Understanding T7 Polymerase Buffer: Key Insights
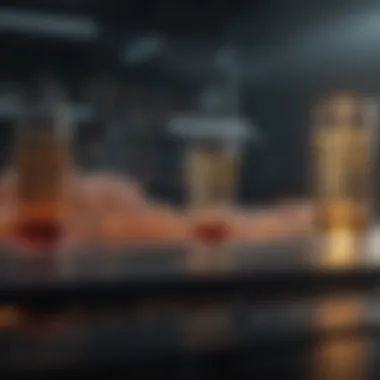
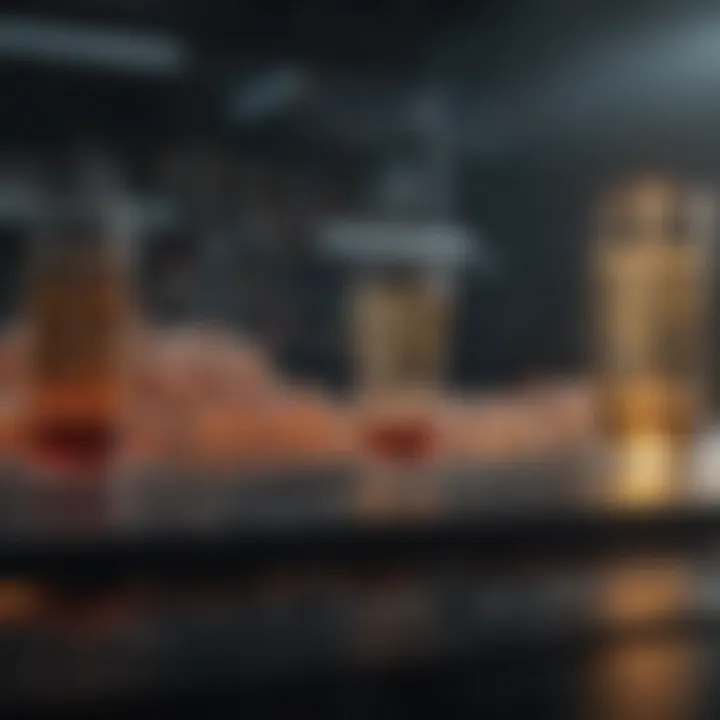
Intro
T7 polymerase is a crucial enzyme in molecular biology, widely utilized for DNA and RNA synthesis. A well-optimized T7 polymerase buffer is essential for enhancing the efficiency and specificity of these reactions. Understanding the composition and mechanisms of the buffer helps researchers design and execute experiments more effectively. This section introduces key elements that impact polymerase activity, ultimately improving the fidelity and yield of nucleic acid products.
Research Overview
Key Findings
T7 polymerase operates optimally under specific conditions influenced by buffer composition. The ratio of salts, the pH level, and the presence of essential cofactors like magnesium ions significantly affect enzyme functionality. Recent studies reveal that slight modifications in these parameters can lead to substantial improvements in synthesis outcomes. Key findings indicate that:
- Ionic strength plays a pivotal role in stabilizing the enzyme-DNA complex.
- pH levels must be maintained within a narrow range to prevent denaturation of the enzyme.
- The inclusion of additives, such as BSA (Bovine Serum Albumin), can enhance reaction efficiency by reducing non-specific binding.
Study Methodology
This exploration employs a combination of literature review and experimental data analysis. By examining various articles and studies related to T7 polymerase buffer, we drive insights into optimal conditions. Researchers outline their methodologies, including buffer preparation and assay conditions, providing a comprehensive approach to understanding polymerase performance and the impact of differing buffer compositions.
Background and Context
Historical Background
The T7 polymerase was isolated from the T7 bacteriophage and has since been refined for laboratory applications. Developed in the 1970s, it has continually evolved through various studies. Understanding its buffer needs was critical to its use in molecular cloning and transcription.
Current Trends in the Field
Molecular biology now leans towards more practical applications of T7 polymerase buffers, especially in the context of synthetic biology and therapeutic development. Researchers strive for buffers allowing high fidelity and efficiency, thus minimizing errors during nucleic acid synthesis. With advancements in genetic engineering, users of T7 polymerase need buffers that adapt to modern multi-component reactions, which may involve simultaneous synthesis of RNA and DNA.
