Understanding the T7 Terminator Sequence in Molecular Biology
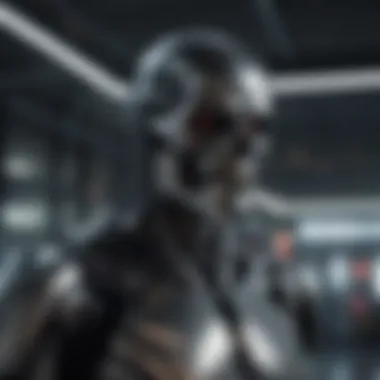
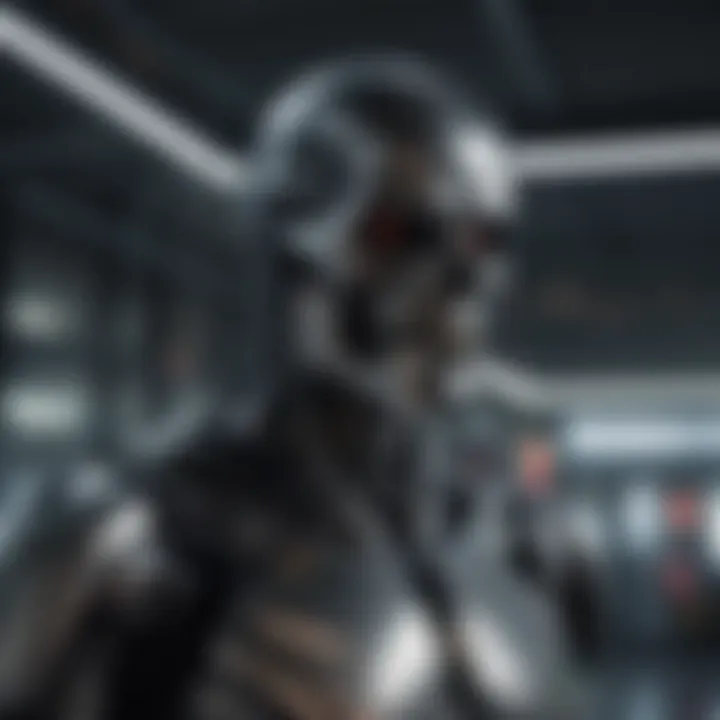
Intro
The T7 terminator sequence is more than just a mere molecular fragment; it acts like a finely tuned stop signal for transcription, essential in the complex world of molecular biology. This article sets out to unravel its intricate structure, elucidate its function, and showcase its diverse applications that stretch across various scientific fields. Whether you're a seasoned researcher or an eager student, understanding this sequence offers insights into the larger workings of genetics and biotechnology.
Research Overview
The T7 terminator sequence has proven its worth in numerous studies, making clear its role in the landscape of gene expression regulation. Notably, it serves as a critical component in synthetic biology frameworks, enabling engineered organisms to exhibit desired traits or behaviors more effectively.
Key Findings
- Transcription Termination: The T7 terminator instructs RNA polymerase to cease transcription, ensuring that genes are expressed at the right time and intensity.
- Efficient RNA Production: Using the T7 terminator can significantly enhance the yield of RNA when utilized in transcription systems, making it invaluable for research and therapeutic applications.
- Versatility in Applications: This sequence is employed in various fields, including synthetic biology, where it's integral to constructing artificial gene circuits.
Study Methodology
Research into the T7 terminator sequence typically involves a blend of experimental and computational approaches. Techniques like:
- Molecular Cloning: Involves inserting the T7 terminator into plasmids to modify transcription outputs.
- In Vitro Transcription Assays: Evaluates the efficiency of the terminator's transcription termination.
- Bioinformatics Analyses: Used to predict the interactions and efficiencies of terminators in synthetic gene designs.
This holistic approach allows researchers to develop nuanced understandings of the T7 terminator's function and utility.
Background and Context
Historical Background
The investigation into terminator sequences began as scientists sought to comprehend the nuances of transcription termination. Researchers soon focused on the T7 bacteriophage, which led to the identification of its effective terminator. As the understanding of genetic regulation evolved, so did the importance of such sequences in genetic engineering and biotechnology.
