Understanding Reverse Transcription PCR Tests
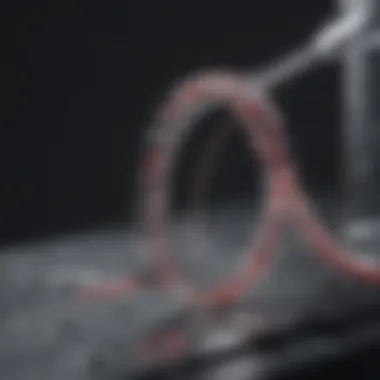
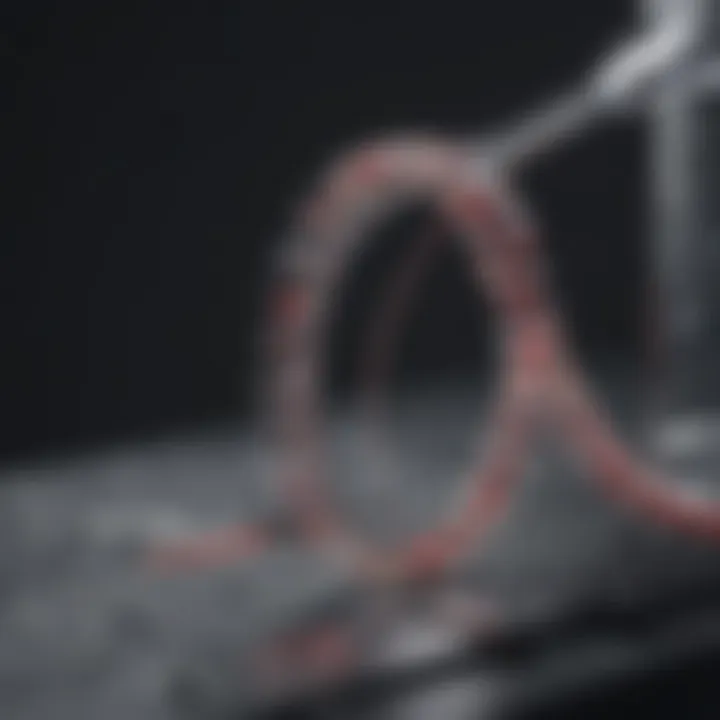
Intro
Reverse Transcription PCR (RT-PCR) stands as a cornerstone in molecular biology. This technique, which elegantly marries reverse transcription and polymerase chain reaction, is essential for converting RNA into complementary DNA (cDNA). Its significance, particularly in the realm of viral detection, cannot be overstated. In an era marked by rapid advancements in biotechnology, understanding RT-PCR is critical for students, researchers, and professionals alike.
Research Overview
In recent times, RT-PCR has emerged as a vital tool in genomics and virology. Its ability to amplify specific segments of RNA makes it invaluable for diagnostic purposes, especially in identifying viral infections like COVID-19. The process begins with extracting RNA from a sample, which is then reverse-transcribed into cDNA; subsequently, PCR amplifies this DNA, enabling the detection of even the minutest viral particles.
Key Findings
- Prominent Diagnostic Tool: RT-PCR has been used as a primary method for the diagnosis of infectious diseases. It offers sensitivity and specificity that other methods may lack.
- Efficiency in Research: Many researchers find RT-PCR indispensable for quantitative analysis of gene expression, providing insights into cellular responses and disease mechanisms.
- Adaptability: This technique is versatile, finding applications in diverse fields ranging from healthcare diagnostics to environmental monitoring.
Study Methodology
A typical RT-PCR process can be broken down into several meticulous steps:
- RNA Extraction: Use of various kits tailored for different sample types to isolate RNA.
- Reverse Transcription: This step involves utilizing reverse transcriptase to convert RNA into cDNA.
- Amplification via PCR: The cDNA is then amplified, typically using specific primers that correspond to the target sequences.
- Analysis: The final step involves analyzing the amplified products, often through gel electrophoresis or quantitative methods.
Background and Context
Historical Background
The origins of RT-PCR can be traced back to the discovery of reverse transcriptase in the early 1970s, a moment that revolutionized molecular biology. Initially developed for research on retroviruses, the adaptability of RT-PCR soon led to its widespread use across various disciplines. Over the decades, improvements in techniques and technologies have enhanced its precision and efficiency.
Current Trends in the Field
Today, RT-PCR is not just confined to labs; it has practical implications in medical diagnostics, agricultural advancements, and even forensic science. Continuous improvements are being made in terms of speed, sensitivity, and robustness. As researchers are pushing the boundaries of science, the integration of real-time RT-PCR has added a layer of sophistication, enabling real-time monitoring of gene expression, thereby enhancing data accuracy and reliability.
"The utility of RT-PCR cannot be diminished; it has become the gold standard in the identification and quantification of RNA, influencing research direction and clinical practices."
