Understanding Voltage Rectification: Principles and Techniques
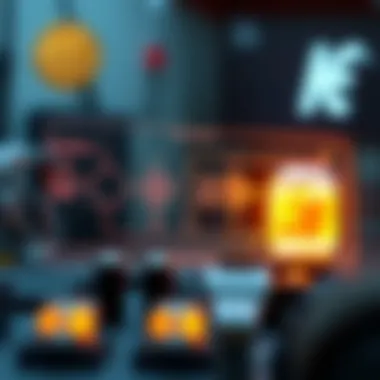
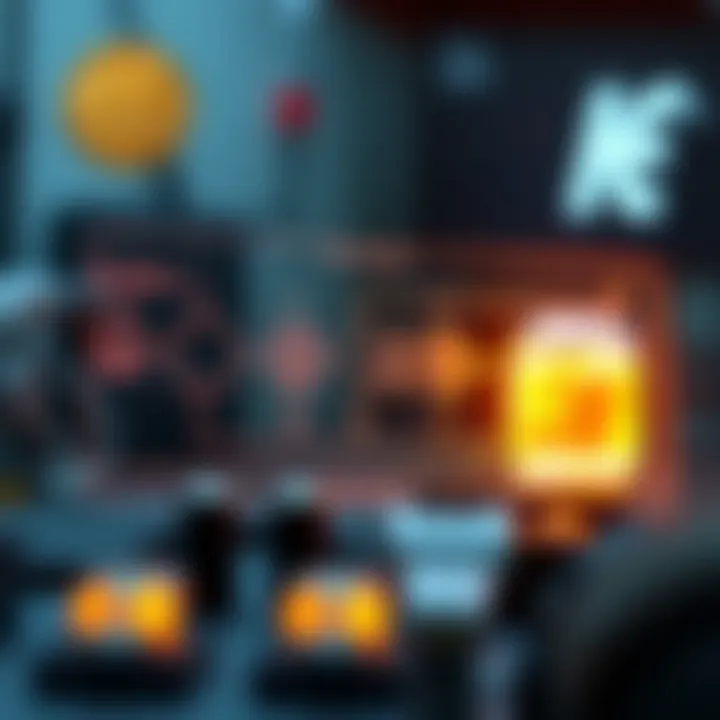
Intro
Voltage rectification plays a pivotal role in the foundation of modern electronics. It is the process of converting alternating current (AC) to direct current (DC), an operation that is integral for most electronic devices today. Without this conversion, many devices would simply not function; hence, understanding how rectification works is essential.
From smartphones to power supplies, the necessity for rectified voltage is ubiquitous. In an increasingly electronic society, where almost every gadget demands an efficient power source, the principles of voltage rectification command significant attention.
In this exploration, we will delve into the nuances of voltage rectification, examining its significance, the technologies driving advancements, and the practical implications for circuit design and efficiency. Here, we aim to paint a detailed picture of how voltage rectification is not just a technical process but a critical element that underpins the functionality of an array of electronic systems.
Let’s take a closer look at the key findings and the methodology that underlies our understanding of this crucial aspect of electrical engineering.
Preface to Voltage Rectification
Voltage rectification is a vital process in the world of electronics that ensures the efficient utilization of electrical energy. It serves as the bridge between the fickle nature of alternating current (AC) and the steadiness of direct current (DC), making it a cornerstone for various applications, from consumer gadgets to renewable energy systems. Understanding voltage rectification not only paves the way for effective circuit design but enhances overall electrical performance, reliability, and energy management. In a world where energy consumption is constantly under scrutiny, the knowledge surrounding rectification is crucial for engineers, researchers, and hobbyists alike.
Fundamental Definitions
To grasp voltage rectification fully, one must familiarize themselves with a few key terms:
- Rectification: This refers to the process of converting AC, which alternates direction and amplitude, into DC, which flows in a single direction.
- Diode: A semiconductor device that acts as a one-way valve for electrical current, allowing it to flow in one direction while blocking it in the opposite direction.
- Voltage: The electrical potential difference between two points in a circuit. In rectification, monitoring voltage levels is essential to determine the efficacy of the process.
These definitions form the foundation for understanding how rectification works and its significance in electronic systems. Essentially, without rectification, many modern electrical devices would be ineffective, if not entirely inoperable.