Current Trends in the Field
In recent years, there has been a surge in interest surrounding the T7 terminator sequence, particularly due to advancements in synthetic biology. Researchers are now leveraging it to optimize gene expression in synthetic organisms, enhancing applications in medicine, agriculture, and environmental science. The trend reflects a broader shift towards precision in genetic manipulation, where every nucleotide plays a crucial role.
Foreword to T7 Terminator Sequence
The T7 terminator sequence occupies a pivotal position in the field of molecular biology, acting as a critical player in the regulation of gene expression. Its importance lies not only in its fundamental biological functions but also in its vast applications spanning various scientific realms such as synthetic biology and biotechnology. Understanding the T7 terminator sequence enhances our grasp of genetic mechanisms, ultimately leading to advancements that might revolutionize therapeutic approaches and bioengineering practices.
Definition of T7 Terminator Sequence
The T7 terminator sequence is a specific segment of the DNA that signals the end of transcription in prokaryotic systems, particularly in those utilizing T7 bacteriophage RNA polymerase. This sequence essentially tells the transcription machinery when to stop synthesizing RNA, ensuring that mRNA is produced with the necessary structural integrity for subsequent translation.
This terminator is characterized by its distinctive secondary structure that enables its function. The sequence typically consists of a conserved stem-loop followed by a series of uridine residues, creating a thermodynamically stable configuration. This stability is paramount as it facilitates efficient termination, leading to precise control over gene expression.
Discovery and Historical Context
The discovery of the T7 terminator sequence can be traced back to the early studies of the T7 bacteriophage, a virus that infects Escherichia coli. Initially, research focused primarily on T7 RNA polymerase, recognized for its robustness in initiating transcription at T7 promoters. However, subsequent studies in the 1970s and 1980s brought attention to the necessity of termination sequences to control RNA synthesis effectively.
"Understanding the dynamics of transcription termination fostered new insights into genetic regulation, propelling research into synthetic constructs that utilize these sequences for precise expression control."
As researchers began to manipulate these sequences, their applications expanded beyond basic research. The T7 terminator became a cornerstone in genetic engineering, particularly in the production of recombinant proteins. Through these historical developments, T7 terminator sequences transitioned from purely bacteriophage studies to indispensable tools in molecular biology and biotechnology, solidifying their relevance in contemporary scientific exploration.
Molecular Structure of T7 Terminator
Understanding the molecular structure of the T7 terminator is crucial for grasping its role in genetic transcription processes. The structural elements not only provide insights into its functioning but also inform its applications in synthetic biology. Indeed, the unique composition and configuration of the T7 terminator sequence form the bedrock of its ability to regulate gene expression and improve the stability of mRNA transcripts.
Nucleotide Composition
The nucleotide composition of the T7 terminator sequence is specifically tailored to fulfill its biological roles. It comprises a variety of nucleotides—adenine, guanine, cytosine, and thymine—present in particular ratios. This sequence typically includes a high proportion of G and C which contributes to stronger hydrogen bonding, enhancing structural stability.
The BC (base composition) directly affects the binding affinity of RNA polymerase during transcription termination. A sequence rich in GC pairs tends to form a more stable double helix, which is vital for effective termination of the transcription process. The specific nucleotide arrangements are not random; they are optimized through evolutionary processes to ensure efficient and reliable function.