"The accurate composition of T7 polymerase buffer can dramatically influence the yield and purity of nucleic acid products."
In summary, examining the role of T7 polymerase buffer provides invaluable insights into the optimization of nucleic acid synthesis, addressing both historical and contemporary practices. The upcoming sections further explore the specific compositions of buffers and their respective applications in experimental setups.
Intro to T7 Polymerase
The significance of T7 polymerase in modern molecular biology cannot be overstated. This enzyme, derived from the T7 bacteriophage, acts as a cornerstone for various applications in genetic research and biotechnology. It is primarily recognized for its high fidelity and efficiency in synthesizing RNA from DNA templates. Understanding T7 polymerase lays the foundation for exploring its buffer, which plays a crucial role in optimizing the enzyme's activity.
T7 polymerase operates under specific conditions that involve various biochemical constituents, including buffers that create ideal environments for enzyme reactions. Knowledge of how these factors interact enables researchers to tailor experiments for increased success rates. Factors such as pH, ionic strength, and concentration of nucleotides are critical in every aspect of experimental design. These elements enhance the performance of T7 polymerase and ultimately influence the quality of the nucleic acids produced.
Furthermore, the applications of T7 polymerase extend beyond basic research to include practical uses in diagnostics, therapeutics, and synthetic biology. The ability to understand and manipulate conditions surrounding T7 polymerase allows for innovations that can propel advancements in these fields. The next sections will dive into the details, beginning with an overview of the polymerase itself, providing insight into its components and roles.
Understanding Polymerase Buffers
The selection of the right polymerase buffer is critical in molecular biology experiments. Buffers play a crucial role in optimizing enzymatic reactions, such as those involving T7 polymerase. Without an appropriate buffer, the efficiency of reactions can significantly decrease, leading to poor yields or inconsistent results.
When it comes to polymerase activity, buffers maintain the right environment for enzymes to function optimally. Understanding the nuances of these buffers is essential for anyone involved in DNA synthesis or transcription studies. They regulate factors like pH, ionic strength, and the presence of necessary ions that impact the overall activity of the polymerase. A good grasp of these elements helps researchers and students design better experiments.
Role of Buffers in Enzymatic Reactions
Buffers serve multiple functions in enzymatic reactions. They maintain a consistent pH, which is vital since many enzymes, including T7 polymerase, have an optimal pH range. Deviations from this pH can lead to denaturation or inactivity of the enzyme. Additionally, buffers can help stabilize the reaction by controlling the ionic strength. This is significant in preventing non-specific interactions and ensuring that the polymerase efficiently binds to the nucleic acids.
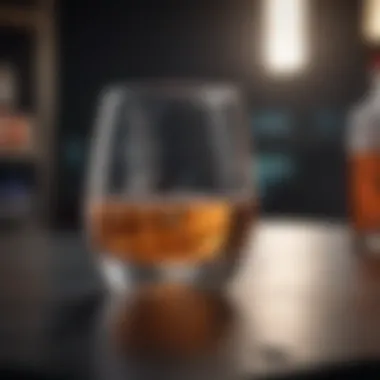
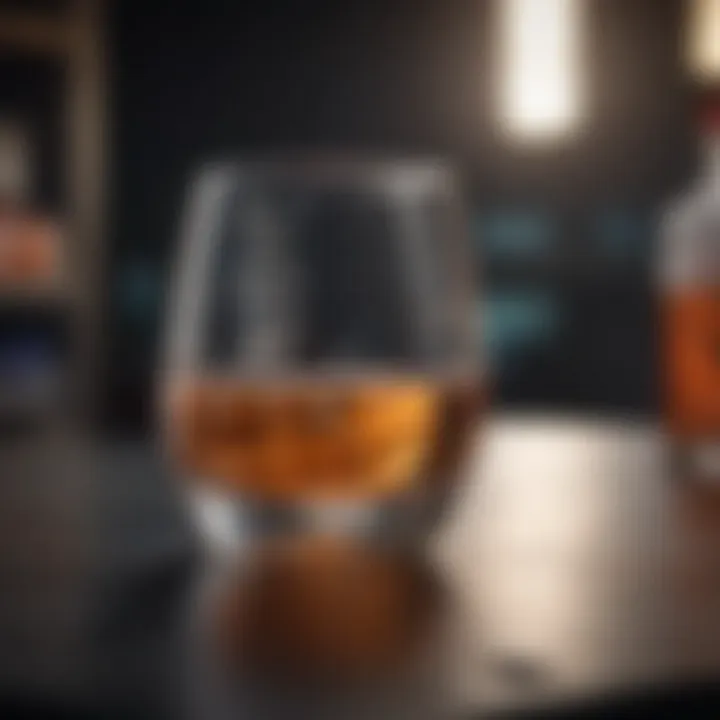
Furthermore, buffers enhance the solubility of all components within the reaction mixture. They can also affect the overall kinetics of the reaction, which can significantly impact experimental outcomes. Thus, choosing the right buffer composition becomes a strategic decision that can streamline success in molecular experiments.