Historical Context
The roots of voltage rectification can be traced back to the late 19th century when electrical engineering was still in its infancy. Early pioneers like Thomas Edison and Nikola Tesla laid the groundwork for AC and DC systems. However, it was the invention of the vacuum tube by John Ambrose Fleming in 1904 that marked a pivotal moment. This groundbreaking invention operated as a rectifier and was instrumental in the development of radio technology, hence changing communication forever.
As technology advanced, the introduction of solid-state components in the mid-20th century led to the proliferation of more efficient rectification methods. The advent of diodes, particularly silicon-based ones, brought a small yet significant change in how electronic circuits handled voltage conversion. Each innovation, from the thermionic valves to modern semiconductor diodes, reflects the ongoing quest for improved efficiency and functionality in electrical engineering.
In today's landscape, voltage rectification is not only a critical component in traditional electronics but also plays a pivotal role in the emergence of renewable energy systems, where it ensures that solar panels and wind turbines provide usable power. The interplay of history and technology in this field continues to evolve, pushing the limits of what can be achieved in the realm of electrical systems.
Understanding Voltage in Circuits
When delving into the realm of electrical engineering, the concept of voltage plays a pivotal role in shaping how circuits function. This section is all about Understanding Voltage in Circuits, shedding light on the various properties, significance, and the practical implications of voltage in electronic systems.
Basic Electrical Concepts
Voltage is sometimes referred to as the potential difference, and it's vital to recognize that it serves as the driving force behind electric current. You can think of voltage as the pressure in a garden hose; the higher the pressure, the more water can flow through the hose. Similarly, a higher voltage can enable a greater flow of electrons through a conductor. This flow is what powers our devices, runs our household appliances, and fuels our advanced technologies.
In essence, voltage represents the energy per unit charge supplied to a circuit. Understanding the basic relationship between voltage, current, and resistance, typically expressed by Ohm's Law (V = I × R), is crucial. Here, V stands for voltage, I for current, and R for resistance. Knowing how these components interact helps engineers and hobbyists alike in designing efficient and effective circuits.
Types of Voltage
Voltage can be categorized primarily into two types: Direct Current (DC) and Alternating Current (AC). Both types have their own unique characteristics and are used in different applications, each making its mark in the world of electrical engineering.
Direct Current ()
Direct Current (DC) is characterized by the unidirectional flow of electric charge. This steady current is constant, which means that its voltage level remains unchanged over time. A staunch advantage of DC is its compatibility with batteries and many electronic devices. When you think about portable electronics—like cell phones or laptops—DC becomes a natural fit, since these devices rely on batteries to function.
An interesting feature of DC is its simplicity in circuit design. Devices that operate on DC typically require less complex circuitry compared to their AC counterparts. However, it does come with a notable trade-off: transmitting DC over long distances can lead to significant energy losses due to resistive heating. This makes it inefficient for large-scale electricity distribution.
Alternating Current (AC)
On the flip side, Alternating Current (AC) alternates its direction periodically, allowing it to flow in reverse. This is the type of current that powers our homes—the familiar electrical outlets provide AC to appliances and devices. The key characteristic of AC is its ability to transfer energy over great distances with less power loss. This is largely due to its higher voltages used in transmission lines, which minimize the heat generated.
One unique feature of AC is its frequency, typically measured in Hertz (Hz). In the United States, standard AC operates at 60 Hz, while in many parts of Europe, it's 50 Hz. This difference in frequency can be crucial when discussing compatibility with various devices.
Yet, AC is not without its disadvantages. Converting AC to DC can add layers of complexity to circuit design, especially in high-frequency applications where signal integrity is critical.
"Understanding the types of voltage—DC and AC—is fundamental when designing efficient electronic systems that cater to specific applications. Each type carries its own strengths and weaknesses that must be weighed carefully."
Rectification Techniques
Rectification techniques play a pivotal role in the realm of electrical engineering, mainly due to their function in converting alternating current (AC) to direct current (DC). This conversion is essential for a variety of applications ranging from household electronics to sophisticated industrial systems. When exploring rectification, it’s important to focus on the distinct methods available, each offering its own set of benefits and considerations.
