the cell takes over, attempting to mend the break. Researchers exploit these natural processes by supplying a donor DNA template, facilitating site-specific integration for corrections or functional modifications.
This entire mechanism is not without challenges. The efficiency and accuracy of these steps can vary based on the characteristics of the PAM and the overall design of the CRISPR system. Therefore, understanding these dynamics is foundational for improving gene editing techniques.
In summary, the mechanism of action in CRISPR systems intricately weaves together PAM recognition and subsequent editing steps, creating a comprehensive toolkit for genetic modification. These operations form the backbone of precision in gene editing, unlocking potential applications across healthcare, agriculture, and beyond.
Importance of PAMs in Gene Editing
The significance of Protospacer Adjacent Motifs, or PAMs, in gene editing cannot be overstated. They serve as vital gatekeepers in the CRISPR-Cas system, playing an instrumental role in defining how effectively and accurately genomic modifications can take place. These specific sequences, often comprising just a handful of nucleotides, assist the Cas proteins in recognizing their target sites, promoting specificity and efficiency during the editing process.
Impact on Target Specificity
When it comes to gene editing, hitting the right target is akin to hitting a bullseye—essential for achieving desired outcomes without collateral damage. PAMs enhance target specificity by guiding the Cas proteins to the correct genomic location. Without the presence of an appropriate PAM sequence, the Cas protein cannot bind to DNA, making it impossible for it to initiate the editing functions. This ensures that even in complex genomes, the likelihood of the Cas proteins misidentifying their targets is greatly reduced.
Insects, plants, and mammals all display variance in their PAM sequences. For instance, Streptococcus pyogenes, one of the most widely utilized bacteria in CRISPR technology, depends on a NGG PAM, while other bacterial species may have different preferences, such as NAA or NAG. This variability can be strategically exploited when designing CRISPR systems tailored for specific applications and organisms.
Influence on Editing Efficiency
The efficiency of CRISPR-mediated gene editing directly correlates to the presence and compatibility of PAMs. When PAMs align properly with a target gene, they enable the CRISPR machinery to incite double-strand breaks and subsequent repair via mechanisms like homology-directed repair or non-homologous end joining. If the PAM is unsuitable or poorly recognized by the Cas protein, editing efficiency diminishes drastically, jeopardizing experimental success.
The careful selection of PAM sequences can sometimes dictate the efficiency level of gene editing by orders of magnitude. In essence, better PAM recognition can lead to higher editing outcomes, a crucial aspect for researchers aiming for precision genetic modifications. Moreover, understanding how PAMs interact with Cas proteins can lead to innovations that bolster efficiency, ultimately paving the way for breakthroughs in both therapy and industry.
"The role of PAMs in gene editing is not just a pivotal detail but the backbone enabling specialists to navigate the complex terrain of genomic science."
In summary, the exploration of PAMs is fundamental to the advancement of gene editing techniques. Efforts toward improving target specificity and editing efficiency hinge on designing CRISPR systems that maximize the potential of PAMs, transforming how we approach genetic engineering across various fields.
Advancements in PAM Research
Research into Protospacer Adjacent Motifs (PAMs) has taken giant leaps in recent years, marking a significant shift in the understanding of CRISPR technology. By uncovering the variability and engineering potential of PAMs, scientists are not only broadening the horizons for gene editing applications but also fine-tuning the precision and efficiency of these systems. As the landscape of genetic engineering evolves, so does the demand for specific innovations that enhance therapeutic effectiveness and minimize risks.