Secondary Structure Formation
The secondary structure of the T7 terminator is where the real magic happens. It’s characterized by the formation of unique shapes, crucially a hairpin loop
Hairpin Loop Formation
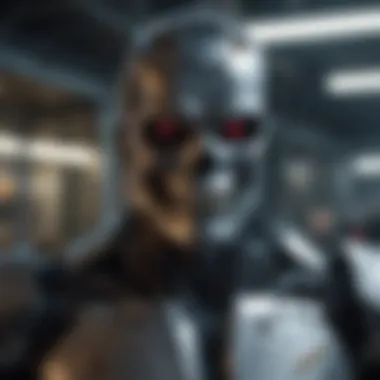
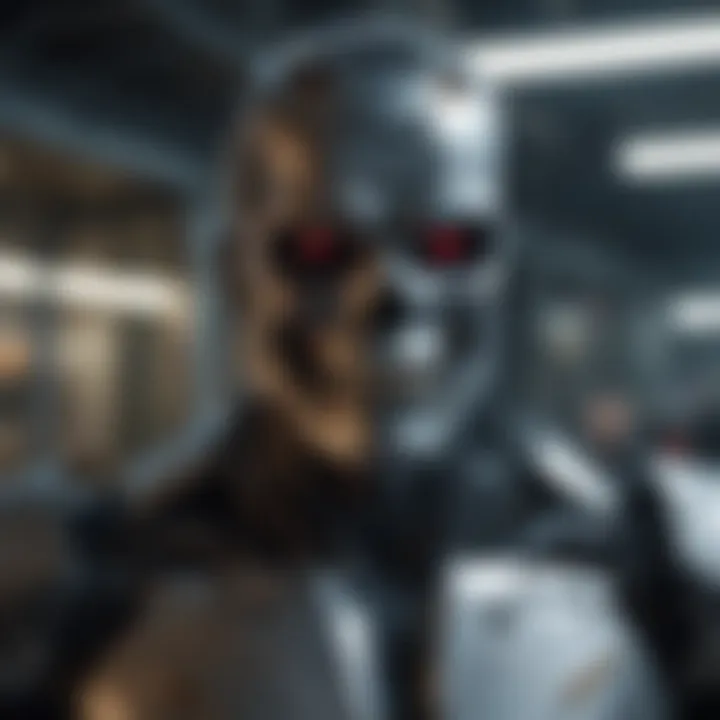
The hairpin loop is a standout feature of the T7 terminator, known for its specific contribution to transcription termination. When the RNA transcript folds back on itself, it creates this loop, effectively signaling the RNA polymerase that it should stop synthesizing RNA. The hairpin structure adds a layer of stability and functionality because, when this loop forms, the enzyme has a harder time continuing, allowing for precise termination.
This characteristic makes hairpin loop formations immensely advantageous. Not only do they act as strong signals for termination, but they also contribute to the overall stability of the RNA molecule. However, it’s crucial to note that if the hairpin does not form properly due to mutations or structural defects, it can lead to incomplete transcription, rendering the gene expression ineffective. The efficiency and precision offered by the hairpin loop are, therefore, invaluable to the goal of maintaining genetic integrity.
Stability Factors
Stability factors are also vital in the context of the T7 terminator's performance. Various parameters, like temperature and ionic strength, can influence the structure and consequent function of the terminator sequence. Specific stabilizing elements, such as base stacking interactions, can significantly affect the longevity and effectiveness of the T7 terminator structure. This stability is essential not just for the termination of transcription itself but also for the subsequent stages where RNA is utilized within the cell.
The presence of specific modifications in the terminator sequence can enhance its stability. For instance, methylation of bases can prevent degradation of RNA, further solidifying the framework upon which gene regulation is built. A fully functional T7 terminator sequence that maintains structural integrity under varying conditions is better equipped to serve its purpose effectively.
In summary, the molecular structure and stability of the T7 terminator play pivotal roles in its function and applications. Its nucleotide composition and the ability to form a stable secondary structure are what empower it to terminate transcription efficiently and regulate gene expression delicately.
Mechanism of Action
The mechanism of action for the T7 terminator sequence is a cornerstone of understanding its functionality within gene expression and transcription termination. This sequence operates as a regulatory element, effectively dictating when transcription should cease. Understanding how it works offers insight into its critical role in molecular biology and biotechnology applications.
The T7 terminator, in essence, is designed to create a pause or stop signal during RNA synthesis. This ensures that the transcription process doesn’t run amok, producing unwanted or excessive RNA products. The significance lies in its precision, allowing for a tightly controlled transcriptional apparatus relevant in many scientific domains.
Transcription Termination Process
Transcription termination is an essential step in gene expression, and the T7 terminator is particularly effective in initiating this process. It acts like a traffic sign on a busy road – when RNA polymerase approaches the T7 terminator, it encounters structural features that signal it to halt transcription.
The sequence typically leads to the formation of a hairpin structure in the nascent RNA transcript, which forms due to complementary base pairing. This secondary structure serves two functions: first, it destabilizes the interaction between the RNA polymerase and the DNA template; second, it can recruit factors that dislodge the polymerase.