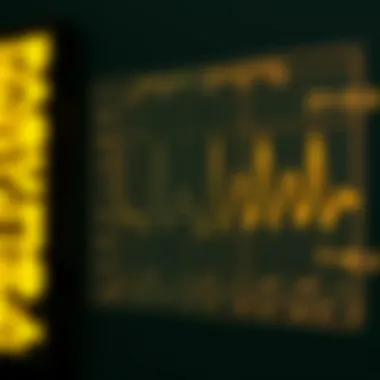
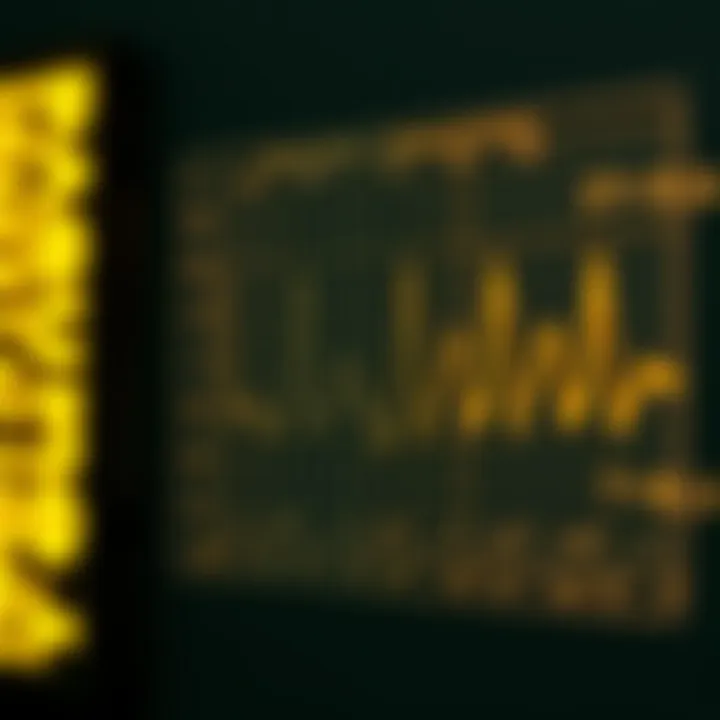
Understanding these techniques not only enhances the efficiency of circuit designs but also facilitates the reliable operation of devices that demand stable voltage levels.
Half-Wave Rectification
Operation Principles
Half-wave rectification is characterized by its simplicity. The operation is rooted in the use of a single diode that allows only one half of the AC signal to pass through, effectively blocking the negative half. This fundamental characteristic enables it to convert AC into a pulsating DC output.
The key feature of half-wave rectification is its low component count. With just one diode, it’s more accessible for beginners and cost-effective for simple applications. But to put it plainly, this convenience comes at a cost. The output is not smooth; it’s riddled with ripples which can cause inefficiencies in power delivery. Thus, while it serves its purpose well in low-power applications, its limitation in output quality makes it less favorable in more demanding scenarios.
Applications and Limitations
In terms of application, half-wave rectification finds its niche in some battery chargers and signal demodulation tasks. Its appeal largely lies in the fact that it can accomplish basic rectification tasks without the need for complex circuitry. This makes it particularly beneficial in situations where space and cost are primary concerns.
However, it’s crucial to discuss limitations, with the most notable being the high ripple factor—a measure of the fluctuation in DC output. This ripple can affect the performance of connected devices. Additionally, because only half of the input cycle is utilized, it is not the most efficient choice for power-intensive applications.
Full-Wave Rectification
Full-wave rectification not only complements half-wave methods but also addresses its shortcomings. This technique employs either bridge rectifiers or center-tapped rectifiers to utilize both halves of the AC waveform. The fundamental result is a smoother, more consistent DC output, which is crucial for modern electrical applications.
Bridge Rectifiers
Bridge rectifiers embody an innovative approach that employs four diodes arranged in a bridge configuration. This method cleverly allows the current from both halves of the AC signal to convert into DC. The main characteristic here is circuit symmetry, yielding a higher average output voltage than half-wave rectifiers.
This symmetrical capability makes bridge rectifiers a favored choice across various electronic projects—from power supply units in computers to battery chargers. An added advantage is their versatility: they can operate with both center-tapped and non-center-tapped transformers, providing an adaptable solution. On the flip side, the diode drop can introduce performance losses, leading to a slight decrease in efficiency compared to other methods.