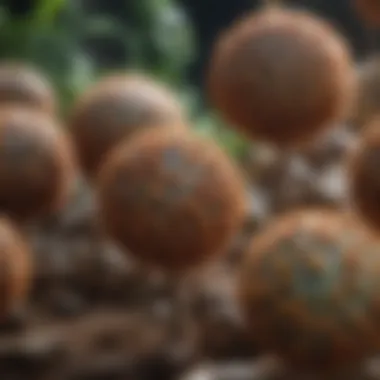
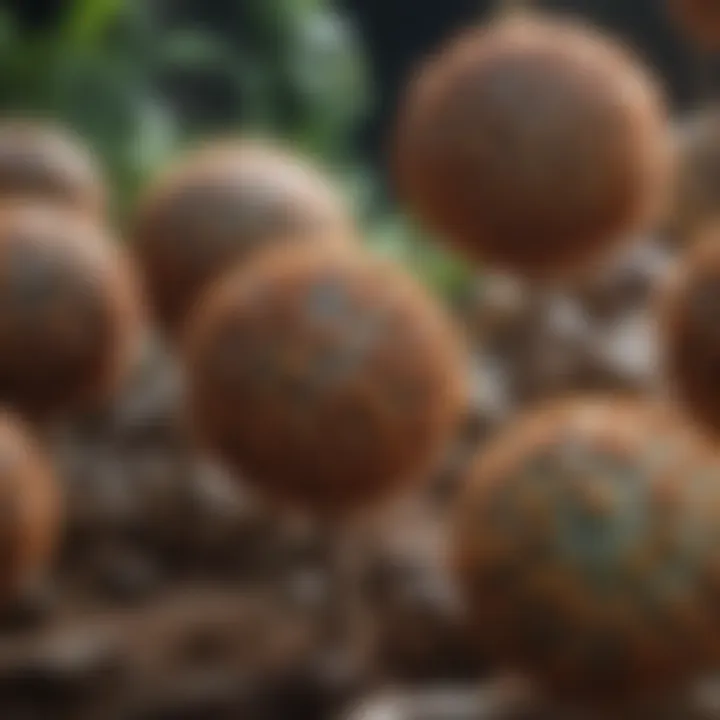
These advancements hold the promise of unlocking new avenues in medicine, agriculture, and biotechnology. As researchers dive deeper into PAM variability, the implications for genetic research become even more profound. Understanding the functions and characteristics of different PAMs allows for a more tailored approach to gene editing, which is vital for addressing disease mechanisms at the molecular level, creating more resilient crops, and developing unique biotechnological solutions.
Recent Discoveries in PAM Variability
Recent studies have showcased various types of PAMs that differ not only between species but also within specific types of CRISPR systems. For instance, researchers have identified PAM sequences specific to Streptococcus pyogenes and other Cas proteins, which adds layers of complexity to how scientists can target and edit genes precisely. Here are some key findings:
- Variability Across Microbial Species: Some organisms exhibit a unique repertoire of PAMs that can be leveraged for specific gene editing tasks. For example, a recent study revealed that certain Acinetobacter species utilize distinct PAMs, prompting interest in their potential applications in targeting specific gene sequences in related organisms.
- Unique PAMs in Archaeal Systems: Research has also shown that archaeal CRISPR systems harbor a variety of PAM sequences. This variability can lead to options for more precise targeting in environments that include extremophiles, which are often ignored in traditional gene editing approaches.
- Ecological Insights: Understanding PAMs in different microorganisms can provide insights into ecological interactions. Such understanding can help us formulate strategies for biocontrol and environmentally friendly applications, paving the way for sustainable practices in agricultural engineering.
These findings showcase the vast potential that lies within the realm of PAM variability, and how harnessing this knowledge can enhance gene editing tools.
Engineering Novel PAMs for Improved Performance
Engineered PAMs present a remarkable opportunity to enhance CRISPR technology significantly. Tailoring PAM sequences to optimize their binding affinity and specificity can transcend some of the limitations posed by naturally occurring PAMs. Here are several notable points regarding this engineering:
- Customizable PAMs: By using synthetic biology techniques, scientists can design PAMs with desired features that can potentially open doors to new gene editing targets. This customizability not only raises the accuracy of target selection but also broadens the scope of the CRISPR systems to interact with previously untargetable genes.
- Stability Improvement: Tailored PAMs may contribute to the stability of the CRISPR-Cas complex, resulting in higher editing efficiency. Variations that enhance stability could lead to increased therapeutic outcomes when employed in gene therapies for diseases like cancer or genetic disorders.
