Understanding RNase A: Structure, Function, and Applications
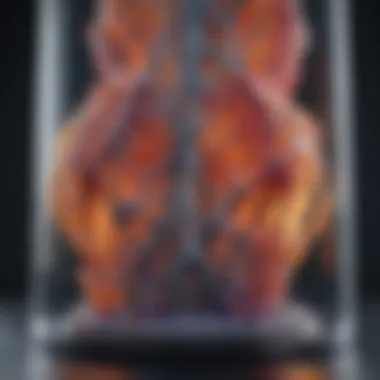
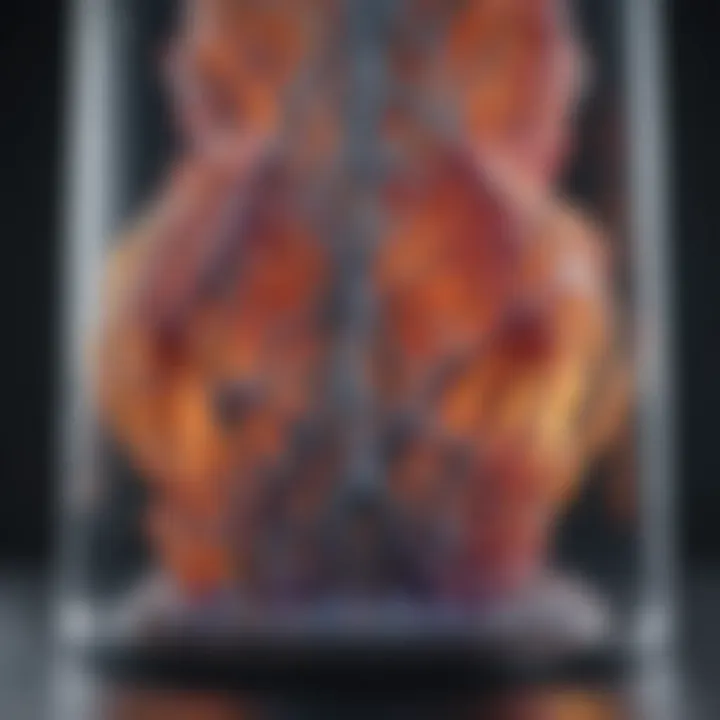
Intro
RNase A is a notable enzyme that plays a vital role in RNA metabolism. As scientists continue to explore its functions and applications, understanding the structural and biochemical properties of RNase A becomes crucial. This narrative aims to dissect its mechanisms, roles, and the broadening landscape of its applications in both biotechnological and therapeutic fields.
Research Overview
Key Findings
Recent studies have provided significant insights into RNase A. First, the enzyme is known for its efficient ability to cleave RNA. This activity is essential for various cellular processes, including RNA turnover and the regulation of gene expression. Second, the structural elucidation of RNase A revealed a robust framework that supports its catalytic functions.
Moreover, emerging research emphasizes the potential therapeutic roles of RNase A. This includes applications in cancer treatment and antiviral strategies. Researchers have demonstrated that modifying RNase A can enhance its specificity and reduce off-target effects, marking progress in medical science.
Study Methodology
The research surrounding RNase A typically employs both in vitro and in vivo methodologies. Common techniques include X-ray crystallography for structural determination and biochemical assays to evaluate enzymatic activity. Additionally, molecular biology techniques, such as site-directed mutagenesis, are frequently applied to investigate the functional aspects of various protein residues. These methodologies allow researchers to paint a comprehensive picture of how RNase A operates within cellular contexts.
Background and Context
Historical Background
The history of RNase A dates back to its initial discovery in the 1950s. Early studies focused on its role in cellular RNA degradation. Since then, ample research has been dedicated to understanding its biochemical mechanisms. The enzyme's ability to hydrolyze RNA at specific nucleotide positions has fascinated scientists for decades, leading to a deeper understanding of RNA metabolism.
Current Trends in the Field
Currently, the study of RNase A is evolving. The field is witnessing a convergence of biochemistry, molecular biology, and biotechnology. Innovations in protein engineering have spurred interest in creating modified RNase A variants with enhanced properties. Furthermore, the integration of RNase A in novel delivery systems for therapeutic agents shows potential in addressing medical challenges.