The benefits of understanding RT-PCR extend beyond mere laboratory techniques. As the world increasingly leans on genetic testing for diagnosis and treatment strategies, familiarizing oneself with this method can pave the way for innovative solutions in healthcare and research.
Intro to Reverse Transcription PCR
Understanding reverse transcription PCR (RT-PCR) is pivotal in the realm of molecular biology. This technique holds significant importance in various fields such as diagnostics, research, and therapeutic development. RT-PCR enables scientists and medical professionals to analyze RNA effectively, providing a vital insight into gene expression and the presence of viral pathogens. Given the increasing complexities of genetic diseases and viral outbreaks, the relevance of RT-PCR cannot be overstated.
In this section, we will explore two key aspects of RT-PCR: its definition and historical background. These elements set the foundation for appreciating the comprehensive details that follow in this article, addressing the mechanisms involved, applications, and the ramifications of RT-PCR in modern science.
Definition and Overview
Reverse transcription PCR is a laboratory technique that allows for the conversion of RNA into complementary DNA (cDNA). This transformation is essential because, when studying genetic materials, DNA is more stable and easier to work with compared to RNA. Therefore, identifying RNA sequencesâand by extension, understanding the underlying pathways of diseases or gene functionsâbegins with RT-PCR.
This process typically consists of two main phases:
- Reverse transcription: This is where RNA is converted into cDNA using the enzyme reverse transcriptase.
- Polymerase chain reaction (PCR): After obtaining cDNA, a PCR amplification process is implemented to generate millions of copies of the cDNA, thereby facilitating detailed analysis.
The importance of RT-PCR in diagnostics is particularly pronounced, especially in detecting viral infections. By enabling the identification of specific viral RNA sequences, RT-PCR has become a critical tool in infectious disease research and diagnostics. Furthermore, this method helps unravel gene expression patterns, offering insights into normal and pathological states of cells.
Historical Background
The journey of reverse transcription PCR began in the 1970s when researchers first identified reverse transcriptaseâthe enzyme responsible for synthesizing cDNA from RNA. This discovery was crucial for expanding our understanding of retroviruses like HIV, leading to groundbreaking research in virology and molecular biology. The initial attempts to incorporate reverse transcription into PCR techniques arose in the late 1980s. The integration of these methodologies provided an advanced tool for examining RNA-based processes.
By the 1990s, RT-PCR had solidified its place in molecular biology labs globally. Techniques like quantitative RT-PCR were developed to not only detect but also quantify mRNA levels, enabling researchers to explore gene expression regulation in greater detail.
In summary, the historical evolution of RT-PCR demonstrates a natural progression toward more sophisticated methods in biological research.
The landmark advancements in this technique have revolutionized fields like clinical diagnostics, genomics, and biotechnology. RT-PCR is now an essential part of the toolkit for many molecular biologists and medical professionals, underpinning our understanding of genetic expressions and pathogen detection.
"Real-time RT-PCR methods have advanced our ability to analyze viral pathogens efficiently, enhancing response strategies during outbreaks."
As we dive deeper into the principles of RT-PCR, it becomes clear that understanding these foundational elements is key to grasping its multifaceted applications and implications.
Principles of RT-PCR
When it comes to the realm of molecular biology, understanding the principles of reverse transcription PCR (RT-PCR) is crucial. This advanced technique, revered for its role in genetic research and diagnostics, hinges on a few key processes that allow scientists to transform RNA into a usable form of genetic template. Thus, getting to grips with the intricate workings of RT-PCR not only enhances comprehension of the technique, but also reveals its broader applications in medical science and biotechnology.
RNA to cDNA Conversion
To grasp the significance of RT-PCR, one must first understand what happens when RNA is converted into complementary DNA (cDNA). This conversion serves as the backbone of the technique. During this process, an enzyme called reverse transcriptase plays a central role. Essentially, this enzyme reads the RNA sequence and synthesizes a complementary strand of DNA. The reaction conditions, particularly the temperature, must be just right to encourage the enzyme's activity while avoiding degradation of the RNA.