- Ngagement with Wider Applications: Another intriguing aspect is their use in stacked traits within crops. Being able to design PAMs that work harmoniously with multiple Cas proteins could lead to the development of plants with enhanced characteristics, such as drought resistance or improved nutritional content.
"Engineering novel PAMs isn't just science for the sake of knowledge; it’s a bridge to applications that could enhance lives globally."
As PAM research continues to evolve, the contributions of engineered PAMs will serve as a cornerstone for future advancements, setting a foundation for precise and practical gene-editing innovations.
Applications of PAM Research
The exploration of Protospacer Adjacent Motifs (PAMs) within CRISPR technology is more than just a niche interest for geneticists; it is a driving force behind revolutionary applications in various fields. The ability of PAMs to dictate target specificity and efficiency makes them central to advancing gene editing methodologies. Their utilization spans from revolutionary medical breakthroughs to sustainable agricultural practices. Understanding where and how these motifs can be effectively employed is pivotal not only for theoretical research but also for practical applications that can benefit society.
CRISPR in Medicine
In the realm of medicine, PAMs have emerged as game-changers. They are crucial for designing CRISPR systems tailored to recognize genetic sequences involved in diseases. For instance, researchers are utilizing PAMs to develop therapies aimed at genetic disorders, such as cystic fibrosis or sickle cell anemia. Here’s how PAMs are transforming medical practices:
- Targeted Gene Therapy: By capitalizing on the specificity that PAMs provide, scientists can target faulty genes with unprecedented precision, potentially correcting mutations at their source.
- Cancer Treatment: PAMs enable the fine-tuning of CRISPR tools like Cas9 to selectively edit genes involved in tumor growth, offering hope for personalized treatment approaches.
- Viral Infections: Certain PAMs allow researchers to edit the genomes of viruses, potentially leading to innovative therapies for viral diseases like HIV.
The application of PAMs in these contexts isn’t without challenges. Off-target effects remain a concern, as unintended modifications can lead to further complications. However, ongoing research constantly seeks to refine PAM identification and enhance the specificity of CRISPR systems. The integration of improved PAMs can bolster efficiency, aiming for minimal side effects while maximizing therapeutic outcomes.
PAMs in Agriculture and Biotechnology
The potential of PAMs extends far beyond medicine; their applications in agriculture and biotechnology promise solutions to pressing global challenges. With the ever-growing population, food security relies heavily on advancements in these fields. Specific roles PAMs play include:
- Genomic Enhancements: PAMs enable precise modifications of crops, boosting their resistance against pests and diseases. For example, the use of PAMs allows researchers to engineer rice varieties resistant to blast disease, a major threat to global rice production.
- Trait Improvement: Traits such as drought tolerance, nutritional content, and growth rate can be efficiently integrated into plants. The specificity provided by PAMs allows for the selection of desirable traits without disrupting other crucial genes.
- Synthetic Biology Efforts: PAMs facilitate vast opportunities in synthetic biology. This involves designing organisms with specialized functions, enriching biotechnological applications, such as biofuels and bioplastics.
The environmental impacts of PAM-driven technologies cannot be overstated. By enhancing crop performance and resilience, it is possible to reduce dependency on chemical fertilizers and pesticides, leading to more sustainable agricultural practices.
"Precision in gene editing is imperative for not just improving crop yields but also for ensuring our food supply remains robust against climate change."
Bioethical Considerations
In the intricate realm of gene editing, discussions around bioethical considerations take center stage. The advent of CRISPR technology, specifically the role of Protospacer Adjacent Motifs (PAMs), has opened a Pandora's box of opportunities and dilemmas. These considerations are crucial as they influence not just the scientific community, but also lawmakers, ethicists, and society at large. With gene editing, particularly with CRISPR, the stakes are sky-high. Understanding the bioethical landscape allows us to navigate this evolving field responsibly.
Ethics of Gene Editing
The ethics of gene editing is a treacherous territory. It’s not just about the science but dives into deep philosophical waters where questions of morality and long-term impact swirl. On one hand, the potential benefits are enticing. For example, altering genes to eradicate genetic diseases can lead to healthier lives for countless individuals. However, the flip side raises significant concerns.