In summary, RNase A is not just an enzyme of historical significance but a focal point in modern research. Its versatility in both fundamental biology and applied sciences makes it a topic of continued investigations. The importance of exploring its structure, function, and potential applications cannot be overstated as it holds promise for future advancements in various scientific domains.
Prolusion to RNase A
RNase A is not just another enzyme; it plays a pivotal role in RNA metabolism, impacting various cellular processes. Understanding RNase A provides crucial insights into how cells manage their genetic material. This section will explore its significance and offer an overview of what will follow in the article.
Definition and Overview
RNase A, or Ribonuclease A, is an endonuclease that catalyzes the degradation of RNA. This enzyme is primarily known for its ability to cleave single-stranded RNA, which is paramount in regulating RNA levels within cells. RNase A belongs to a family of ribonucleases that are widely studied for their functional diversity and biochemical properties. They are essential for various processes, including the maintenance of cellular RNA homeostasis.
In physiological terms, RNase A helps eliminate defective or unnecessary RNA, thus ensuring the proper functioning of cellular machinery. This activity is critical for RNA metabolism, as it directly influences the availability of RNA for translation and various regulatory functions. Moreover, RNase A’s ability to target RNA is leveraged in numerous biotechnological applications.
Historical Context
The discovery of RNase A dates back to the mid-20th century when its unique properties began to intrigue scientists. Initially isolated from bovine pancreas in the 1950s, it was a significant milestone that opened avenues for research into ribonucleases. The early studies focused on its enzymatic activity and substrate specificity. Researchers were keen to understand its structural aspects to illuminate the molecular basis of its function.
Over the decades, various techniques, including X-ray crystallography, have elucidated the intricate molecular structure of RNase A. These structural insights have played a crucial role in biotechnology, as they provide a framework for developing innovative applications. The historical trajectory of RNase A research reflects its transition from a mere subject of academic inquiry to an essential component in modern molecular biology and therapeutic development.
By grounding the discussion in the definition and historical context of RNase A, the subsequent sections will delve deeper into its biochemical properties, biological significance, and its applications in biotechnology and research. This journey through the functionalities and peculiarities of RNase A highlights not only its scientific value but also its practical implications in the field of molecular biology.
Biochemical Properties of RNase A
Understanding the biochemical properties of RNase A is essential as it provides insight into its roles in RNA metabolism and its applications in biotechnology. Analyzing these properties reveals how RNase A functions at a molecular level, its interaction with substrates, and how its structure contributes to its enzymatic activity. This section covers the molecular structure and catalytic mechanisms of RNase A, emphasizing their significance in both fundamental research and practical applications.
Molecular Structure
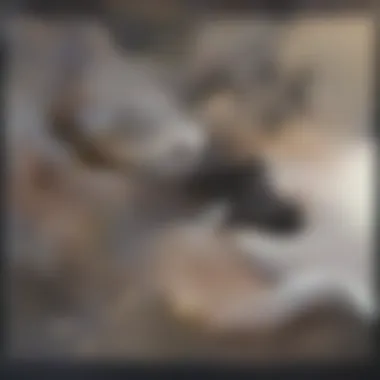
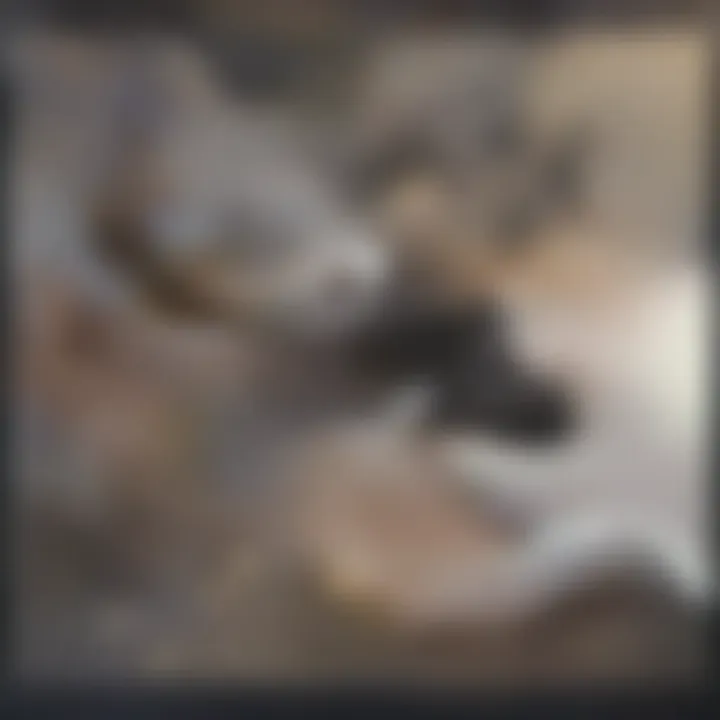
Primary Structure
The primary structure of RNase A refers to the specific sequence of amino acids in the polypeptide chain. This sequence is crucial as it determines the enzyme's overall function and activity. RNase A consists of 124 amino acids, and its primary structure includes several key motifs that are common among ribonucleases. A notable characteristic of this primary structure is the presence of a high number of positively charged residues, which contribute to the enzyme's ability to interact with negatively charged RNA substrates. This feature is beneficial for RNase A's binding and catalytic action. However, the specific sequence can also determine the enzyme's susceptibility to degradation or inhibition by various factors, which may pose challenges in certain experimental settings.