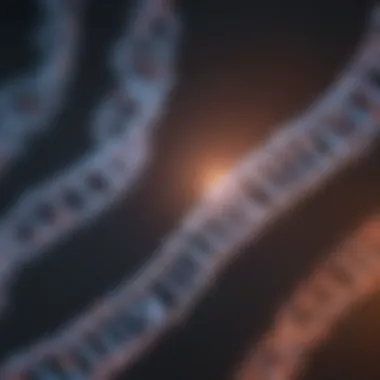
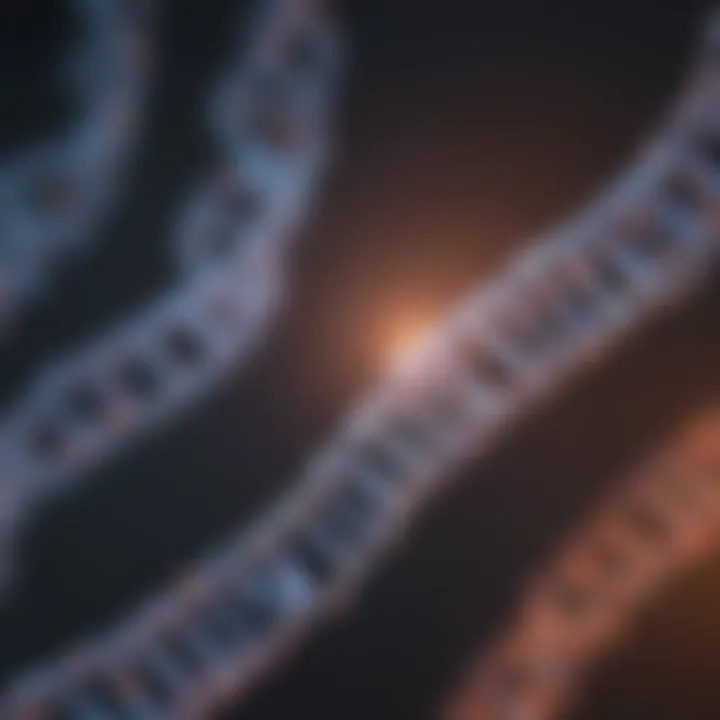
Hereâs a quick breakdown of this conversion process:
- RNA preparation: Begin with high-quality RNA, as impurities can lead to less than optimal results.
- Enzyme addition: Reverse transcriptase, together with primers and dNTPs (deoxynucleotide triphosphates), is added to the reaction mix.
- Incubation: The mix is then incubated at 37-50 degrees Celsius, conducive for the enzyme to work.
After this step, researchers are left with cDNA, which is stable and more amenable for subsequent amplification. Itâs worth noting that the accuracy of this step is vital, since errors can propagate in later stages, leading to incorrect results in analyses.
Amplification of DNA
Once cDNA is produced, the stage is set for amplificationâa critical aspect of RT-PCR. This phase enables scientists to generate millions of copies of the cDNA, increasing its concentration to detectable levels. The magic happens in cycles, typically involving denaturation, annealing, and extension.
Here's how it generally runs:
- Denaturation: The double-stranded cDNA is heated to around 95 degrees Celsius to break the hydrogen bonds, separating the strands.
- Annealing: After the separation, the temperature drops to allow primers to bind to the specific sequences on the cDNA strands. Primers are crucial, as they lay the groundwork for the next phase.
- Extension: The temperature is then raised again, allowing a DNA polymerase enzyme to extend the primers, creating new strands of DNA.
This cycle is repeated several times, generally 25 to 40 cycles, and each cycle amplifies the amount of target DNA exponentially. By the end of this process, the quantity of amplificient can rise to the level where it becomes easier to analyze with techniques like gel electrophoresis.
Ultimately, the principles of RT-PCR stand not only as the cornerstone of this methodology but also underscore its powerful versatility in applications like detecting viral pathogens, assessing gene expression, and much more.
Components of RT-PCR
Understanding the components of reverse transcription PCR is essential for grasping how this technique operates. Each part plays a critical role, ensuring that the process runs smoothly and effectively. Letâs take a closer look at the key elements involved in RT-PCR, emphasizing their individual and collective significance.
Reagents and Enzymes
At the heart of RT-PCR lies a carefully curated mix of reagents and enzymes, each chosen for its specific function. Here are the primary components:
- Reverse Transcriptase: This enzyme is crucial because it converts RNA into complementary DNA (cDNA) during the first step of RT-PCR. The efficiency and fidelity of conversion rely heavily on the type of reverse transcriptase used. Some variants, like M-MLV or AMV, offer better performance depending on the conditions of the experiment.
- RNA Sample: The starting material, often extracted from biological samples, can be mRNA or total RNA. The quality of the RNA is paramount. Contaminated or degraded RNA can lead to inaccurate results, thereby undermining the entire experiment.
- Primers: Short sequences of nucleotides specifically designed to bind to the cDNA target region facilitate the amplification process. The design of these primers is intricate because mismatches can drastically reduce the efficiency of the amplification.
- Nucleotides (dNTPs): These are the building blocks of DNA, providing the essential components for synthesizing new DNA strands. High-quality dNTPs are necessary for an effective RT-PCR, as impurities can impede the enzymatic reactions.
- Buffer Solutions: The enzyme activities are influenced by pH and ionic strength, making buffer solutions vital. They maintain optimal conditions for reverse transcriptase and DNA polymerase function, thereby enhancing the reliability of the results.