Key Components of Polymerase Buffers
The composition of polymerase buffers includes several key components that strongly influence the reaction conditions. The commonly used components are:
- Tris-HCl: This compound is essential for maintaining stable pH levels within a desired range, typically around 7.5 to 8.5. It helps resist fluctuations in acidity or alkalinity, ensuring that the reaction remains optimal.
- KCl: Potassium chloride provides the necessary ionic strength. It assists in the stabilization of DNA and RNA interactions, which are crucial for polymerase activity.
- MgCl2: Magnesium chloride is required as a cofactor for polymerase activity. It activates the polymerase enzyme and aids in the polymerization of nucleotides, which is fundamental in DNA synthesis.
- dNTPs: Deoxynucleotide triphosphates are the building blocks of DNA. They are necessary for the synthesis process and must be present in sufficient quantities for the polymerase to perform its function.
Understanding these components is vital when preparing a buffer for T7 polymerase reactions. Each one plays a distinct role, impacting the overall performance of the polymerase.
In molecular biology, the choice of buffer and its composition can significantly alter experimental outcomes.
By learning about the function and necessity of these components, researchers can effectively troubleshoot problems and optimize their experimental protocols. Proper buffer composition not only enhances polymerase activity but also increases the reliability of results in research findings.
Components of T7 Polymerase Buffer
In molecular biology, understanding the components of T7 Polymerase buffer is crucial. These components determine the efficiency and specificity of the polymerase enzyme during nucleic acid synthesis. Each element plays a distinct role, optimizing conditions tailored to specific applications. Researchers benefit from a clear grasp of these components, as variations can lead to significant improvements in experimental outcomes.
Tris-HCl: pH Regulation
Tris-HCl is a buffer that stabilizes the pH during reactions involving T7 Polymerase. The enzyme has an optimal activity at a specific pH range, typically around 7.5 to 8.0. pH fluctuations can affect enzyme activity, resulting in decreased efficiency. This is crucial in maintaining the desired conditions throughout the duration of the reaction. By carefully choosing the Tris-HCl concentration, researchers can enhance the yield and accuracy of their synthesis, ensuring a stable environment for polymerization.
KCl: Ionic Strength and Its Effects
Potassium chloride (KCl) is essential for maintaining the ionic strength of the buffer. This property is key for the enzyme's binding affinity to the DNA template. The presence of KCl can influence the enzyme's stability and activity by creating an appropriate environment for the DNA molecule. For instance, increasing KCl concentration tends to improve T7 Polymerase's performance, but it must be monitored closely, as excessive ionic strength might inhibit enzyme function. Using KCl wisely can lead to optimal enzyme behavior.