Role of RNA Polymerase
Dynamics of Interaction
The dynamics of interaction between RNA polymerase and the T7 terminator is a fascinating aspect. When RNA polymerase approaches this terminator region, an intricate ballet of molecular movements takes place. The unique folding of the RNA into a hairpin loop creates mechanical stress and alters the enzyme's conformation. This contrast between the steady-state binding mode and the unsteady termination mode illustrates why the T7 terminator is held in such high regard.
A notable feature of this interaction is its rapid response; once the hairpin forms, the likelihood of RNA polymerase dissociating increases significantly. This ability to trigger rapid termination makes it an advantageous element for synthetic biology applications, where timely regulation of gene expression is often necessary.
Termination Efficiency
Termination efficiency is another crucial aspect contributing to the overall effectiveness of the T7 terminator. High termination efficiency ensures that a clear and decisive stop signal is delivered to the transcription machinery, vital for maintaining the functionality of genetic constructs.
A key characteristic of the T7 terminator is its reliability in producing discrete RNA transcripts. This predictability is essential in applications such as gene editing and protein synthesis, where controlling the duration of RNA synthesis can greatly influence outcomes. However, variations might occur depending on the cellular environment, which could impact the overall efficiency. Thus, while it’s generally a staple in research and industrial applications, ongoing assessment of termination efficiency within different contexts remains essential.
"Understanding the T7 terminator's mechanism of action fosters innovations in how we manipulate genetic information, paving the way for advances in biotechnology."
Both the dynamics of interaction and the termination efficiency culminate in establishing the T7 terminator as a vital tool, with implications ranging from fundamental research to biotechnological applications. Each element works collectively to not only stop transcription but also to ensure that the process is executed with precision.
Functional Significance
Understanding the functional significance of the T7 terminator sequence is crucial for both theoretical inquiry and practical applications in molecular biology. This sequence plays a pivotal role in regulating gene expression and maintaining mRNA stability, two cornerstones that govern how genes are utilized in various biological contexts. Let's delve deeper into these elements, illuminating their benefits and key characteristics.
Regulation of Gene Expression
The T7 terminator sequence serves as a fundamental switch in gene expression regulation. When incorporated into synthetic biology constructs, the terminator aids in controlling when and how much mRNA is produced. This means that researchers can effectively manage gene expression by using the T7 terminator to halt the transcription process at the right moment.
In this context, the terminator often interacts dynamically with RNA polymerase, ensuring that the transcription machinery stops at the desired locus. Such regulation ensures that proteins are synthesized only when they are needed, contributing to cellular efficiency and precision.
This regulation has profound implications:
- It allows for the fine-tuning of metabolic pathways, which is especially useful in engineering microorganisms for bioproduction.
- By ensuring that gene expression occurs in a controlled manner, researchers can minimize unwanted side effects, such as toxicity, that can arise from overexpression of certain proteins.
In short, the T7 terminator is not just a passive sequence. Instead, it actively shapes the expression landscape of genes, echoing the underlying biological rhythms necessary for optimal cell function.
Impact on mRNA Stability
Beyond regulation, the T7 terminator sequence significantly contributes to the stability of mRNA. A stable mRNA is essential for effective translation into proteins, and the terminator plays a role in protecting mRNA from degradation by cellular enzymes. This stability can make a notable difference in protein yields in synthetic systems, such as those found in recombinant DNA technology.
Here are some considerations regarding its impact on mRNA stability:
- Protective Role: The downstream sequence of the terminator helps shield the mRNA from RNases, enzymes that can break down RNA molecules. This is particularly vital when mRNA needs to be harvested for analysis or to produce proteins in a laboratory setting.
- Decoupling Mechanisms: Stabilizing mRNA allows it to persist longer in the cellular environment, which may lead to increased protein production. This decoupling is of great importance when establishing robust expression systems.