The interplay of these components ensures that the RT-PCR process is not merely an experiment, but a finely tuned operation designed to yield consistent results. Well-prepared reagents and enzymes lead to effective and reproducible RT-PCR outputs.
Thermal Cycler's Role
One cannot overlook the thermal cycler in the RT-PCR setup. This device is more than just a temperature control; it orchestrates the entire PCR process. The role it plays can be broken down as follows:
- Temperature Cycling: The thermal cycler provides precise temperature settings necessary for the various stages of RT-PCR. Typically, it involves:
- Time Management: Each step in RT-PCR is time-sensitive. The thermal cycler must maintain the right temperatures for the correct duration, as even small deviations can affect the fidelity of the amplification.
- Real-Time Monitoring: Advanced thermal cyclers now include real-time monitoring capabilities, allowing for the detection of amplified DNA as the process unfolds. This development gives researchers the ability to quantify the starting RNA and track dynamics during the reaction.
- Denaturation: Heating the mixture to separate the strands of DNA (or cDNA).
- Annealing: Cooling down to allow primers to bind to the target sequences.
- Extension: Raising the temperature again for the DNA polymerase to synthesize new DNA strands.
Therefore, the thermal cycler doesn't merely conduct the reactions; it is instrumental in ensuring that the RT-PCR yields precise and reliable results. Without these critical components operating in unison, researchers and professionals would struggle to achieve their objectives in RNA analysis.
RT-PCR Methodology
Understanding the methodology behind Reverse Transcription PCR (RT-PCR) is crucial for grasping how this powerful technique can be applied in various fields, particularly in molecular biology and clinical diagnostics. The methodology involves a series of steps and considerations that ensure both the reliability of results and the efficiency of the procedure. Through this section, we will unpack the detailed step-by-step process along with strategies for optimization, highlighting key benefits and considerations that users should keep in mind.
Step-by-Step Process
The RT-PCR process can appear intricate at first glance, yet it is structured in a systematic manner. This clarity facilitates its application in both research and clinical settings. Hereâs a typical flow of how the RT-PCR is conducted:
- Sample Collection: The first step often starts with gathering appropriate samples. This could be anything from blood, tissues, or other biological materials containing RNA.
- RNA Extraction: Post collection, total RNA is extracted from the samples. Various kits are available that utilize methods such as phenol-chloroform extraction or column-based techniques to isolate RNA effectively.
- Reverse Transcription: The extracted RNA is then converted into complementary DNA (cDNA). This step involves the use of reverse transcriptase enzymes along with primers that can be either random hexamers, oligo(dT) primers, or gene-specific primers to initiate the process.
- PCR Amplification: The cDNA serves as a template for the PCR amplification phase. Here, primers specific to the target genes are added along with the necessary reagents like dNTPs and Taq polymerase. This mixture undergoes cycles of denaturation, annealing, and extension in a thermal cycler, amplifying the cDNA for analysis.
- Analysis of Results: Following amplification, the results can be analyzed using various methods such as gel electrophoresis or real-time PCR detection systems to quantify and visualize the DNA fragments.
This systematic approach ensures that researchers and clinicians can derive meaningful insights from their results, aligning perfectly with the requirements in diagnostics or experimental settings.
Optimization Techniques
While the step-by-step approach lays down the groundwork, optimization plays a critical role in enhancing the reliability and precision of RT-PCR outcomes. A subpar optimization can yield unreliable results, disrupting downstream analyses. Here are several key optimization techniques:
- Primer Design: Developing efficient primers is paramount. Primers should be specific to the target sequence, with an appropriate melting temperature and minimal secondary structures. Tools like Primer3 or NCBI Primer-BLAST can assist in this.
- Enzyme Selection: The choice of reverse transcriptase enzyme can impact the quality of cDNA synthesis. Select an enzyme based on factors like stability, fidelity, and inhibition sensitivity to reagents in the assay.
- Reaction Conditions: Adjusting reaction conditions, including salt concentration and pH, can help achieve optimal cDNA synthesis and amplification. It's recommended to perform a gradient PCR to find the best annealing temperature for primers.
- Inhibitor Removal: Since many biological samples contain substances that can inhibit PCR reactions, optimizing the extraction and purification process can mitigate this. It may require selecting specific kits dedicated to the type of sample being analyzed.
- Designing Replicates: Running samples in duplicates or triplicates can provide more reliable data and allows for statistical analysis of the results to ensure the integrity of the findings.
Incorporating optimization techniques helps researchers achieve greater accuracy in quantification, paving the way for better interpretation of biological significance in gene expression studies, viral load assessments, and more.
"Mastering RT-PCR techniques opens a realm of possibilities in diagnostics and research, promising new insights into complex biological processes."
By following these procedures and optimization methods, those engaged in molecular biology can improve both the consistency and sensitivity of RT-PCR results, laying a strong foundation for subsequent applications in understanding viral infections and genetic variants.