MgCl2: Role in Polymerization
Magnesium chloride (MgCl2) serves as a cofactor necessary for the polymerization reaction. It is involved in the activation of the nucleotide substrate, providing the necessary ions for the formation of phosphodiester bonds during DNA synthesis. Without adequate concentrations of MgCl2, the polymerase may not function effectively, leading to reduced efficiencies in synthesizing DNA. Therefore, it is vital to balance MgCl2 levels according to the specific reaction requirements.
dNTPs: Building Blocks of DNA
dNTPs, or deoxynucleotide triphosphates, are the essential building blocks for DNA synthesis. Each dNTP corresponds to one of the four nucleotides required for DNA assembly: dATP, dCTP, dGTP, and dTTP. The concentrations of these nucleotides in the buffer significantly impact the rate and fidelity of DNA synthesis. If dNTP concentrations are insufficient, primer elongation cannot occur efficiently. Sufficient dNTP availability enables the T7 Polymerase to produce high-quality DNA, crucial for downstream applications such as cloning or sequencing.
Effective optimization of these components is key for successful T7 Polymerase reactions. Researchers should carefully consider the interactions between each element to design their experiments, maximizing efficiency and yield.
Optimal Conditions for T7 Polymerase Activity
Optimal conditions for T7 polymerase activity are crucial in ensuring the efficiency of transcription and related reactions. Understanding these parameters allows researchers to fine-tune experimental setups. Key elements include temperature, pH balance, and ionic strength. Adjustments in these factors help maximize the activity of T7 polymerase, thus improving outcomes in nucleic acid synthesis. They not only influence the enzyme's kinetics but also impact overall reaction yield.
Temperature Effects
Temperature plays a significant role in enzymatic reactions. T7 polymerase has a defined optimum temperature around 37 degrees Celsius. At this temperature, the enzyme exhibits maximum activity. When temperatures rise above 40 degrees Celsius, there is often a noticeable decrease in polymerase function. Enzymes like T7 polymerase can denature, losing their functional three-dimensional shape. This degradation is reversible to an extent, but prolonged exposure to high temperatures can lead to irreversible damage.
Conversely, very low temperatures can also be detrimental. While they may slow down reactions, they can lead to reduced polymerization rates. It is thus important to maintain a balance during reaction setup to avoid suboptimal conditions. For a successful experiment, keeping the working temperature around the optimum allows for greater efficiency and better yields.
pH Balance Considerations
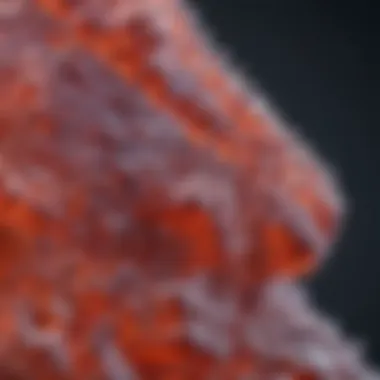
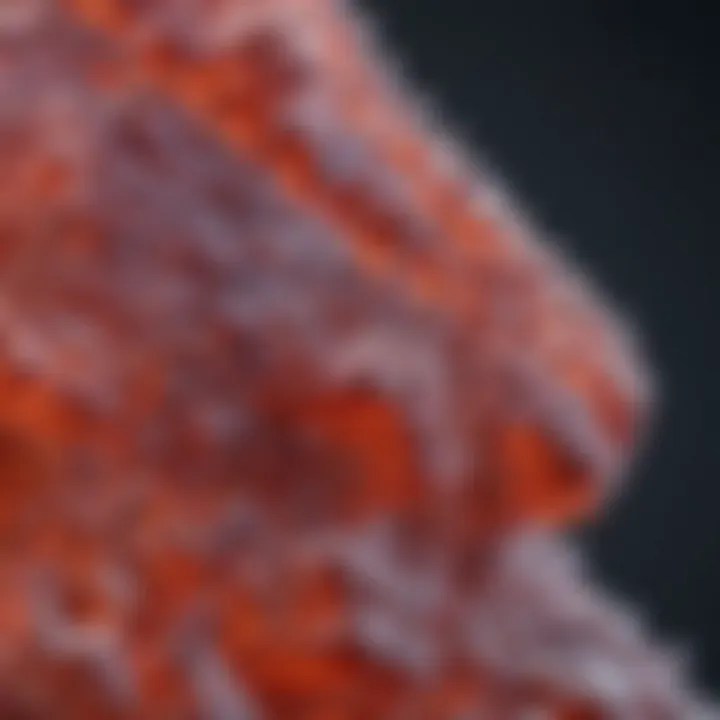
The pH of the reaction environment can drastically affect enzyme activity. T7 polymerase functions best in a buffer system that typically maintains a pH of around 7.5 to 8.5. Within this range, the enzyme maintains stability and activity. Deviations from this pH range can lead to reduced enzymatic efficiency.