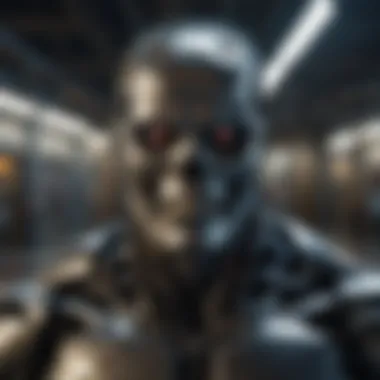
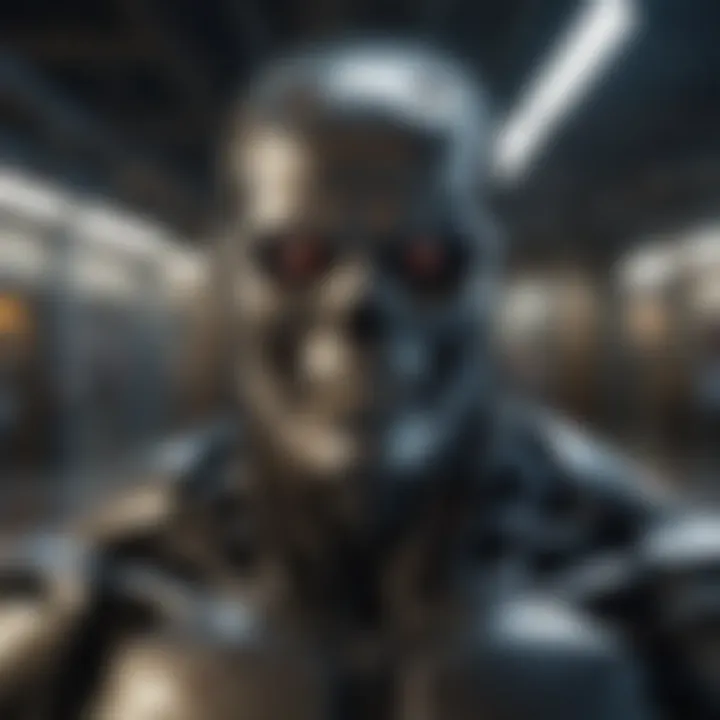
"By comprehending the functional significance of the T7 terminator, we gain insights that unlock the vast potential of biotechnology, offering solutions not only to scientific challenges but also to real-world applications."
With the functional nuances of the T7 terminator clarified, we can now explore its far-reaching applications in biotechnology, where its utility is genuinely tested and showcased.
Applications in Biotechnology
The T7 terminator sequence plays a pivotal role in various biotechnological applications, further solidifying its importance not just in molecular biology but also in practical science. In this section, we shall explore two primary domains of application: synthetic biology constructs and therapeutic applications. Each domain showcases how the T7 terminator enhances functionality and efficiency in biotechnological processes.
Synthetic Biology Constructs
Gene Expression Systems
Gene expression systems utilizing the T7 terminator have become increasingly popular among scientists. One of the key traits of these systems is their ability to yield high levels of protein production. This is crucial for research and commercial purposes. The T7 system provides a robust platform, allowing for precise control over gene expression, which is essential for ensuring consistency and reliability in experimental results.
What stands out in these systems is the unique interplay between the T7 promoter and the T7 terminator. This synergy creates a ‘push-pull’ effect that significantly enhances transcript yield, making it a favorable choice for those looking to optimize protein production without extensive resource investment. However, one downside can be the inherent complexity in constructing these systems, which may deter some novice researchers.
Bioreactor Optimization
Moving on to bioreactor optimization, the T7 terminator again makes its mark. A significant characteristic of bioreactor setups that employ this sequence is enhanced metabolic efficiency. Over the years, scientists have aimed to improve the growth rates of microbial strains for large-scale production, and the integration of the T7 terminator into these systems has yielded positive results.
Through various studies, it has been observed that bioreactors equipped with T7 sequences can offer a higher yield of desired products—be it proteins, enzymes, or even biofuels. Utilizing this approach, researchers can minimize contamination risks and maximize output. Nevertheless, the initial setup can be cost-prohibitive, making it a consideration for laboratories with limited funding.
Therapeutic Applications
The therapeutic applications of the T7 terminator sequence mark another realm of biotechnology where it holds significant promise. This is particularly evident in fields like vaccine development and gene therapy approaches, where the accuracy and robustness of genetic constructs can be the difference between success and failure.
Vaccine Development
When it comes to vaccine development, the T7 terminator sequence facilitates remarkable features. Notably, vaccines that utilize the T7 system benefit from enhanced expression of antigens, which is critical for stimulating a strong immune response. The ability to rapidly produce large quantities of antigen proteins also accelerates the development timeline, an urgent need in response to emerging pathogens.