Applications of RT-PCR
Reverse transcription PCR (RT-PCR) plays a pivotal role in modern molecular biology. Its advantages extend far beyond basic research, finding significant applications in diagnosing viral infections, analyzing gene expression, and detecting genetic variants. Each of these areas not only showcases the versatility of RT-PCR but also emphasizes its importance in both clinical and research settings.
Viral Infection Diagnosis
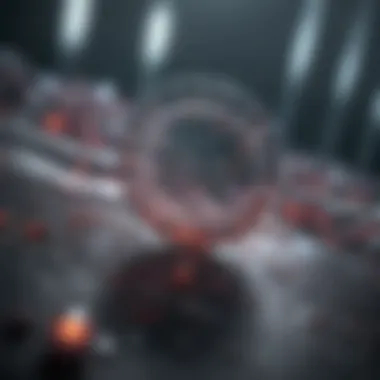
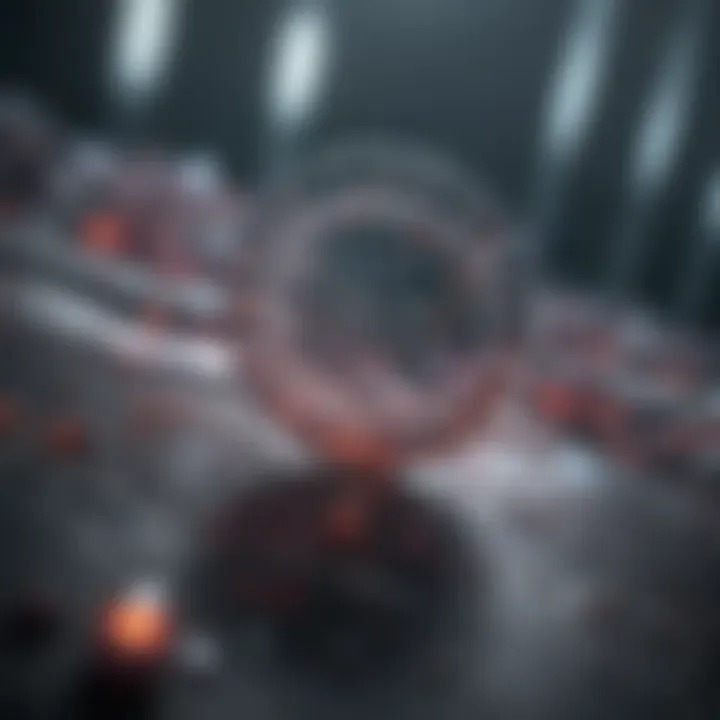
Viral diseases have long posed challenges to the healthcare system. RT-PCR emerges as a reliable and rapid tool for the detection of viral infections. By transcribing and amplifying the viral RNA into DNA, it enables the identification of even low levels of viral genetic material. This sensitivity is crucial during early infection stages when symptoms may not yet manifest.
In the context of epidemics, consider the COVID-19 pandemic; RT-PCR was instrumental in diagnosing cases swiftly. The test identified viral RNA from patient samples, facilitating timely isolation and treatment, thus helping curtail the spread of the virus. The ability of RT-PCR to provide accurate results in a short time frame makes it immensely valuable in managing public health.
"The sensitivity and specificity of RT-PCR are unmatched, making it a gold standard for viral diagnosis."
Gene Expression Analysis
Understanding gene expression is foundational in molecular biology. RT-PCR allows researchers to quantify the expression levels of specific genes under various conditions. This application is pivotal in areas such as developmental biology and cancer research, where gene expression patterns can indicate how cells respond to stimuli or evolve.
For instance, studying how cancer cells respond to treatment involves comparing their gene expression profiles before and after therapy. RT-PCR offers a meticulous technique to measure the levels of mRNA, providing insights into which genes are over or under-expressed. Such data can inform treatment decisions and help in the development of targeted therapies.
Detection of Genetic Variants
The identification of genetic variants is another critical application of RT-PCR. Genetic variants can have profound implications for disease susceptibility, treatment responses, and overall health. By using RT-PCR to analyze RNA samples, researchers can pinpoint mutations that might not be easily identified through other methods.
For example, RT-PCR can be employed to examine polymorphisms in genes associated with hereditary diseases. Clinicians can use this information not only for diagnosing conditions but also for guiding personalized treatment plans. The ability to detect and analyze these variants illustrates how RT-PCR serves as a bridge between fundamental research and clinical applications.
In summary, the applications of RT-PCR are vast and essential in both diagnostic and research contexts. Its capabilities in viral infection diagnosis, gene expression analysis, and the detection of genetic variants showcase how it shapes the landscape of molecular biology. As both technology and methodologies advance, the relevance of RT-PCR is likely to expand, opening new avenues in genomics and personalized medicine.