Center-Tapped Rectifiers
Center-tapped rectifiers utilize a transformer with a center tap, employing two diodes to rectify both halves of the AC cycle. The distinguishing feature of this rectification style is its ability to produce a relatively high output voltage, leveraging both halves of the input signal while minimizing ripple.
Though center-tapped rectifiers are effective, their requirement for a center-tapped transformer adds complexity and cost. However, they can be exceptionally advantageous in applications where voltage regulation is critical. Acknowledging a drawback, they tend to be less efficient due to the need for two diodes and the resulting voltage drop.
In summary, both full-wave techniques offer a more robust solution than half-wave rectification, making them the preferred choice in power-sensitive environments.
Components Involved in Rectification
Understanding the components involved in voltage rectification is crucial for both aspiring engineers and seasoned professionals. The selection and function of these components can significantly affect the efficiency and reliability of electronic circuits. This section will dissect the primary components, focusing on diodes and capacitors, as well as their roles in rectification processes.
Diodes and Their Functionality
Diodes are the unsung heroes in the realm of voltage rectification. These semiconductor devices allow current to flow in one direction while blocking it in the opposite direction. This property is what makes diodes indispensable for converting alternating current (AC) into direct current (DC). Without diodes, the very nature of AC would lead to chaotic and unstable voltage levels.
The functionality of a diode hinges on its construction. Typically made from materials like silicon or germanium, most diodes exhibit a forward voltage drop when conducting. This means that the voltage across the diode must exceed a certain threshold before it turns on, which can introduce minor losses into the system. However, their ability to handle high-frequency signals and their robustness are key advantages that help maintain circuit integrity.
Unlike other components, diodes are generally more compact and can be arranged in various configurations. For instance, in full-wave rectifiers using bridge configurations, multiple diodes operate together to enhance output efficiency. ”
Capacitance and Filtering
Capacitors also play a vital role in the rectification process by acting as filters that smooth output voltage. After rectification, the voltage signal is often a pulsed waveform rather than a smooth DC signal. This is where capacitors step in.
Voltage Smoothing
Voltage smoothing is a specific aspect of the filtering process that helps reduce ripples in the DC output following rectification. By temporarily storing energy, capacitors release it gradually, leading to a more stable voltage. This characteristic makes voltage smoothing a vital choice in numerous applications, particularly in power supplies for sensitive electronic devices that require a consistent power level.
One unique feature of voltage smoothing is its reliance on capacitance value; larger capacitor values yield better smoothing effects. However, they can also lead to slower charge and discharge times, which can affect the responsiveness of circuits. In addition to selecting appropriate capacitance levels, engineers must also account for the equivalent series resistance (ESR) present in capacitors, which can introduce further complications in high-frequency applications.
Impact on Circuit Performance
The impact of capacitance and filtering is far-reaching in circuit performance. Efficient filtering not only stabilizes output voltage but also ensures that the connected components operate smoothly without glitches. It enhances circuit reliability by reducing noise and voltage fluctuations which could potentially lead to malfunctioning devices.
A critical characteristic of good filtering is the ability to maintain performance under various load conditions. In essence, effective filtering allows for seamless transitions between the power demands of different devices connected within the same network.
This unique feature of filtering capability can be a double-edged sword. While high-value capacitors contribute to better noise suppression and voltage stability, they also add bulk and complexity to circuit design, making layouts more challenging. Hence, the integration of advanced capacitors with lower ESR values can strike a balance between performance and design efficiency.
Ultimately, the right combination of diodes and capacitors not only facilitates efficient rectification but also enhances overall circuit performance, providing a reliable foundation for countless electronic applications.