Secondary Structure
The secondary structure of RNase A involves the local folding patterns formed by hydrogen bonding among amino acids. Specifically, the enzyme predominantly exhibits alpha-helix and beta-sheet formations. This arrangement stabilizes the molecule and is essential for its functionality. The key characteristic of the secondary structure is its compact configuration, which provides the necessary architecture for the active site. Such a structure is advantageous as it fosters efficient substrate binding and catalysis. Nevertheless, the rigid nature of certain secondary structure elements may limit the enzyme’s flexibility, which is sometimes needed for effective substrate interaction.
Tertiary Structure
The tertiary structure describes the overall three-dimensional shape of RNase A, determined by interactions among the amino acid side chains. The enzyme's tertiary structure reveals a well-defined active site that is shaped to accommodate RNA. A prominent feature is the formation of disulfide bridges, which contribute to the stability of the enzyme under varying conditions. This high stability is vital for its functionality in diverse environments, including those found in biotechnological applications. However, this stability might also lead to decreased dynamics, limiting the enzyme’s adaptability to different RNA substrates or conditions, which could be a disadvantage in some experimental scenarios.
Catalytic Mechanism
Substrate Specificity
Substrate specificity refers to the ability of RNase A to recognize and cleave particular RNA molecules. RNase A primarily targets single-stranded RNA and prefers to cleave at the phosphodiester bonds adjacent to pyrimidine nucleotides. This characteristic is essential for its biological function, allowing it to selectively degrade RNA in various cellular processes. The specificity for pyrimidines is notable because it ensures efficiency in RNA metabolism. However, this limited specificity can sometimes hinder its utility in applications requiring broader activity against diverse RNA types.
Active Site Configuration
The active site configuration of RNase A is meticulously designed to facilitate the catalytic process. This site includes essential amino acid residues that engage in substrate binding and catalysis. The arrangement of these residues is crucial, as it directly impacts the enzyme’s efficiency and speed of reaction. The configuration of the active site makes RNase A a beneficial tool in molecular biology, particularly in RNA analysis. However, mutations or alterations in this configuration can significantly impact enzyme activity, presenting challenges for certain applications.
Enzyme Kinetics
Enzyme kinetics studies the rates of enzymatic reactions and is fundamental to understanding RNase A's behavior in various conditions. The kinetic parameters, such as Michaelis-Menten constants, provide valuable insights into the efficiency and potential applications of RNase A in experimental protocols. A key characteristic of its kinetics is its relatively high catalytic efficiency, making it a popular choice in research and biotechnology. Nevertheless, enzyme kinetics can vary widely based on environmental conditions such as pH and temperature, which can either enhance or inhibit its activity.
Understanding the biochemical properties of RNase A not only contributes to fundamental research but also enhances its applications in biotechnology, impacting fields such as molecular cloning and gene therapy.
The detailed examination of the biochemical properties of RNase A illustrates its complexity and importance in molecular biology, offering significant insight into its role in various biological processes and technological applications.
Biological Significance of RNase A
The biological significance of RNase A is profound, as this enzyme plays a critical role in various cellular processes. By facilitating RNA metabolism, it not only ensures the degradation of unnecessary RNA but also regulates the overall balance within the cell. Understanding these functions is essential for anyone studying molecular biology or related fields.