Buffers like Tris-HCl help stabilize the pH during reactions. A stable pH minimizes the risk of protonation or deprotonation of active site residues, which can inhibit enzyme-substrate interactions. Regular monitoring of pH during experiments is essential. Using appropriate indicators or pH meters can aid in achieving the ideal balance to ensure optimal polymerization processes.
Ionic Strength Adjustments
Ionic strength is an important aspect often overlooked. It influences the stability of enzyme-substrate complexes, which directly affects reaction rates. T7 polymerase typically performs better with an ionic strength around 100 mM KCl. Adjusting the concentration of potassium chloride in buffer solutions can effectively modulate this strength.
Excessively high ionic strength may lead to enzyme precipitation, while too low can destabilize the substrate binding. Careful consideration of ionic composition during preparation of buffer solutions is essential. Optimizing ionic strength can enhance the interactions between T7 polymerase and its substrates, resulting in higher efficiency in transcription processes.
Carefully controlling optimal conditions will significantly enhance the utility of T7 polymerase in experimental applications, leading to more accurate and reliable results.
Applications of T7 Polymerase Buffer
The T7 polymerase buffer is pivotal in various molecular biology applications, directly influencing the efficiency and accuracy of experiments. Understanding its applications facilitates optimized experimental designs, leading to more reliable results. This section elaborates on three primary domains: in vitro transcription studies, DNA amplification techniques, and gene cloning protocols. Each application capitalizes on the unique properties of the T7 polymerase buffer to achieve specific experimental goals.
In Vitro Transcription Studies
In vitro transcription utilizes T7 polymerase to synthesize RNA from a DNA template. This method is vital for producing RNA probes, mRNA for translation studies, or even synthesizing RNA for vaccine development. The composition of the T7 buffer—especially its pH and ionic strength—ensures optimal activity of T7 polymerase. When adjusting conditions, researchers must be meticulous, as slight variations can lead to significant impacts on RNA yield and integrity.
For successful in vitro transcription, a well-balanced buffer is crucial. The presence of MgCl2 promotes proper folding and catalysis, enabling efficient RNA synthesis. Regular monitoring of reaction conditions is also recommended. Temperature and ionic conditions must typically be optimal to maintain T7 polymerase stability throughout the reaction duration.
DNA Amplification Techniques
T7 polymerase buffer finds essential use in various DNA amplification methods, including polymerase chain reaction (PCR). Its formulation allows for robust amplification of target sequences. In this application, the buffer's role extends beyond simply providing a conducive environment for the enzyme. The high fidelity of T7 polymerase, especially with the right buffer, can enhance specificity and yield in amplification processes.
Maintaining a consistent buffer composition is key. The buffering capacity can affect the behavior of DNA templates during denaturation and annealing stages of PCR. Fine-tuning ionic concentrations can help mitigate issues such as nonspecific amplification or mispriming, leading to cleaner and more interpretable results.
Gene Cloning Protocols
Gene cloning often relies on the efficiency of T7 polymerase and its buffer. This application usually involves inserting a gene of interest into a plasmid vector, enabling further studies or applications such as protein expression. The T7 polymerase buffer aids in ensuring the efficiency of constructing recombinant DNA.
Proper buffer conditions allow for better ligation and transformation efficiencies. When using vectors designed to utilize T7 polymerase, researchers can quickly generate the desired plasmids. Additionally, the buffer's components provide a stable environment that favors quick and accurate replication, reducing the likelihood of errors during the cloning process.
Key takeaways: The applications of T7 polymerase buffer are multifaceted. By understanding each application’s requirements, researchers can critically assess their methods and refine their techniques for enhanced experimental outcomes.