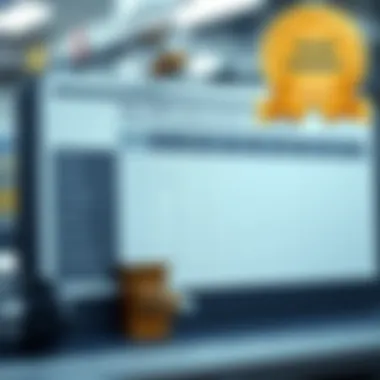
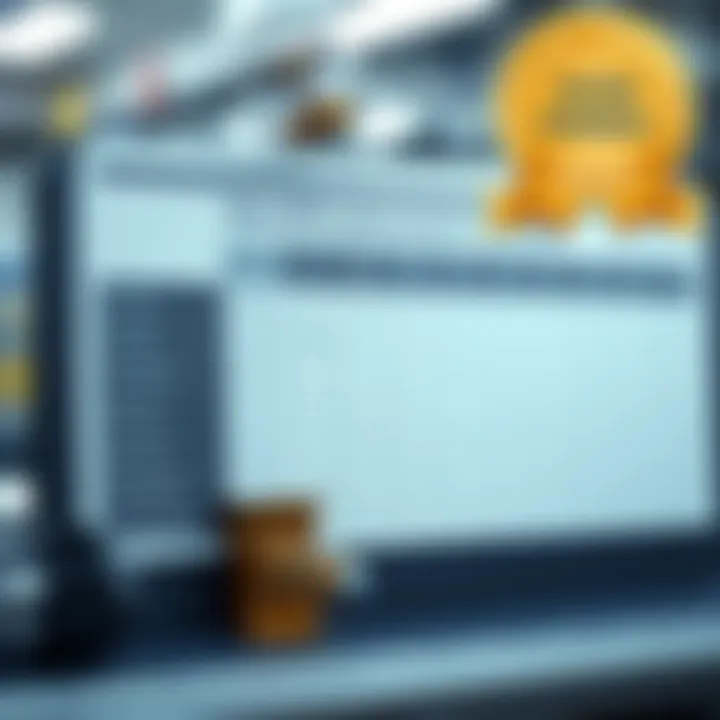
Voltage Regulation Techniques
In the pulsating world of electrical engineering, voltage regulation techniques play a pivotal role in ensuring devices run smoothly and efficiently. These methods govern how voltage levels are maintained or altered, keeping circuits stable and functionality intact. Without proper regulation, electronic devices can face devastating effects from voltage fluctuations—think of it like trying to drink from a fire hose instead of a garden tap.
Linear Voltage Regulation
Linear voltage regulators are favored for their simplicity and reliability. They operate by adjusting the resistance in response to changes in load and input voltage, helping maintain a steady output voltage. This mechanism is particularly integral for applications like battery chargers and low-noise audio systems.
Advantages and Disadvantages
When diving into the advantages, it becomes clear that linear voltage regulators are easier to implement, especially for those less schooled in the more complex realms of electronics. They typically provide very low output noise and are uncomplicated in their design, catering to users needing basic yet reliable performance. However, there are distinct disadvantages associated with them as well. These regulators often waste energy in the form of heat during operation, especially when input voltage is significantly higher than output voltage. Consequently, this can lead to thermal management issues, not to mention the inefficiency they introduce in power-sensitive applications. The subtle balance between efficiency and reliability is what makes them a mixed blessing.
Typical Use Cases
Looking at typical use cases, linear regulation shines brightest in low-power applications. For example, devices like simple sensors, microcontrollers, or lightweight RF transmitters often leverage linear regulators for their straightforward voltage maintenance. These use cases highlight a broader theme: simplicity can often be advantageous when refining circuit designs in various scopes of electronics. However, their usage tends to plateau when faced with high current demands, where the drawbacks of inefficiency become a glaring issue.
Switching Voltage Regulation
Switching voltage regulators, often viewed as the jazzy modern alternative, operate with a high degree of flexibility and efficiency! By rapidly switching their components on and off, they control the output voltage while minimizing wasted energy. This technique proves beneficial for applications like power supply units for computers and other devices requiring significant power.
Operational Methodologies
Exploring operational methodologies, switching regulators conceptually revolve around storing energy in inductors or capacitors during the 'on' phase and releasing it when switched 'off.' This method of energy transfer vastly improves efficiency compared to linear regulators, making them particularly beneficial in designs where energy conservation transcends mere desire. Moreover, the adaptability of switching regulators in delivering various voltage outputs from a single source is a significant draw.
Efficiency Considerations
Efficiency considerations become crucial, especially when dealing with larger currents. Switching regulators are famed for transforming power with minimal energy loss. In a world striving for greener technologies, this efficiency is tantamount to electric vehicles and renewable energy systems benefiting immensely from such innovations. However, it is essential to note that the design complexity can be a hindrance, and may not resonate well with those looking for straightforward implementations. The noise generated in the switching process can also become an unwanted guest in sensitive analog applications.