- Informed Consent: Who decides? If gene editing modifies heritable traits, future generations may not have a say in their genetic makeup. Can we justify making such decisions on behalf of those who haven’t even been born yet?
- Equity and Access: There's a genuine risk of creating a genetic divide—where only the wealthy can benefit from advanced gene editing technologies. Should healthcare not be a universal right?
- Playing God: The notion of humans having control over life and death, or the very essence of what it means to be human, weighs heavily on ethical discussions.
It’s imperative to engage with these complexities, as the answers can shape the trajectory of CRISPR technologies for generations.
Regulatory Frameworks Surrounding CRISPR Applications
As CRISPR technology zips ahead at breakneck speed, regulatory frameworks lag as if moving through molasses. Establishing guidelines for ethical usage is crucial.
- Global Disparities: Different countries have varying regulations regarding gene editing. For instance, the US tends to be more permissive, while Europe exercises stricter controls. This inconsistency can lead to genetic tourism—where individuals travel to countries with looser regulations for treatments unavailable at home.
- Preclinical and Clinical Trials: Regulatory bodies need robust protocols that ensure safety and efficacy of CRISPR applications. Clinical trials should follow strict guidelines to protect human subjects and ensure transparency.
- Public Engagement: For regulations to be effective, public discourse is vital. Citizens must have a say in how these technologies are shaped, as their lives will be impacted. Organizations like Reddit and platforms for civic engagement can provide a stage for these discussions.
"Science must not only advance but also be accompanied by appropriate measures to ensure ethical and societal considerations are addressed."
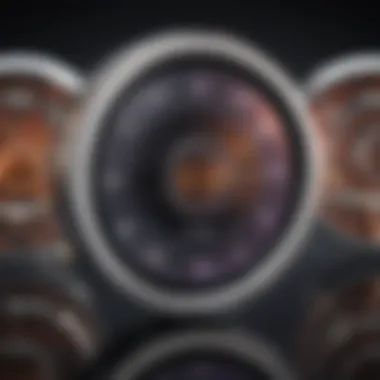
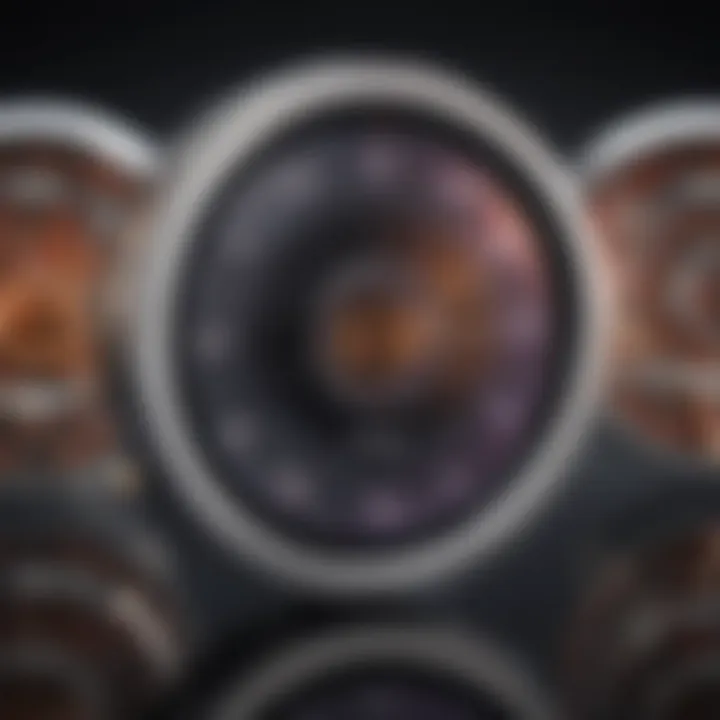
Challenges and Limitations
The burgeoning field of gene editing presents unique challenges and limitations, particularly with the role of Protospacer Adjacent Motifs (PAMs) in CRISPR technology. While PAMs are crucial for the specificity and efficiency of gene editing, their variability across different organisms and target sites introduces complexities that researchers must grapple with.