Troubleshooting Common Issues
Effective use of T7 polymerase buffer requires attention to specific problems that may arise during experiments. Troubleshooting common issues is essential because it helps to ensure successful nucleic acid synthesis. Recognizing symptoms and understanding their underlying causes enhances the reliability of research outcomes.
Inhibition of Polymerase Activity
Inhibition of polymerase activity is a frequent issue faced by researchers using T7 polymerase. Several factors can lead to this inhibition. First, specific ions can interfere with enzyme function. For instance, high concentrations of magnesium ions are necessary, but an excess can disrupt the enzyme’s active site. Other possible inhibitors include certain nucleic acids or proteins present in the reaction mixture, which can stably bind to the polymerase and hinder its function.
To address inhibition, it may be beneficial to simplify the buffer composition. Reducing the concentration of certain components might enhance enzyme activity. Another approach includes optimizing incubation conditions, such as temperature and time, as these can vary modularly to promote proper enzyme activity.
Additionally, performing control reactions can help identify whether the problem lies within the buffer or with the polymerase itself. Establishing a standard test with positive control can facilitate the identification of the inhibitory factors reliably.
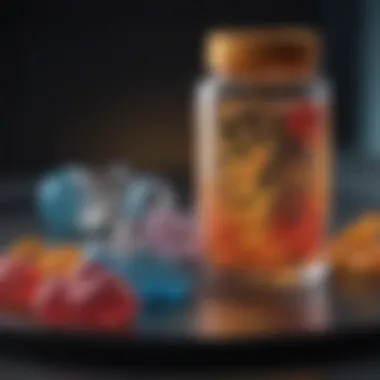
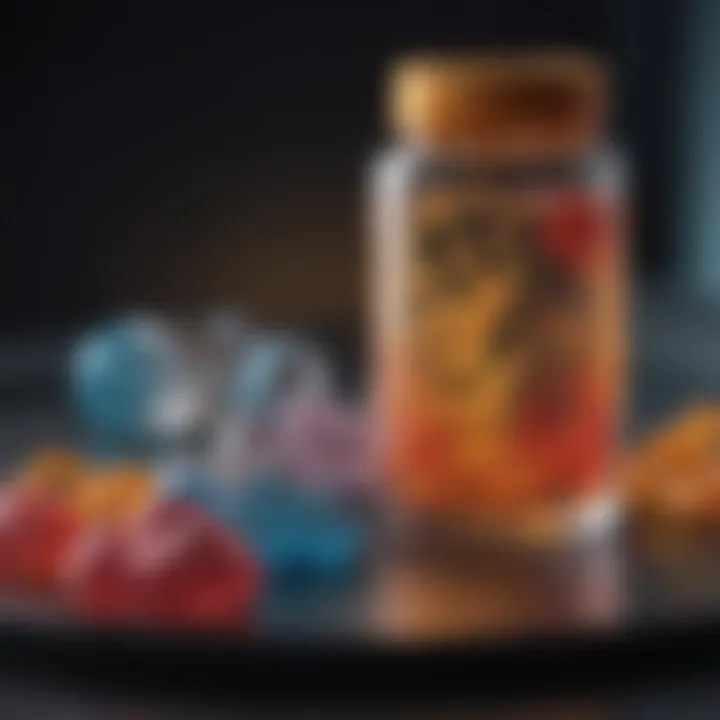
Poor Yield Problems
Poor yield in T7 polymerase reactions can indicate several underlying concerns. A common reason for low yield is an inadequate concentration of dNTPs, which are crucial for DNA synthesis. It is essential to verify that the dNTPs are present in sufficient amounts and are not degraded or improperly stored.
Buffer components, such as Tris-HCl or KCl, also play a vital role in achieving optimal yields. Inappropriate pH levels or ionic strength can adversely affect the polymerase's efficiency. Always ensure the buffer is prepared accurately and stored correctly to avoid issues with concentration shifts.
Furthermore, inefficient template concentration can lead to suboptimal yields. It's advisable to optimize the amount of DNA template used, as too little or too much can impede the reaction. Generally speaking, following troubleshooting protocols can diminish these yield problems.