Role in RNA Metabolism
RNA Degradation
RNA degradation is a vital process for maintaining cellular homeostasis. RNase A contributes to this by breaking down RNA molecules, thus controlling their levels within the cell. This reduction is important because excess RNA can lead to cellular malfunctions and interfere with protein synthesis. The key characteristic of RNA degradation by RNase A is its specificity for RNA substrates over other biomolecules. This selective action helps to prevent unregulated accumulation of RNA. Moreover, the activity of RNase A helps in recycling nucleotide components, which can then be reused in other cellular processes. However, while RNA degradation promotes cellular efficiency, excessive degradation can result in the loss of important genetic information, which is a disadvantage worth noting.
Regulatory Mechanisms
Regulatory mechanisms involving RNase A further establish its significance in RNA metabolism. The enzyme is not merely a passive participant; it also serves as a regulator of various biological functions by modulating RNA levels. This function is particularly relevant during stress responses where RNase A can alter the availability of specific RNA molecules, impacting gene expression. The key characteristic of this regulatory mechanism lies in its adaptive nature, allowing cells to respond to environmental changes effectively. A unique feature of RNase A’s regulatory role is its interaction with other molecules that may enhance or inhibit its activity. This multifaceted approach can be both an advantage, allowing for intricate control of cellular pathways, and a potential challenge if not properly regulated, leading to disruption of cellular homeostasis.
Impact on Cellular Processes
Cell Growth
Cell growth is another significant area affected by RNase A. By regulating RNA metabolism, RNase A indirectly influences the synthesis of proteins necessary for cell proliferation. A balanced availability of RNA ensures that cells can grow and divide properly. The importance of RNase A in promoting normal growth patterns makes it a beneficial focus of study in oncology. The unique feature of this relationship is the dependency on precise regulation of RNase A activity; either insufficient or excessive activity can disrupt normal cell growth, leading to pathological conditions.
Death and Immune Response
The role of RNase A in cell death and immune response showcases its versatility. It is involved in apoptotic processes, providing a way to eliminate damaged or unrequired cells. This function is essential for maintaining tissue integrity. Additionally, RNase A has been shown to play a part in modulating the immune response. By degrading viral RNA, it helps to reduce the potential impact of viral infections on cells. The characteristic of RNase A to initiate cell death under certain circumstances is critical for overall health. However, this same mechanism can be detrimental if cells that should remain alive are prematurely degraded, highlighting a delicate balance in its functional role.
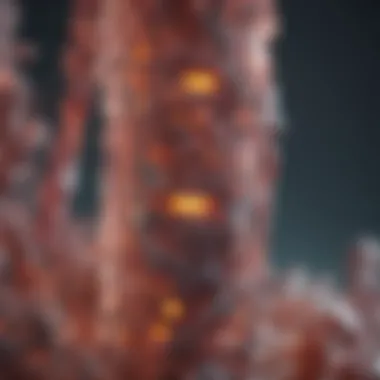
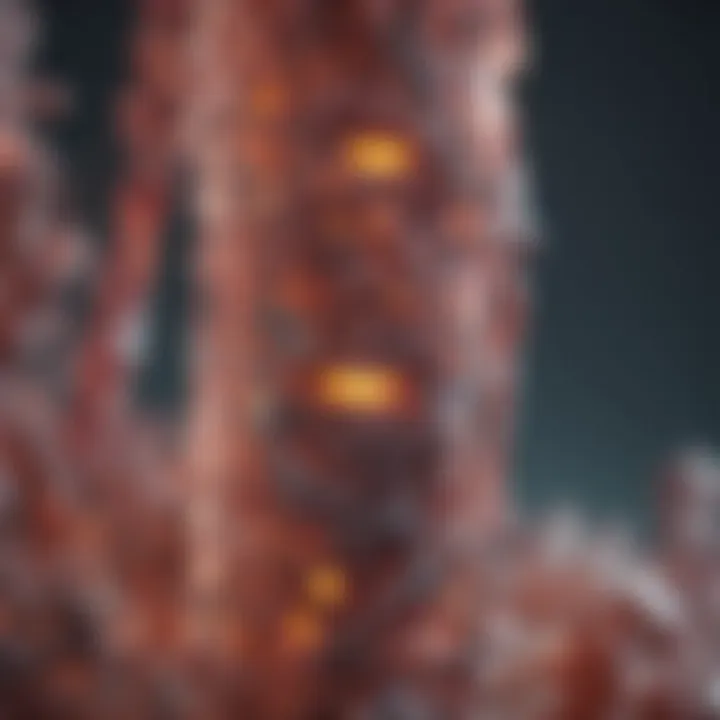
Applications of RNase A in Biotechnology
The applications of RNase A in biotechnology showcase its versatility and significance in various scientific fields. This enzyme is not just essential for basic research but also provides foundational support for innovation in molecular biology and medical technology. Understanding the diverse applications of RNase A helps to further signal its importance in advancing biotechnological practices. Its role in molecular cloning, gene therapy, and diagnostics illustrates not only its functionality but also its pivotal importance in modern science.