This method has also been used in the creation of mRNA vaccines, where the T7 terminator plays a crucial role in ensuring mRNA integrity and stability. However, practical hurdles aside from technological challenges also persist, such as public perception and regulatory approval processes.
Gene Therapy Approaches
Finally, let’s discuss gene therapy approaches. The ability to deliver genes efficiently and safely is vital in this field, and the T7 terminator has shown to enhance the efficacy of various delivery systems. By ensuring precise termination during transcription, the T7 sequence supports higher expression levels of therapeutic genes, which is essential when treating genetic disorders.
A unique feature in employing the T7 terminator here is its compatibility with viral and non-viral vectors, offering researchers flexibility in choosing the most suitable method for gene delivery. Nevertheless, while gene therapies hold immense potential, they also come with challenges, including risks of immune responses and integration into the host genome.
Comparison with Other Terminators
In the realm of molecular biology, understanding different transcription terminators is essential. This knowledge not only sheds light on the T7 terminator sequence but also allows for insight into how various terminators interact with RNA polymerases and influence gene expression. Comparing the T7 terminator with other sequences can yield significant insights into their distinct mechanisms and potential suitability for specific applications.
T3 Terminator Sequence
The T3 terminator sequence serves as a compelling point of comparison with the T7 terminator. For starters, both sequences are derived from bacteriophages, but they exhibit notably different structures and functions. This divergence is crucial, as it impacts how they interact with RNA polymerases during transcription termination.
- Structural Differences:
- Transcription Dynamics:
- The T3 terminator tends to have a shorter conserved region compared to T7. This shorter length may lead to variations in termination efficiency when used in synthetic biology applications.
- The structural composition can influence the formation of secondary structures in the RNA, thus affecting stability.
- While the T7 terminator operates effectively under certain conditions, the T3 terminator can sometimes offer advantages in specific experimental setups, particularly where steric hindrance is a factor.
- Researchers have found that the T3 terminator can lead to higher yields of downstream products in some contexts, making it a favorable option for gene expression systems.
By examining the similarities and distinctions between T3 and T7 terminator sequences, researchers can make more informed decisions on which terminator to utilize based on their specific experimental requirements.
Rho-dependent Termination Mechanisms
Rho-dependent termination mechanisms represent a different strategy altogether. Unlike the T7 terminator sequence, which relies on intrinsic termination mechanisms, Rho-dependent termination depends on the Rho protein to cease transcription.
- Mechanism of Action:
- Applications and Limitations:
- Rho acts as a helicase; it binds to the RNA chain and moves along it until it catches up with RNA polymerase, which is paused at a termination site. Upon reaching the polymerase, Rho induces it to detach from the DNA, effectively terminating transcription.
- In contrast, the T7 terminator effectively terminates transcription through intrinsic mechanisms via the formation of a hairpin structure.
- Rho-dependent termination is not as widely used in genetic engineering applications as the T7 terminator. This difference arises from the fact that Rho is less predictable and consistent, potentially leading to varying results in experiments.
- However, understanding how Rho-dependent termination works allows researchers to utilize alternative strategies in gene expression, particularly in complex systems where intrinsic terminators may not function optimally.
In summary, comparing the T7 terminator with T3 and Rho-dependent terminators helps highlight their distinct mechanisms and usability in various applications. This nuanced understanding empowers scientists to make educated choices, pushing the boundaries of genetic research and biotechnological innovation.