Advantages of RT-PCR
In the realm of molecular biology, the advantages of Reverse Transcription PCR (RT-PCR) stand out prominently. This technique is not just a passing trend; it has redefined the way scientists approach the study of genes and the diagnosis of diseases. RT-PCR enables researchers to engage with RNA transcripts directly, revealing insights that were previously obscured. These benefits extend to various fields, encompassing clinical diagnostics, infectious disease monitoring, and even genetic research.
Sensitivity and Specificity
One of the most compelling features of RT-PCR is its remarkable sensitivity and specificity. Sensitivity refers to the methodâs ability to detect even the tiniest amounts of target RNA. For instance, in the case of a viral infection, detecting low levels of viral RNA is crucial. Imagine a scenario where a patient shows mild symptoms, yet standard tests miss the virus due to low concentrations. RT-PCR shines here; its high sensitivity ensures that even minute quantities are amplified, making detection feasible.
Specificity, on the other hand, denotes how accurately the method identifies the target RNA without confusion from non-target sequences. In practical terms, this means RT-PCR can discern a specific viral RNA from the myriad of other RNA types present in a sample. This dual capability ensures not just detection, but precise identification, which is essential for forming appropriate treatment plans.
"The power of RT-PCR lies in its ability to pinpoint and amplify, catering to the needs of both diagnostics and research."
Speed of Results
Aside from sensitivity and specificity, the speed of results is another notable advantage. Traditional laboratory diagnostics can be time-consuming, requiring days or even weeks. In contrast, RT-PCR can deliver reliable results within hours. This rapid turnaround can mean the difference between effective treatment and missed opportunities, particularly in clinical settings dealing with emerging viruses.
For example, during an outbreak of a contagious disease, timely identification of the pathogen can facilitate quicker quarantine measures, thereby controlling spread. The speed offered by RT-PCR is not merely a convenience; it plays a critical role in public health responses, guiding decisions that impact population safety.
In summary, the advantages of RT-PCRâits sensitivity, specificity, and rapid resultsâpropel it to the forefront of contemporary molecular diagnostics. As this technology continues to advance, it holds the potential to revolutionize how we understand and respond to both genetic and infectious challenges.
Limitations of RT-PCR
While reverse transcription PCR (RT-PCR) is a powerful tool, it is not without its shortcomings. Understanding these limitations is crucial for students, researchers, and professionals who rely on this technique in their work. Recognizing the drawbacks not only helps in mitigating risks but also promotes the optimization of experimental designs, leading to more reliable results.
Potential for Contamination
One significant issue with RT-PCR is the potential for contamination. When a small amount of target RNA is amplified, even minute traces of foreign genetic material can lead to erroneous results. This contamination often goes unnoticed and can severely impact experimental outcomes.
Laboratories must adhere to strict protocols to limit contamination risks. Here are a few strategies:
- Dedicated Equipment: Using specific tools for RNA work, avoiding overlaps with DNA procedures.
- Clean Workspaces: Regular decontamination of surfaces to eliminate any residual nucleic acids.
- Post-PCR Practices: Implementing areas with controlled access where amplified products are handled reduces unwanted interactions.
A common practice is the use of uracil-DNA glycosylase (UDG) to degrade any carryover products from previous reactions. This enzyme can help mitigate false positives originating from amplified remnants.
"In the world of molecular biology, contamination could be the sneaky thief of accuracy."
Quantification Challenges
Another notable limitation of RT-PCR lies in quantification challenges. Although RT-PCR can provide qualitative insights, measuring exact RNA quantities becomes tricky. Unlike qPCR, which is designed for quantitative assessments, RT-PCR generally yields semi-quantitative results.
Several factors contribute to these quantification difficulties:
- RNA Quality: The integrity of the RNA samples significantly affects the results. Degraded RNA compromises reliability.
- Variability in Efficiencies: The efficiency of cDNA synthesis can vary depending on the primers and conditions used, leading to inconsistent results.
- Data Interpretation: Without standardization or relevant controls, interpreting the results can sometimes feel like trying to read the tea leaves.
Scientists often employ a calibration curve based on known concentrations of RNA to help in quantification. However, generating this curve adds additional complexity to the experiment and requires careful execution and validation.
In summary, understanding the limitations of RT-PCRâincluding contamination risks and quantification challengesâprovides essential insights. Addressing these concerns allows for better experimental design and interpretation, ultimately enhancing the efficacy of RT-PCR in both research and clinical settings.