Molecular Cloning
Molecular cloning is a fundamental technique in genetic engineering that involves creating copies of specific DNA fragments. RNase A is often used in this context to degrade RNA during the cloning process. This is crucial because any RNA present can interfere with the reactions needed for successful cloning. By removing RNA, researchers can ensure a higher purity of DNA, which in turn increases the efficiency of subsequent cloning steps.
- Benefits:
- Ensures clean and uncontaminated DNA samples.
- Facilitates better transformation efficiency in host cells.
- Improves the reliability of downstream applications, such as sequencing.
Moreover, RNase A helps in minimizing RNA interference in libraries of cDNA, leading to more accurate results. In summary, using RNase A in molecular cloning enhances the effectiveness of the process, thereby enabling advanced research and applications in genetic engineering.
Gene Therapy
Gene therapy aims to treat or prevent diseases by altering the genetic makeup of a patient. RNase A plays a significant role in this emerging field. It is often utilized in the delivery of therapeutic RNA molecules, such as small interfering RNA (siRNA), which are designed to silence specific genes associated with disease.
- Specific Applications:
- Facilitating the removal of unwanted RNA species from therapeutic preparations.
- Enhancing the stability and efficacy of siRNA in biological systems.
The ability of RNase A to selectively degrade RNA can also help regulate the expression of therapeutic genes. Decreasing the levels of certain RNA can mitigate potential side effects, making the therapy more effective. Therefore, RNase A's role in gene therapy is a prime example of how enzyme applications can pave the way for novel therapeutic strategies.
Diagnostics and Therapeutics
In the fields of diagnostics and therapeutics, RNase A is valuable for developing assays and treatment protocols. Its enzyme properties are harnessed in the detection of RNA-based pathogens, a crucial aspect of infectious disease diagnostics. For instance, the ability of RNase A to degrade RNA is particularly useful in assays that identify viral RNA in clinical specimens.
- Key Considerations:
- Enhances specificity in RNA detection assays.
- Can be integrated into diagnostic kits for rapid testing.
- Plays a role in RNA-based therapeutic modalities.
Furthermore, RNase A applications extend to therapeutic contexts, such as targeted RNA therapy, where it assists in the destruction of pathogenic RNA. This dual role in diagnostics and therapeutics underscores RNase A's essential contributions to public health.
In summary, RNase A serves as a pivotal tool in various biotechnological applications, enhancing molecular cloning, enabling gene therapy advancements, and supporting diagnostic innovations.
Ultimately, the applications of RNase A in biotechnology reflect its adaptability and utility, offering profound insights into both the challenges and directions of contemporary research.
Research Advances Involving RNase A
Research advances involving RNase A highlight significant strides in understanding its biochemical properties and applications. These advances not only deepen our knowledge about RNase A but also open new avenues for therapeutic interventions and molecular biology applications. Investigating RNase A is essential due to its role in RNA metabolism, and it serves as a model system for understanding enzymatic functions and interactions.
Experimental Techniques
Structural Biology Approaches
Structural biology approaches play a crucial role in elucidating the three-dimensional structure of RNase A. Techniques like X-ray crystallography and nuclear magnetic resonance (NMR) spectroscopy provide detailed insights into its molecular arrangement. The key characteristic of these approaches is their ability to reveal atomic-level details, which are vital for deciphering the enzyme's function.
Structural biology is popular in studying RNase A because it offers a direct view of how the enzyme interacts with RNA substrates. Each method has unique features; for instance, X-ray crystallography can yield high-resolution structures but often requires crystallization of the enzyme. In contrast, NMR is less complex regarding sample preparation but may offer lower resolution. Despite these differences, both techniques provide valuable information on RNase A's active site and binding interactions, thus enhancing our understanding of its catalytic mechanism.