Off-Target Effects and PAM Variability
One of the primary concerns surrounding CRISPR technology is the issue of off-target effects. When a PAM sequence varies or does not match precisely with the intended target in the genome, the Cas proteins can inadvertently attach to non-target sequences. This misstep can lead to unintended mutations, which poses significant risks, especially in therapeutic applications.
Research has shown that PAM sequence specificity can influence the precision of gene editing. For instance, while the commonly used Streptococcus pyogenes Cas9 has a preference for the NGG PAM sequence, variants like Staphylococcus aureus Cas9 utilize a wider array of PAMs, such as NAG and NGA. This broadening might seem advantageous for targeting more sites, but it also increases the chance of off-target events.
"Ensuring target accuracy is as critical as the gene editing itself; otherwise, we might as well throw darts blindfolded."
Key factors to consider regarding off-target effects include:
- PAM Sequence Design: Variability can lead to mismatches, emphasizing the need for careful PAM design.
- Genome Complexity: Different tissues have various levels of accessibility, impacting how PAMs perform in vivo.
- Editing Context: The cellular environment and state can influence the binding affinity of Cas proteins.
Moving forward, engineers and researchers are investigating novel PAMs and their genomic landscape to enhance specificity while reducing off-target interactions. These include creating designer PAMs, which could theoretically optimize the targeting process itself.
Technical Hurdles in PAM Engineering
PAM engineering represents a pivotal yet intricate challenge in CRISPR research. The underlying intent is to modify PAM sequences to enhance their functionality without compromising safety. One technical hurdle is the inherent limitations in our understanding of how PAM interactions with Cas proteins dictate specificity.
Some of these challenges include:
- Predictability: Designing PAMs with predictable behavior remains tricky. Not every engineered PAM will yield the expected outcome in terms of efficiency or specificity.
- Delivery Mechanisms: The capability to deliver modified PAM sequences into cells effectively without degradation is still under exploration. This is especially relevant in therapeutic applications where precision is paramount.
- Evolutionary Constraints: Nature has fine-tuned PAM sequences over eons; manipulating these sequences may produce unintended evolutionary effects that can’t be easily predicted.
The search for improved methods in PAM engineering is ongoing. Techniques involving synthetic biology and machine learning are gaining traction. Leveraging these technologies could result in more manageable PAM variations that meet the desired criteria for efficiency and specificity.
Future Directions in CRISPR and PAM Research
The exploration of Protospacer Adjacent Motifs (PAMs) plays a pivotal role in shaping the future landscape of CRISPR technology. Understanding PAMs is not just an academic exercise; it opens up avenues for precision in modifying genes, addressing various applications across healthcare, agriculture, and environmental science. This section delves into the specific elements of potential innovations and the transformative impact of artificial intelligence in PAM discovery.
Potential Innovations in Gene Editing Technologies
As researchers navigate the evolving field of gene editing, several promising innovations are on the horizon. By focusing on the intricate role of PAMs, scientists aim to enhance the efficiency and specificity of CRISPR systems. Here are some innovative facets that warrant attention:
- Expanded PAM Diversity: Current research into PAM variability is uncovering a wealth of potential targets that could broaden the reach of CRISPR applications. New PAM sequences mean that even more specific gene editing can be accomplished, minimizing off-target effects.
- Targeted Delivery Systems: Combining PAM research with novel delivery mechanisms could lead to improved targeting of CRISPR components to specific tissues or cells. This is particularly significant in therapeutic contexts where precision is paramount to avoid unintended alterations in the genome.
- Modular CRISPR Systems: These systems employ different Cas proteins paired with distinct PAM sequences, streamlining the engineering process for various applications. This modular approach not only enhances versatility but also allows researchers to tailor gene editing tools to specific needs.
- PAM-centric Screening Techniques: Developing efficient screening methods to identify and validate PAM sequences in various biological contexts can accelerate discovery, bridging the gap between fundamental research and practical applications.