RT-PCR vs. qPCR

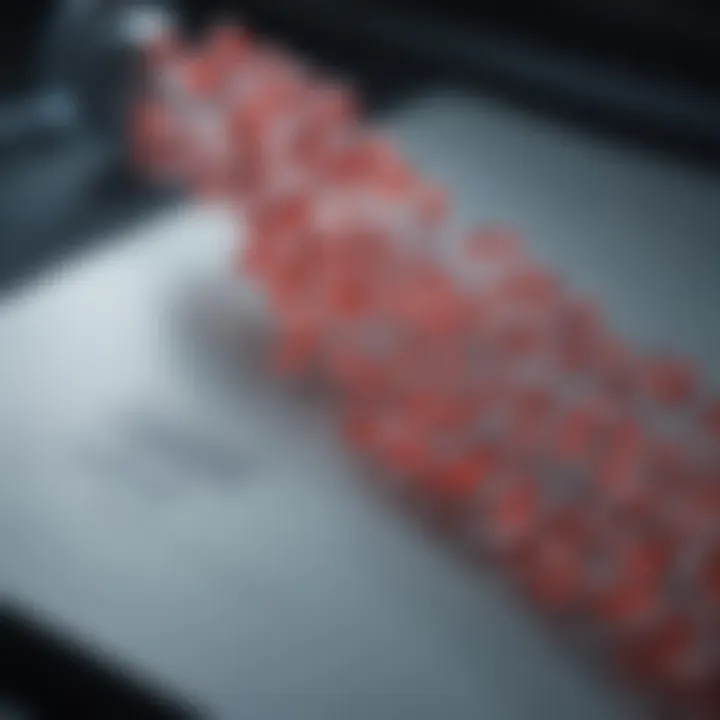
The comparison between RT-PCR and qPCR is not just a technical discussion; it highlights two cornerstone techniques in the field of molecular biology. Both techniques are critical for nucleic acid analysis, yet they approach the task from different angles. Understanding how they stack up against each other is key for students, researchers, and professionals looking to optimize their studies, particularly in detecting gene expression or diagnosing infections.
Comparative Analysis
At their core, RT-PCR (Reverse Transcription PCR) and qPCR (Quantitative PCR) serve distinct roles in the laboratory. RT-PCR is primarily used for qualitative detection of RNA by converting it to complementary DNA (cDNA). This conversion step anchors RT-PCRâs applications, especially in scenarios where RNA is the target, such as identifying RNA viruses like HIV or SARS-CoV-2.
On the other hand, qPCR is focused on quantification. It measures the amount of DNA, whether that DNA is genomic or cDNA from a previous RT-PCR. qPCR utilizes fluorescent dyes that emit light, enabling real-time analysis of the PCR amplification process. This can be beneficial in diagnosing conditions based on the threshold cycle (Ct) value, which reflects the amount of target DNA present.
"While RT-PCR allows for the qualitative assessment of RNA, qPCR delivers quantitative insights that can illuminate significant variations in gene expression."
From a technical perspective, RT-PCR can be seen as a precursor to qPCR when studying RNA samples due to its foundational role in converting RNA to cDNA. Thus, in practice, researchers may employ RT-PCR to create the cDNA first and subsequently use qPCR for accurate quantification. Itâs not uncommon for laboratories to integrate both techniques, thus enhancing the breadth of information garnered from a single sample.
Key factors to weigh when considering the differences between RT-PCR and qPCR include:
- Purpose: RT-PCR focuses on detecting and amplifying RNA, whereas qPCR quantifies DNA.
- Output: RT-PCR provides qualitative results, while qPCR offers quantitative data.
- Sensitivity: Both methods are sensitive, but context matters. RT-PCR may have a higher sensitivity for RNA targets due to the absence of DNA conversion bias.
Situational Utilization
The applications for RT-PCR and qPCR vary considerably based on the needs of the user. In clinical diagnostics, RT-PCR is often employed when a viral infection is suspected, particularly where RNA viruses are involved. For instance, during a viral outbreak, RT-PCR can quickly confirm the presence of the pathogen, providing crucial information in the early stages of infection.
Conversely, researchers involved in studying gene expression often utilize qPCR once RT-PCR has been conducted. If you're investigating how a specific gene is regulated or how its expression changes under particular conditions, qPCR can yield the quantitative data necessary to draw meaningful conclusions.
- RT-PCR Situations:
- qPCR Situations:
- Detection of viral RNA in patient samples.
- Assessing gene expression levels through initial cDNA synthesis.
- Measuring gene expression levels in different experimental conditions.
- Analyzing genetic variants through quantification of DNA.
In sum, both RT-PCR and qPCR shine in their respective domains. Choosing the right approach hinges on the specific goals of the analysis at hand. Whether you find yourself needing to identify a viral agent or quantify gene expression, understanding the distinctions between these methods will prepare you to navigate the complexities of molecular diagnostics with ease.
Recent Advances in RT-PCR Technology
Recent advances in reverse transcription PCR (RT-PCR) technology highlight its crucial role in molecular biology and diagnostics. With the continuous evolution of techniques and tools, RT-PCR has become more accurate, efficient, and relevant in various applications today. Keeping pace with the advances means understanding the nuances, potential benefits, and some considerations that come along with these new developments.