Kinetic Studies
Kinetic studies allow scientists to assess the rates of biochemical reactions involving RNase A. This technique measures how quickly the enzyme catalyzes RNA degradation, providing insights into its efficiency and substrate specificity. A key characteristic of kinetic studies is their ability to quantify enzymatic activity under varying conditions, such as pH and temperature. This makes them a beneficial choice in understanding how external factors influence RNase A's performance.
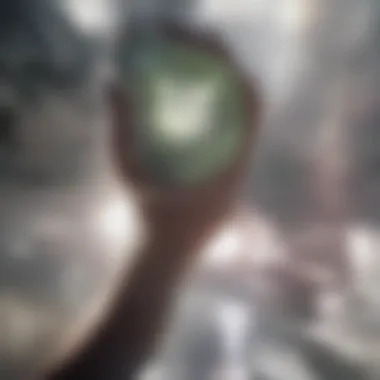
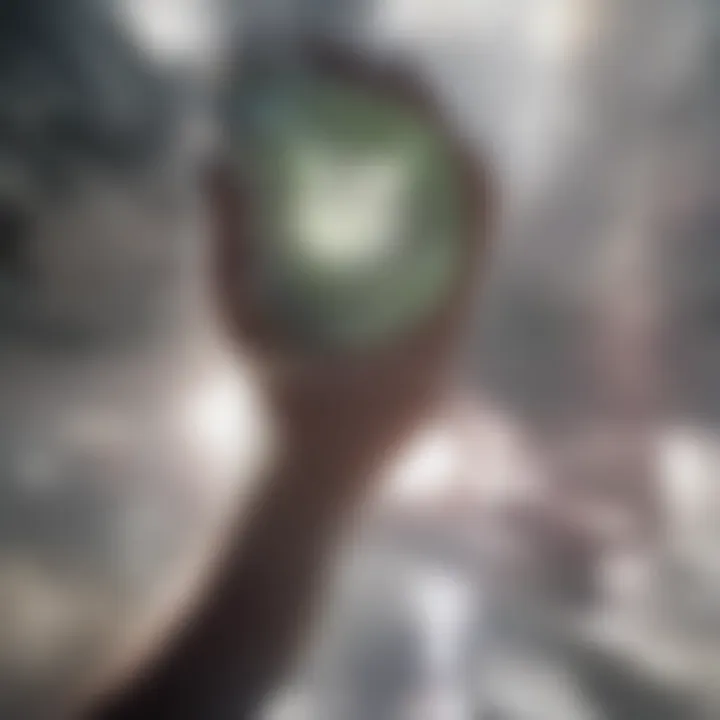
The unique feature of kinetic studies lies in their application of mathematical models, which can predict the reaction dynamics of RNase A. For example, Michaelis-Menten kinetics is often used to analyze enzyme behavior. One advantage is the ability to conduct real-time monitoring of substrate conversion, helping researchers design experiments based on real-time data. However, a potential disadvantage is the requirement for precise measurements, which can introduce variability if not managed carefully.
Emerging Applications
Targeting Cancer Cells
Targeting cancer cells is a promising application of RNase A, especially due to its ability to degrade RNA within these cells. This targeted approach aims to selectively eliminate cancerous cells while minimizing damage to normal tissues. The distinguishing feature of this application is its specificity; studies suggest that RNase A can identify and bind to altered RNA structures commonly found in malignant cells.
Using RNase A for cancer therapy offers benefits, such as reduced side effects compared to traditional chemotherapies, which often damage healthy cells. However, challenges exist in effectively delivering the enzyme to the target site within the body. Currently, researchers are exploring various delivery methods, including nanoparticle systems, to enhance the therapeutic efficacy of RNase A in oncological settings.
Interfering RNA Mechanisms
Interfering RNA mechanisms represent another significant advance involving RNase A. This application revolves around using the enzyme to manipulate RNA interference pathways, which are crucial for gene regulation. A critical feature of this approach is its ability to enhance the knockdown efficacy of target genes, thereby improving the outcomes of genetic therapies.