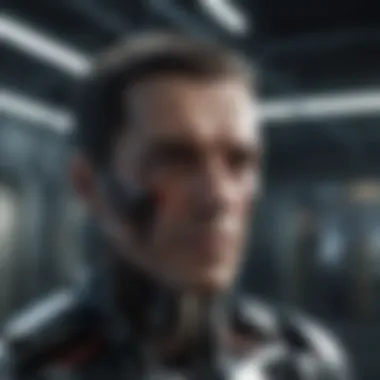
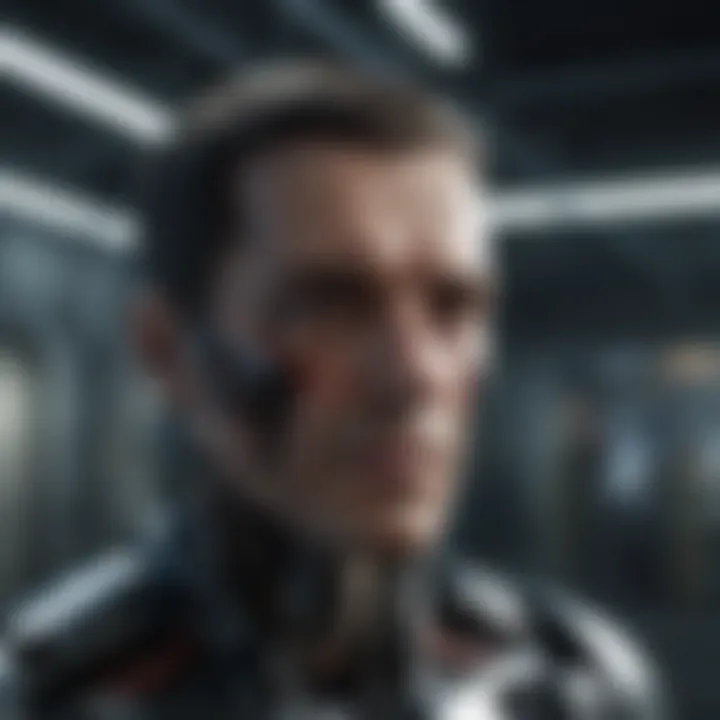
"Comparison of terminator sequences not only enhances understanding but also refines our approach to genetic engineering."
By fostering a thorough comprehension of various terminator mechanisms, the research community can focus on optimizing gene expression strategies across a diverse range of applications.
Challenges in Research and Applications
When delving into the intricacies of the T7 terminator sequence, the challenges that arise in both research and practical applications must be underscored. These hurdles can profoundly impact the ability of scientists and researchers to harness the full potential of this vital genetic sequence. Understanding these challenges is crucial not only for theoretical exploration but also for practical implementation in real-world settings.
Variability in Sequence Performance
The performance of the T7 terminator sequence can oscillate widely across different contexts, which is one of the primary concerns in molecular biology. Its effectiveness is often modulated by the surrounding genetic landscape. For instance, variations in upstream promoter strength, the presence of specific transcription factors, or even the cellular environment can alter how efficiently the terminator does its job. This unpredictability is akin to baking a cake; just because the recipe works perfectly for one baker doesn't mean it will yield the same results for another.
"Such variability necessitates rigorous testing and validation before adopting T7 terminators in downstream applications."
This inconsistency can lead to substantial challenges in synthetic biology constructs, where the reliability of gene expression needs to be spot on to ensure stable outputs. Inefficient transcription termination could result in read-through transcription, essentially causing unexpected protein products or, in worse scenarios, detrimental effects on cellular processes. Researchers must emphasize a robust experimental framework to assess the performance of T7 terminators across various systems.
Technical Limitations in Gene Editing
Another critical challenge arises from the technical limitations that confront gene editing techniques, specifically when employing T7 terminators. Traditional methods like CRISPR-Cas9, while groundbreaking, can struggle with precision when incorporating terminators into modified sequences. For instance, achieving the exact placement of the T7 terminator can be tricky, as any misalignment could jeopardize the intended gene expression outcomes.
Additionally, variations in the efficiency of delivery tools used to introduce these constructs can further complicate matters. Some delivery vectors may not disseminate the T7 terminator efficiently within the target cells. In scenarios where high fidelity is non-negotiable, such inconsistencies can become roadblocks.
Overall, addressing these limitations calls for an interdisciplinary approach, blending insights from molecular biology, genetics, and bioinformatics to develop more effective methodologies.
Future Directions in T7 Terminator Research
The field of molecular biology is like an ever-evolving tapestry, with each thread representing new discoveries and innovations. As we steer deeper into the nuances of the T7 terminator sequence, it’s crucial to visualize the future directions that research might take. Recognizing the importance of this topic paves the way for groundbreaking insights that could shape how we approach synthetic biology and gene regulation.