"Voltage regulation techniques are the unsung heroes of electronics, ensuring devices stay cool under pressure and maintain stability throughout their operation."
End
Ultimately, whether choosing linear or switching voltage regulation techniques, understanding their workings should not be overlooked. Not only are they foundational to circuit design, but they wield the control necessary to keep electronic devices functioning at optimal levels. Understanding how they contribute to efficiency and performance can pave the way for better electrical designs and innovations.
Applications of Voltage Rectification
The applications of voltage rectification are crucial in electrical engineering, presenting a myriad of benefits across various fields. By converting alternating current (AC) into direct current (DC), rectification underpins the functionality of countless devices we rely on daily. Understanding these applications is vital as it demonstrates not only the technical adaptability of rectification technologies but also their contribution to efficiency and performance in electronic systems.
Consumer Electronics
In the realm of consumer electronics, voltage rectification plays a fundamental role. From smartphones to laptops, any device powered by batteries or DC circuits relies heavily on this technology. For instance, chargers utilize rectifiers to convert the AC from wall sockets into usable DC for charging devices. This process is not just a matter of convenience; it enhances safety by providing stable and reliable voltage levels, protecting delicate circuits within devices from voltage spikes.
Moreover, with the proliferation of smart devices, energy efficiency becomes paramount. Using advanced rectification methods, manufacturers can decrease waste, prolonging battery life and reducing heat generation—two key considerations for today’s environmentally conscious consumer.
Electric Vehicles
Electric vehicles (EVs) represent another significant application of voltage rectification. These cars depend on efficient energy management systems where rectification is pivotal. During regenerative braking, for example, the vehicle converts kinetic energy back into stored electrical energy. Here, rectifiers allow the conversion of the generated AC back into DC, making it possible to recharge the vehicle's battery. This process not only boosts efficiency but also extends the range of electric vehicles by optimizing energy recovery.
Furthermore, as the global push for sustainability continues, electric vehicles are poised to benefit from advancements in rectification technologies. Innovations aim to improve charging time and overall system efficiency, making EVs a more viable option for the masses.
Renewable Energy Systems
The integration of renewable energy systems—such as solar panels and wind turbines—also hinges on effective voltage rectification. These systems typically generate AC but need to convert it to DC for usage and storage purposes. Rectifiers serve as the bridge in this process, ensuring that the energy harnessed from natural sources is efficiently utilized.
In solar power systems, for instance, the application of string inverters with integrated rectification enhances system performance. By managing voltage variations and maintaining a steady DC output, these technologies maximize energy production and minimize losses.
With the ongoing advancements in renewable technologies, the role of rectification is likely to evolve, promoting broader adoption of clean energy solutions and contributing to the fight against climate change.
"Voltage rectification is not merely a technical necessity but the backbone of modern efficiency in numerous applications."
In summary, the applications of voltage rectification extend to many critical areas, including consumer electronics, electric vehicles, and renewable energy systems. Each sector highlights the importance of stable and efficient voltage management, paving the way for further advancements and innovations that will shape our technological landscape.