The unique aspect of employing RNase A in this context lies in its potential to facilitate the degradation of unwanted RNA molecules, thus reinforcing the RNA interference mechanism. The advantages include precise control over gene expression, making it a popular choice in gene therapy research. Nonetheless, the complexity of RNA interaction networks poses challenges, which require deeper investigation to maximize the effectiveness of RNase A in regulating gene activity.
The Evolutionary Perspective of RNase A
Understanding the evolutionary context of RNase A can provide significant insights into its current functions and applications. By examining its phylogenetic relationships and adaptations, researchers can better appreciate how RNase A has evolved to meet the needs of different organisms. This perspective also aids in recognizing the enzyme's historical roles and the factors that have shaped its molecular characteristics over time.
Phylogenetic Analysis
Phylogenetic analysis of RNase A reveals its place within the broader context of ribonucleases. This analysis often employs techniques like DNA sequencing and comparative genomics to trace the evolutionary lineage of the enzyme across different species.
Utilizing molecular phylogenetics, scientists can create evolutionary trees that illustrate how diverse organisms have developed unique RNase A variants. For instance:
- Comparison with Other Ribonucleases: RNase A shares structural similarities with other ribonucleases, such as RNase T1 and RNase H, suggesting a common ancestor.
- Species-Specific Variants: Variants of RNase A exist in various mammals, with notable differences that may reflect adaptations to specific ecological niches.
- Conservation Across Species: Some motifs in the RNase A sequence are highly conserved among distant species, indicating important functional roles that have persisted throughout evolution.
These patterns are essential for understanding the functional diversity of RNase A among different taxa.
Evolutionary Adaptations
The adaptability of RNase A across various organisms highlights its evolutionary significance. Over time, RNase A has undergone several adaptations in response to environmental pressures and physiological needs.
Key adaptations include:
- Substrate Specificity Changes: Variants of RNase A have developed enhanced substrate preferences, allowing them to target specific RNA types or structures more effectively.
- Regulatory Mechanisms: Evolution has optimized the regulation of RNase A activity, ensuring that its action is tightly controlled in response to cellular conditions.
- Role in Defense Mechanisms: In certain organisms, RNase A plays a role in immune responses, showcasing its functional versatility.
"The evolutionary journey of RNase A illustrates how molecular adaptations can lead to sophisticated solutions for biological challenges."
Culmination
The conclusion of this article offers a synthesis of the critical insights regarding RNase A, underlining its significance within the realm of molecular biology. RNase A is not merely an enzyme; it is a fundamental component of RNA metabolism that bridges the gap between basic biological function and applied biotechnology. This section will emphasize several pivotal elements that enhance the reader's comprehension of RNase A's multifaceted roles.
Summary of Key Points
In this article, we have traversed various aspects of RNase A, highlighting its biochemical properties, biological significance, and numerous applications. Here are the main points:
- Biochemical Properties: RNase A exhibits unique molecular structures that dictate its functionality. Understanding its primary, secondary, and tertiary structures provides insights into its catalytic capabilities.
- Biological Significance: The enzyme plays an essential role in RNA degradation and regulates cellular processes such as growth and immune responses.
- Applications in Biotechnology: Its utility extends to molecular cloning, gene therapy, and diagnostic methodologies, demonstrating its importance in modern science.
- Research Advances: Recent studies emphasize novel experimental techniques and emerging applications targeting cancer and RNA mechanisms, showcasing the enzyme's future potential.
The interplay of these elements marks RNase A as a central figure in myriad biochemical processes and biotechnology applications.
Future Directions for Research
Looking ahead, research on RNase A holds promising prospects. Several areas warrant further exploration:
- Targeting Novel Applications: As our understanding grows, exploiting RNase A in the development of tailored cancer therapies could be transformative. Investigating its interaction with tumor microenvironments could yield breakthroughs in treatment strategies.
- Structural Studies: Enhancing knowledge about RNase A’s structural dynamics can improve its application in biotechnology. Advanced techniques like cryo-electron microscopy may reveal significant insights.
- Genetic Engineering: Modifying RNase A could lead to better specificity and efficiency in therapeutic uses. Researching its potential in gene editing presents exciting avenues for innovation.
- Ecological Studies: Examining RNase A in various organisms may showcase evolutionary adaptations that could influence biomedical applications.
Engaging in these research directions will not only further elucidate the biological roles of RNase A but also contribute to advancements in health and technology. The future of RNase A research is bright, with the potential for significant implications in our understanding of molecular processes and their applications.