Every innovation in this realm has the potential to revolutionize gene editing technology as they usher in a new era marked by enhanced accuracy and reliability.
Integration of AI in PAM Discovery and Application
Artificial Intelligence (AI) finds itself at the heart of modern research methodologies, bringing a transformative lens to the study of PAMs in CRISPR systems. Here are key considerations regarding the integration of AI:
- Predictive Modeling: Machine learning algorithms can be employed to analyze vast datasets related to PAM sequences and their interactions with various Cas proteins. Through predictive modeling, researchers can anticipate how changes in PAMs might affect gene editing outcomes, saving time in experimental design.
- Automated Analyzes: Integrating AI facilitates the automation of routine tasks, freeing up researchers to focus on strategic aspects of their work. This not only boosts productivity but also enhances accuracy in data collection and analysis, driving further insights into PAM functionality.
- Enhanced Data Mining: AI-driven data mining tools can sift through existing genomic databases to identify novel PAMs, expediting the discovery process. This enables researchers to harness previously overlooked information that could inform future gene editing endeavors.
- Optimizing Experimental Conditions: AI can analyze outcomes from various experiments and suggest optimal conditions for PAM interactions based on historical data, which is vital for advancing experimental protocols and increasing efficiency in the lab.
In summary, the marriage of AI with gene editing technology, particularly PAM research, holds promise not just for theoretical exploration, but for real-world applications that could change the genetic landscape of many organisms.
"As we stand on this brink of innovation, exploring every avenue—including AI—truly amplifies the precision and efficacy of CRISPR technology."
Through these discussions on future directions, it is clear that both innovation and technology integration are crucial for realizing the full potential of PAMs in the realm of CRISPR gene editing. The advancements not only create new opportunities but also enhance our understanding of genetic engineering, propelling us toward a future that could be more precise than we ever imagined.
Culmination
As we wrap our exploration of PAMs within the CRISPR landscape, it’s clear that understanding these crucial motifs is fundamental to harnessing the full potential of gene editing technologies. PAMs serve as gatekeepers in the CRISPR-Cas systems, determining the specificity and efficiency of the gene editing process. Without PAMs, the precision needed to target specific genes would unravel into a chaotic mess, inevitably leading to potential off-target effects.
Summary of Key Insights
Throughout this article, we highlighted several important elements regarding PAMs and their interplay with CRISPR technology:
- PAM Definition and Role: We defined PAMs as short DNA sequences that are essential for the Cas proteins to identify and bind effectively to their target DNA. This interaction dictates the overall success of gene editing efforts.
- Diversity Across Species: The various types of PAMs found in different species showcase the adaptability and evolution of CRISPR systems, evidencing how organisms have tailored their gene-editing tools to meet their unique requirements.
- Impact on Specificity and Efficiency: We underscored how the characteristics of PAMs significantly influence both target specificity and editing efficiency, which in turn affects the reliability of gene editing outcomes.
- Recent Advances: Emerging research has brought forth new ancestral PAM variability and the engineering of novel PAMs, aiming to enhance CRISPR's capabilities.
- Biotechnological Applications: PAM research is not confined to a laboratory; it has real-world applications in medicine and agriculture that can benefit society at large.
Final Thoughts on the Future of PAMs in CRISPR
Looking forward, the future of PAMs in CRISPR does not just hinge on refining existing techniques, but also on innovative approaches that could reshape gene editing. With the integration of AI in the discovery and application of PAMs, we could witness unprecedented accuracy in genetic engineering. This could lead to therapies that tackle genetic disorders head-on, and agricultural advancements that bolster food security.
The successful manipulation of PAMs may well determine the trajectory of future CRISPR technologies, representing a beacon of hope in both the medical and agricultural fields.
Moreover, as research progresses, it’s crucial to maintain a vigilant stance regarding the ethical implications surrounding gene editing. As scientists push the boundaries of what’s possible, responsible stewardship of such powerful tools is essential to ensure safe and equitable use.