Challenges and Limitations
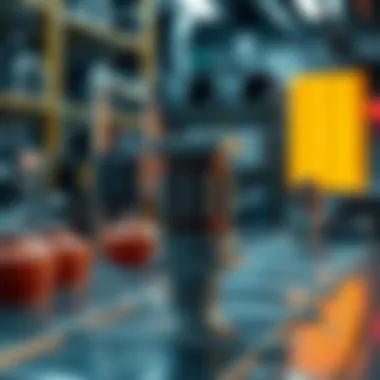
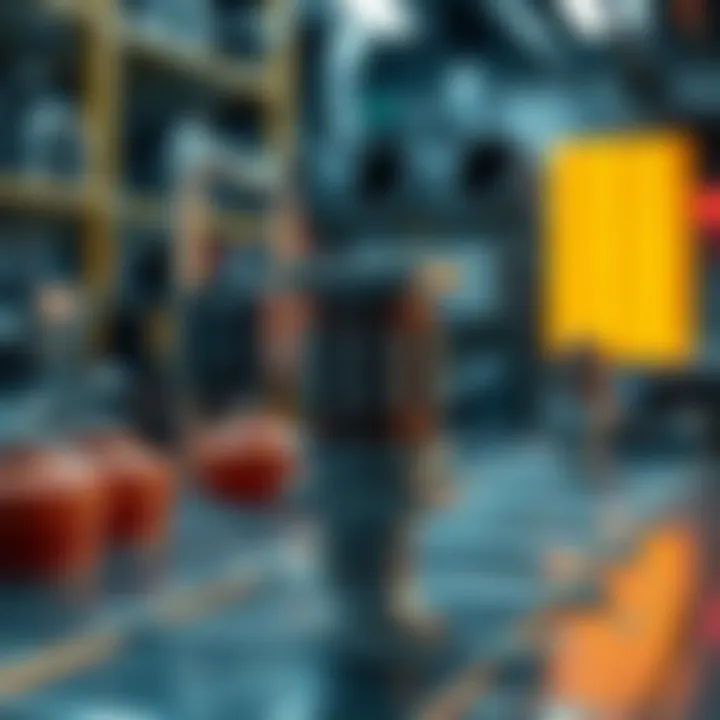
When delving into the realm of voltage rectification, it becomes imperatively crucial to acknowledge the challenges and limitations that accompany this essential technology. Recognizing these aspects not only helps in enhancing the efficiency of electrical systems but also promotes better design choices in various applications. This section aims to shine a light on efficiency losses and thermal management, both of which are pivotal considerations for researchers, educators, and industry professionals alike.
Efficiency Losses
Efficiency losses in voltage rectification are not just minor inconveniences; they can significantly affect the overall performance of electronic devices. Primarily, these losses occur due to energy dissipated as heat in the rectifying components, particularly diodes. The forward voltage drop in diodes introduces a notable resistance that can result in power loss.
"Understanding efficiency losses is vital for improving the designs of electrical circuits, ensuring that as little energy as possible goes to waste."
For example, in a standard silicon diode, the typical forward voltage drop may range from 0.6 to 0.7 volts. While this might not sound like much, in larger circuits or systems that require high currents, these small voltage drops accumulate, leading to significant energy losses.
The implications of these losses extend far beyond mere energy inefficiency. In critical applications such as renewable energy systems, where maximizing output is vital, efficiency losses can severely inhibit performance. Innovations like Schottky diodes and wide bandgap semiconductors aim to reduce these drops, showing much promise in improving overall system efficiency. However, their cost and availability often pose additional challenges.
Thermal Management
Thermal management presents another layer of complexity in voltage rectification, fundamentally intertwined with efficiency losses. As current flows through diodes during rectification, heat generation becomes unavoidable. Excessive heat can jeopardize component lifespan and lead to component failures, which are two unwelcomed scenarios in both consumer electronics and industrial systems.
Effective thermal management strategies should be implemented to mitigate these issues. Common approaches include:
- Heat sinks: These help dissipate excess heat away from critical components.
- Cooling systems: Active or passive cooling solutions can provide necessary temperature control.
- Optimized placement: Ensuring that components are strategically positioned to minimize heat concentration.
Moreover, choosing components with high thermal efficiency, such as power MOSFETs, may also be an answer to managing thermal loads better. However, it is essential to weigh the cost against performance, especially in budget-sensitive projects.
Future Trends in Voltage Rectification
The landscape of voltage rectification is undergoing significant transformations. As technology leaps forward, understanding these trends becomes essential for anyone involved in electronics, from students to seasoned professionals. The emphasis on efficiency and sustainability is reshaping how we design circuits and utilize rectification methods. Notably, future trends will also incorporate the advancement of materials, leading to more effective and adaptable systems, alongside the integration of smart technologies.
Advancements in Materials and Technologies
Materials science plays a vital role in enhancing rectification technologies. Traditional silicon diodes are gradually being supplemented by new semiconductor materials like gallium nitride (GaN) and silicon carbide (SiC). These offer better thermal conductivity and higher breakdown voltage, making them ideal in high-performance applications.
The potential of these materials lies in their ability to operate at higher frequencies with lower energy losses. For instance, using GaN in power converters can increase efficiency by 20-30% when compared to conventional silicon solutions. This is particularly crucial in applications demanding quick switching actions, such as in electric vehicles and renewable energy systems.