Novel Detection Methods
The landscape of RT-PCR has seen the advent of innovative detection methods that enhance sensitivity and specificity. Technologies such as digital PCR (dPCR) come into play, providing an unprecedented level of quantification. This type of PCR allows detection at a single-molecule level, which minimizes the risk of false positives and negatives. Compared to traditional methods, the inclusion of these newer techniques leads to more robust analytical tools, especially in clinical diagnostics.
Furthermore, methods like multiplex RT-PCR enable simultaneous detection of multiple targets within a single reaction. This not only conserves precious samples but also saves time and lowers costs in clinical settings. For instance, during viral outbreaks, multiplex RT-PCR can identify various pathogens in one go, thus streamlining the diagnostic process.
"The future of RT-PCR hinges on the innovative methods that reliably push the boundaries of detection sensitivity."
Real-Time Applications in Medicine
The implications of recent RT-PCR advancements extend deeply into medicine. Real-time RT-PCR has made its mark in monitoring disease progression and treatment efficacy. For example, oncologists increasingly rely on this technology to track residual disease in patients undergoing cancer treatment. It gives them a clearer picture of a patientâs response to therapy on a molecular level, enabling personalized treatment plans that adjust based on real-time data.
Moreover, the integration of RT-PCR with point-of-care testing is revolutionizing diagnostics. Devices that incorporate RT-PCR capabilities are now portable and user-friendly, pushing diagnostics from centralized labs to clinics and even home healthcare environments. Such accessibility has massive implications, especially in detecting infectious diseases.
In summary, these advances not only bolster the capabilities of RT-PCR tests but also widen their applicability. As researchers and healthcare professionals delve deeper into their potential, we can expect a more nuanced understanding of diseases, leading ultimately to improved patient outcomes and enhanced diagnostic capabilities.
Culmination
The importance of understanding reverse transcription PCR (RT-PCR) cannot be overstated, especially in the realms of research and diagnostic medicine. This article has laid out the foundational principles and applications of RT-PCR, highlighting how it efficiently transforms RNA into complementary DNA (cDNA), a step critical for subsequent amplification and analysis. The nuances exploredâfrom basic methodologies to advanced techniquesâprovide a comprehensive guide for students, educators, and professionals alike, illustrating how RT-PCR is utilized to detect viral infections, analyze gene expression, and identify genetic variants.
Among the several key points discussed, the sensitivity and specificity of RT-PCR stand out. This technique's ability to pinpoint minute quantities of target RNA makes it invaluable in clinical settings, particularly in timely diagnostics for infectious diseases. Furthermore, the rapid turn-around times associated with RT-PCR testing allow for quick health decisions, further emphasizing its role in effective public health responses.
Moreover, the technological advances in RT-PCR, such as novel detection methods and real-time applications, are driving the field forward. These developments not only enhance the accuracy and efficiency of testing but also expand the scope of applications, potentially paving the way for breakthroughs in genetic research and therapeutic interventions.
In reflecting on limiting factors, such as contamination risks and quantification challenges, the article underscores that no method is without its challenges. Awareness and mitigation of these limitations are crucial for maximizing the potential of RT-PCR in both research and clinical scenarios.
Ultimately, the integration of RT-PCR into standard laboratory practices and clinical workflows highlights its significance in modern biology and medicine. Understanding its intricacies is essential for those involved in disease diagnosis, genetic testing, and the broader landscape of molecular biology.
Summarization of Key Points
In summary, the article has unpacked several elements regarding RT-PCR:
- Conversion Process: RT-PCR effectively converts RNA into cDNA, allowing for further genetic analysis.
- Applications: It's a central technique for diagnosing viral infections and assessing gene expression.
- Advantages and Limitations: Sensitivity and speed make it effective, while contamination and quantification present challenges.
- Advancements: New technologies continue to enhance its application scope in various domains.
Future Perspectives
Looking ahead, RT-PCR is poised to play an even more critical role in various fields.
- Integrative technologies: The fusion of RT-PCR with other genomic techniques, such as next-generation sequencing, can lead to more comprehensive analyses and a deeper understanding of viral genomes and mutations.
- Point-of-care testing: The future may see increased adoption of RT-PCR in decentralized, point-of-care settings, permitting quicker diagnostics in remote locations.
- Therapeutic Applications: The continued exploration of cDNA could spur novel therapeutic approaches, providing targeted treatments in gene therapy.
- Public Health Monitoring: With the ongoing need for surveillance of infectious diseases, RT-PCR will likely become integral in tracking and responding to outbreaks, emphasizing its relevance in public health initiatives.
As research progresses, further innovation in RT-PCR methods could revolutionize diagnostics, making it an ever-evolving, vital tool in both research and clinical practice.