In every molecular biology experiment, identifying and rectifying issues can intensively improve results, underscoring the necessity of a comprehensive approach to troubleshooting.
In summary, addressing inhibition of polymerase activity and overcoming poor yield problems is crucial for optimizing T7 polymerase buffer applications. By acknowledging these common challenges, researchers can refine their experimental designs and enhance the efficiency of nucleic acid synthesis.
Future Directions and Innovations
As molecular biology continues to evolve, the significance of T7 polymerase buffer in various applications cannot be overstated. Future directions in this field are critical for driving innovation, enhancing efficiency, and improving the overall understanding of nucleic acid synthesis. New formulations and innovative methods will cater to advanced research needs and can facilitate results that are more accurate and reproducible.
Advancements in Buffer Formulations
Recent years have seen a concerted effort towards developing improved buffer formulations for T7 polymerase. These advancements focus on optimizing ionic strength, pH levels, and component interactions.
Some key trends include:
- Reduced reagent concentrations: Minimizing the levels of certain reagents can decrease costs and enhance environmental sustainability.
- Enhanced stability: By modifying components in the buffer, researchers are achieving longer shelf-life and better performance during storage.
- Tailored buffers: Custom buffers that cater to specific reactions or experimental conditions provide researchers with more precise control over polymerase activity. This can significantly impact yield and efficiency.
These advancements in buffer formulations directly affect the reproducibility of experimental results, demonstrating their critical role in research and applications.
Novel Applications in Synthetic Biology
Synthetic biology represents a forefront of modern biological research, and the role of T7 polymerase buffer is pivotal in this area. Its applications are extending into various novel realms, reshaping approaches in research and industry.
Some notable applications include:
- Gene Synthesis: Fine-tuning the buffer can allow for more efficient gene synthesis, making it easier to construct synthetic genes for research purposes.
- Synthetic Circuit Development: T7 polymerase is increasingly vital for constructing genetic circuits. These circuits can perform complex functions, demonstrating significant potential in bioengineering.
- Vaccines and Therapeutics: The development of mRNA-based vaccines relies heavily on T7 polymerase. Optimizing the buffer conditions can lead to higher yields of mRNA, improving the efficiency of vaccine production.
By exploring these novel applications, researchers can unlock new possibilities for biological innovation, emphasizing the importance of continued development in the buffer composition and application methodologies.
Ending
The conclusion of this article emphasizes the critical role that T7 polymerase buffer plays in molecular biology research and experimentation. Understanding the specific composition and applications of this buffer is essential for scientists looking to optimize their enzymatic reactions. Properly selected buffer components can drastically affect the efficiency, accuracy, and yield of nucleic acid synthesis, making it a focal point in protocol design.
Summary of Key Points
In summary, there are several key points discussed throughout the article:
- Unique Composition: T7 polymerase buffer has specific components like Tris-HCl, KCl, MgCl2, and dNTPs that serve distinct functions.
- Optimal Conditions: The importance of temperature, pH balance, and ionic strength can not be overstated in maximizing polymerase activity.
- Real-world Applications: Understanding its applications in in vitro transcription, DNA amplification, and gene cloning demonstrates the versatility of this buffer in various experimental settings.
- Troubleshooting: Being aware of common issues such as polymerase inhibition and low yield empowers researchers to refine their methods.
Importance of Continued Research
Continued research into T7 polymerase buffer and its components is essential for several reasons:
- Innovations in Buffer Design: Ongoing studies can lead to the development of next-generation buffer formulations that enhance enzymatic performance.
- Expanding Applications: Innovation may unlock new uses for T7 polymerase in synthetic biology, creating pathways for new scientific discoveries.
- Understanding Mechanisms: Deeper insight into the biochemical interactions within buffers can inform how to manipulate conditions for specific experiments effectively.
- Training Future Scientists: Continuous research contributes to the education of new generations of scientists, ensuring they can navigate complex molecular biology techniques proficiently.
The pursuit of knowledge in this area fosters a deeper understanding of molecular processes and cultivates advancements that benefit the broader scientific community.
The quality of experimental results heavily depends on the understanding and optimization of buffers used in complex reactions.