Additionally, the evolution of flexible and organic materials is opening doors to innovative applications. They offer promising pathways for the development of lightweight and flexible electronics that can adapt to various geometric constraints. This trend aligns well with the increasing need for portable and integrated devices. Such advancements pave the way for more compact, efficient designs that hold great potential for the future.
The shift towards new materials is not just about performance but also about adapting to a world focused on sustainability and innovation.
Integration with Smart Technologies
Integration with smart technologies is another critical trend shaping voltage rectification's future. As smart grids, Internet of Things (IoT) devices, and autonomous systems proliferate, the requirement for sophisticated voltage management becomes ever more pressing.
Smart rectifiers that can adapt their operating conditions based on real-time data offer numerous advantages. For example, implementing machine learning algorithms can optimize voltage regulation in response to fluctuating loads, ensuring peak performance while minimizing energy wastage. Such systems enable better responsiveness to varying demands, enhancing overall energy efficiency.
Moreover, the intersection of rectification with battery management systems is a focal point. As electric vehicles gain traction, managing voltage levels effectively to extend battery life and efficiency is vital. Smart charging strategies, which consider both the state of the vehicle’s battery and the grid’s conditions, will become the norm, ensuring optimal energy usage.
Lastly, enhancing connectivity between devices through smart technologies introduces unprecedented control over voltage in a decentralized manner. Imagine a home where all electronic devices communicate their energy needs, leading to an elaborate yet efficient energy distribution. Such scenario is indicative of how far the field is poised to evolve.
In summary, the future trends in voltage rectification emphasize the importance of material advancements and the integration with smart technologies. Both elements contribute significantly to developing more efficient, sustainable, and adaptive systems, signifying a promising frontier for electrical engineering.
The End
In wrapping up our exploration of voltage rectification, it's essential to emphasize why this topic matters not just in a theoretical sense, but also in practical applications. Voltage rectification is a cornerstone in electrical engineering, driving efficiency and functionality in myriad dispositivos—from the simplest battery charger to complex solar energy systems.
Recapping Key Points
To distill the rich information discussed in the sections above, here are some key takeaways:
- Understanding of Voltage Types: Recognizing the crucial difference between Direct Current (DC) and Alternating Current (AC) is foundational; each has unique implications for how voltage is managed in circuits.
- Techniques of Rectification: We navigated through half-wave and full-wave rectification methods, where the latter offers broader applications due to its higher efficiency.
- Components Essential for Rectification: The role of diodes, capacitors, and filters showcases the intricate interplay of electrical components in achieving desired voltage outcomes.
- Regulation Strategies: Shining a light on linear and switching regulation techniques reveals the trade-offs engineers must consider to optimize circuit design.
- Applications Across Industries: The impact of rectification extends into sectors like consumer electronics, electric vehicles, and renewable energy, underscoring its versatility.
- Emerging Challenges: Identifying thermal management issues and efficiency losses brings attention to potential areas for improvement.
Ultimately, the study of voltage rectification serves as a foundation for not just understanding current technological capabilities but also for anticipating future developments.
The Importance of Continued Research
The dynamics of voltage rectification are ever-evolving, representing a field ripe for further exploration. Continuing research in this domain holds several vital benefits:
- Innovation in Materials: Advancements in semiconductor technology can revolutionize diode performance, enhancing efficiency and reducing size.
- Smart Integration: As smart technologies gain traction, ensuring that voltage systems can communicate seamlessly becomes pivotal.
- Sustainability Initiatives: A focus on renewable energy solutions places voltage rectification at the heart of future energy strategies, aiding in the conversion of variable energy sources to stable outputs.
- Addressing Obsolescence: As electronics become more complex, old rectification methods may no longer suffice. Research ensures that existing theoretical frameworks adapt to modern needs.
Thus, the call for ongoing inquiry is not just necessary; it’s paramount for progress. By diving into the depths of voltage rectification, researchers and practitioners alike can unlock new potentials that will shape the future of electrical engineering and technology.
"Innovation distinguishes between a leader and a follower." — Steve Jobs
Continued investment in research and development fuels the progression of this critical field, ensuring that we keep pace with the rapid advancements in technology and sustainable practices.