Significant effort is needed to unravel the complexities surrounding innovations in molecular design and gene regulation mechanisms. These elements are vital not just in comprehending the T7 terminator sequence itself, but also in pushing the boundaries of what can be achieved in biotechnology.
Innovations in Molecular Design
When considering innovations in molecular design, the T7 terminator sequence offers a fertile ground for exploration. Scientists are looking for ways to refine existing sequences to enhance effectiveness and specificity. By manipulating the nucleotide composition and secondary structures, researchers could create variants that respond to specific environmental cues or metabolic conditions.
For instance, engineers might seek to develop tailored terminators that are less prone to variability in performance across diverse cellular contexts. Techniques such as CRISPR can be modified to incorporate optimized T7 terminator sequences tailored for specific applications, enhancing precision in gene expression control.
- Increase in Specificity: New sequences could be engineered to improve the specificity of termination.
- Adaptive Design: By integrating feedback mechanisms, terminators can be designed to adapt to varying intracellular conditions.
- Multifunctional Constructs: Future designs may feature terminators that can engage in multiple roles, adjusting gene expression or contributing to metabolic pathways based on environmental signals.
The focus on optimizing these characteristics can lead to vast improvements in the performance of biotechnological applications, from pharmaceuticals to biofuels.
Gene Regulation Mechanisms
Understanding gene regulation mechanisms is akin to peeling layers from an onion. Each layer unveils more intricate relationships that govern how genes are turned on and off. The interplay between T7 terminator sequences and regulatory elements remains a rich area of inquiry.
New research avenues may explore how terminator sequences can influence downstream elements, not only halting transcription but possibly affecting adjacent gene activities as well. This variability poses intriguing questions:
- Transcriptional Read-Through: What role do T7 terminators play in Read-Through phenomena? Understanding this could unlock avenues for more robust expression systems.
- Co-Regulatory Effects: How do environmental factors or other genetic components modify the functionality of T7 terminators? There’s a chance we might discover synergistic relationships that could be harnessed therapeutically.
- Dynamic Regulation: Exploring the dynamic regulation of T7 terminators, influenced by the cell’s metabolic state or intracellular signals, would deepen our grasp on real-time gene expression.
Researching these mechanisms not only heightens our understanding of molecular biology but also enhances practical applications in gene therapy, vaccine development, and even crop engineering.
As we look toward the horizon of T7 terminator research, the potential is not just promising but extraordinary. It emphasizes the need for continued research and development in understanding these complexities and unlocking new biotechnological strategies. Engaging with this future is not merely an academic exercise; it’s a gateway into transforming how we perceive and utilize genetic information.
The End
Understanding the T7 terminator sequence is not just an academic exercise; it's a crucial step into the larger puzzles presented in molecular biology and genetics. This sequence plays a vital role in ensuring efficient transcription termination, which is essential for controlling gene expression. Without proper termination, genes might be expressed erroneously, leading to misguided pathways and dysfunctional cellular mechanisms.
Summary of Key Insights
In summary, the examination of the T7 terminator reveals several key insights:
- Structural Importance: The unique nucleotide composition and secondary structure allow for effective transcription termination.
- Regulatory Functions: Beyond mere termination, it plays a role in maintaining mRNA stability, affecting the overall regulation of gene expression.
- Versatile Applications: Its practical applications stretch beyond basic research into synthetic biology and therapeutic endeavors, proving it as a vital tool for scientists.
"The T7 terminator sequence, despite its simplicity, is a linchpin in both research and biotechnological applications, highlighting how minute changes can lead to significant outcomes in gene regulation."
Implications for Future Research
Looking forward, researchers must ponder several implications surrounding the T7 terminator sequence:
- Design Innovations: Further explorations into the molecular design can lead to better terminators, tailoring them to specific applications.
- Gene Regulation Mechanisms: Understanding how terminators interact with various transcription factors will deepen our knowledge of gene regulation.
- Personalized Therapies: With advances in medicine, there's potential for applying T7 terminators in custom gene therapy approaches, ensuring tailored solutions for genetic disorders.
The ongoing investigation into the T7 terminator and its multiple functionalities promises to lay the foundation for novel biotechnology advancements and refined therapeutic interventions. As this field evolves, we stand on the brink of transforming our approaches to genetic engineering, aiming for more efficient and safer methods.